Meniscus injuries are among the most common athletic injuries and result in functional impairment in the knee. Repair is crucial for pain relief and prevention of degenerative joint diseases like osteoarthritis. Current treatments, however, do not produce long-term improvements. Thus, recent research has been investigating new therapeutic options for regenerating injured meniscal tissue. This review comprehensively details the current methodologies being explored in the basic sciences to stimulate better meniscus injury repair. Furthermore, it describes how these preclinical strategies may improve current paradigms of how meniscal injuries are clinically treated through a unique and alternative perspective to traditional clinical methodology.
Key points
- •
Meniscus injury is one of the most common athletic injuries.
- •
Current reparative techniques fail to produce long-term improvements, and thus, alternative regenerative medicine applications are being investigated in the meniscus field.
- •
Acellular and cellular therapies as well as their delivery methods are being investigated for repair and regeneration of meniscus tissue.
- •
Various progenitor/stem cell types are being investigated as optimal cell sources to help stimulate native tissue regeneration of the meniscus.
Introduction
The meniscal injury is a major cause of functional impairment in the knee joint. This fibrocartilaginous tissue was once considered to be an unnecessary, vestigial appendage that could be sacrificed with minimal consideration. This total meniscectomy technique, although common in the past, has largely been abandoned because long-term results after major meniscectomy reported disappointing and adverse effects, such as the degradation of underlying articular cartilage and subsequent development of early osteoarthritis. As clinical medicine and basic science have evolved, the meniscus is now properly recognized as a necessary structure in the knee joint that is vital for biomechanical and anatomic purposes. Namely, the menisci are key for knee stability, distributing axial load, shock absorption between the articular cartilage of the tibia and femur, and nutrient distribution for protection of the underlying articular cartilage.
Biochemical Composition
The meniscus is composed of a dense extracellular matrix (ECM) that consists of 72% water, 22% collagen, and 0.8% glycosaminoglycans (GAGs). The remaining dry weight is made up of proteins, glycoproteins, and interspersed cells within the meniscus. These cells are referred to as fibrochondrocytes , because they have a marked resemblance to both fibroblasts and chondrocytes and synthesize the ECM and meniscal tissue. Type I collagen is expressed abundantly throughout the meniscus, whereas type II collagen is detected in the inner region. The interactions of these collagens, GAGs, and proteins likely account for the compressive load resistance, lubrication, and semielastic deformation properties of the meniscus.
Biomechanics and Gross Anatomy
The knee joint capsule houses the menisci, which are smooth, lubricated, crescent-shaped discs composed of fibrocartilaginous tissue with a medial and lateral component. These menisci sit on the natural contours of the tibial plateau between the femoral condyle and tibia of the knee. Joint motion and the biomechanical stressors associated with physical activity are important factors in determining the orientation of the collagen fibers that provide meniscal structure. There are 2 types of structural fibers that make up the meniscus: type I and type II collagen. These fibers are typically oriented based on the layers of the meniscus from surface to core: superficial (random orientation), lamellar (more organized radially at anterior and posterior horns), and deep (oriented circumferentially with some radial fibers) and allow the meniscus to expand under compressive forces to increase contact area of the joint.
A capillary network that originates in the synovium provides a direct blood supply to the meniscus, but only in certain “zones” of the meniscus. These zones are named based on the extent of vascularization of each respective region. The peripheral, outer third of the meniscus is known as the “red-red” zone, which has an excellent prognosis for repair because the blood supply is directly provided here. The intermediate “red-white” zone receives a limited blood supply and usually has a fair prognosis, as long as it is at the border of the vascular zone. The inner “white-white” zone of the meniscus, however, is completely avascular and presents a poor prognosis for recovery, regeneration, and healing. The lack of blood supply in this region confers difficulties for lesion/injury repair and regeneration of injured meniscus tissue. Current clinical reparative capacity seems to be restricted mainly to the peripheral, “red-red” vascular region of the meniscus, where there is a sufficient blood supply to promote healing. Biological tissue engineering and cell-based therapies constitute preclinical meniscus repair/regeneration strategies that offer much promise for the future.
Injury Prevalence and Call for Repair
With a combination of axial loading and rotational forces that generate a shear force, the meniscus is subject to both acute and degenerative injury, making it arguably the most commonly injured tissue in the knee. The epidemiologic data for the incidence and prevalence of meniscus injuries and clinical repair are limited, but seem to be rising every year. Logerstedt and colleagues, Hede and colleagues, and Jones and colleagues reported that the incidence rate of meniscus injury was 0.33 and 0.61 per 1000 person-years, with a prevalence of 12% to 14%. In the United States, of the estimated 850,000 to 1,000,000 cases per year in 2010, 10% to 20% of orthopedic surgeries involved surgical repair of the meniscus.
Clinicians and scientists alike agree that meniscal injury is considered an essential predictor of the subsequent development of degenerative joint disease and specifically strongly correlated with the development of early osteoarthritis. Therefore, considering the high incidence rate and increased risk of osteoarthritis development, it is critical to develop an ideal method for the prevention, repair, and treatment of injured meniscus tissue. Recent efforts have been directed toward regeneration of native meniscus tissue rather than meniscal resection. Although surgical techniques for mending damaged meniscus tissue have been extensively explored in the clinical setting, these repair attempts continue to fail for various reasons (ie, lack of longevity, tissue avascularity). Thus, it seems ideal to reframe this problem through an alternative perspective from the lens of basic science and regenerative medicine. This avenue, rather than meniscectomy or resection, has become an intriguing idea for addressing meniscal injuries in preclinical models. This review comprehensively details the current methodologies that are being explored in the basic sciences to stimulate better meniscus injury repair. Furthermore, it describes how these preclinical strategies may stand to improve current paradigms of how meniscal injuries are clinically treated through a unique and alternative perspective to traditional clinical methodology.
Current clinical paradigm
When clinicians come across an irreparable tear in the meniscus or with patients who have undergone a total or subtotal meniscectomy, meniscal allograft transplantation (MAT) may be considered a preferred modality for knee joint restoration. However, the indications for MAT remain controversial, because meniscal transplantation has been demonstrated to produce unsatisfactory results in the knee, including issues with graft size mismatching, donor incompatibility and sterilization, transplant remodeling and stability, and long-term chondroprotection.
With an increasing incidence rate of meniscal injury, the urgent need for an innovative and efficacious repair strategy has become evident. Because of the aforementioned limitations, MAT cannot serve as a “fix-all” model. Because of this, the development of acellular biological scaffolds emerged as an interesting alternative to MAT. The primary goal of using these scaffolds is to use a minimally immunogenic 3-dimensional (3D) tissue-replacement that stimulates migration, proliferation, and integration of endogenous/native cells into the scaffold for the purpose of restoring meniscus function with a secondary goal of chondroprotection with respect to joint-loading function.
Collagen Meniscus Implant
Currently in the United States, there is only 1 Food and Drug Administration–approved cell-free scaffold for meniscus replacement: the collagen meniscus implant (CMI). This scaffold was the first regenerative technique specifically invented and used for meniscus replacement in the clinical setting. The CMI is composed primarily of type I collagen fibers and GAGs isolated from bovine Achilles tendon that are sterilized via gamma-irradiation. This scaffold is perforated and porous to allow for ideal cell infiltration for better tissue integration purposes; clinical studies have indeed reported this native cell integration into the CMI. In theory, this scaffold appears to be a better replacement than MAT; because it is less destructive to the joint, its shape can be custom fit, and it is composed of biological tissue, thus virtually eliminating the risk of an immunologic response from donor mismatch. However, there has been debated controversy in terms of efficacy of the CMI. In 1999, Rodkey and colleagues reported that 8 patients who underwent arthroscopic replacement with the CMI at short-term follow-up demonstrated tissue regeneration, no degenerative progression, and chondroprotection of the joint surface. These results were confirmed by radiographs, and the histologic grading was confirmed new fibrocartilage formation. Furthermore, a medium-term follow-up study was done by Bulgheroni and colleagues in 2010, with 34 patients who underwent CMI implantation. These patients were evaluated 2 to 5 years later and showed good to excellent results with chondroprotection, no further degradation of the joint surface, and some newly synthesized tissue that appeared healthy. However, the CMI had rescinded in size and was presenting some clinical challenges. Long-term follow-up studies have demonstrated similar adverse results, because the implant significantly shrank in size after 5 to 6 years, possibly because of degradation, and led to decreased biomechanical function. The dangers of a potential size mismatch in the joint after implantation of the CMI would change the mechanical environment of the knee and decrease the chondroprotection of the underlying cartilage, resulting in further joint damage and likely further the progression of osteoarthritis.
Platelet-Rich Plasma/Fibrin
Within the field of orthopedics, the use of platelet-rich plasma (PRP) as a clinical therapeutic technique has seen a rapid increase in popularity. In the United States alone, it is estimated that 86,000 athletes are treated with PRP annually. PRP is an autologous blood product containing numerous growth factors and cytokines that is being implemented as a clinical intervention for musculoskeletal defects, including meniscus injuries. The increased concentration of platelets and growth factors is purported to aid in the native wound-healing process through the stimulation of meniscus cell proliferation and migration, angiogenesis, and matrix synthesis. Despite its growing popularity both in medicine and in the mainstream media, the efficacy and usage of this biological treatment remain controversial.
Pujol and colleagues attempted to augment repair and promote meniscal healing through the use of PRP treatment of horizontal meniscal tears. At a minimum of 24 months postoperatively, they reported a slight improvement in functional outcome and MRI-documented healing during midterm follow-up in young patients. Furthermore, Blanke and colleagues treated 10 patients with grade II meniscal lesions with percutaneous injections of PRP in 7-day intervals. Four of 10 patients showed a decrease of the meniscal lesion and relief of pain in a follow-up MRI and pain score after 6 months. In addition to slight pain relief and improved functional outcomes, certain groups have demonstrated the growth factor and immunologic response associated with PRP treatment. Wasterlain and colleagues found that serum growth factors were significantly elevated after PRP injection, which may contribute to a better repair response. It is thought that PRP may augment healing by increasing the levels of growth factors and cytokines localized to the injury site.
Although these groups have demonstrated positive results, there are also many others that report either (1) no significant improvements using PRP treatment or (2) adverse outcomes associated with PRP treatment. For example, 1 study conducted at the University of Virginia performed 35 isolated arthroscopic meniscal repairs with and without PRP augmentation and reported no clinical advantage of using PRP over non-PRP after a minimum follow-up of 2 years. Furthermore, Zellner and colleagues reported that implantation of a composite matrix loaded with PRP failed to improve meniscal healing in the avascular zone. The PRP-loaded matrix treatment outcome was characterized by poor tear filling without meniscal regeneration after a 3-month period. Available in vitro data reporting on the efficacy of PRP treatment of meniscal tears appear to be mixed as well, with groups finding contrasting results. It should be noted that recent studies have found some success by using various mesenchymal stem cell (MSC) sources supplemented with PRP for meniscus repair ; however, this field is still being investigated and needs more published data.
Although some studies have demonstrated the benefits of PRP as a therapeutic for meniscal regeneration and healing, the clinical efficacy and results seem to be mixed and unclear. More clinical studies with larger sample sizes and medium- to long-term follow-ups with measurable outcomes, such as histologic analysis and functional grading of meniscus repair, are needed to determine the true efficacy of PRP.
Acellular meniscus regeneration and repair techniques in basic/translational science
Decellularized Scaffold and Growth Factors
As an alternative method for a biomimetic cell-free scaffold, some have suggested using the process of decellularization of intact, native meniscus tissue in order to preserve the native structure and fiber-level organization. This decellularized scaffold technique is a significant advantage compared with other scaffolds because these retain the naturally occurring collagen networks present in the healthy meniscus. Furthermore, this type of scaffold would have a much lower risk of an adverse immunogenic reaction. However, 1 major challenge associated with using decellularized tissue is the low infiltration of cells into these scaffolds, because of their dense ECM structure as well as improper fitting and mismatch sizing issues in the joint.
In response to this, some groups have suggested using biomimetic, biosynthetic scaffolds coupled with chemotactic agents, such as growth factors, to stimulate native cell migration and infiltration to enhance integration into the scaffold. These growth factors that are used usually play a significant role in limb development. Local delivery or supplementation of growth factors may create a beneficial microenvironment to promote endogenic repair and integration for engineered tissue scaffolds. Growth factors act on target cells to stimulate cellular growth, proliferation, healing, and cellular differentiation. This effect is achieved usually through a receptor-mediated mechanism, whereby a growth factor will bind to its respective receptor and trigger a secondary system of signals or messengers to activate nuclear genes that control different cellular processes.
Certain growth factors have been demonstrated to play a key role in the metabolic activity of meniscal fibrochondrocytes (MFCs): regulating development, homeostasis, cell rejuvenation, and regeneration. With this idea in mind, there have been a myriad of different growth factor delivery methods to treat native fibrochondrocytes in both in vivo and in vitro experimental studies with the ultimate goal of optimizing meniscus tissue engineering and repair. Specifically, this review focuses on the supplementation of growth factors to MFCs (the native cell of the meniscus) or direct addition of growth factors to meniscal tissue. The most commonly used growth factors for treating meniscal tissue or MFCs seem to be basic fibroblast growth factor (bFGF), transforming growth factor beta-1 and -3 (TGFB1, TGFB3), and insulin-like growth factor-1 (IGF-1), with others being used less frequently ( Table 1 ).
Citation Source | Growth Factor Used | Cell Source | Results/Effects | In Vitro/In Vivo |
---|---|---|---|---|
Ionescu et al, 2012 | bFGF | None (tissue scaffold) | Short-term delivery-enhanced integration strength of native tissue with scaffold | In vitro explant–scaffold (bovine) |
Hiraide et al, 2005 | bFGF | MFCs | Enhanced cell proliferation | In vitro–monolayer |
Kasemkijwattana et al, 2000 | bFGF | MFCs | Enhanced cell proliferation | In vitro–monolayer |
Stewart et al, 2007 | bFGF | MFCs | Enhanced cell proliferation | In vitro–PGA scaffold culture |
Ionescu et al, 2012 | bFGF + TGFB3 | None (scaffold) | Improved scaffold/tissue integration and enhanced meniscus repair | In vitro–electrospun PCL scaffold |
Ionescu et al, 2012 | TGFB3 | None (tissue scaffold) | Sustained delivery-enhanced integration strength of native tissue with scaffold and increased proteoglycan content | In vitro explant–scaffold (bovine) |
Bochyńska et al, 2017 | TGFB3 | None (scaffold) | Regeneration of articular cartilage underlying meniscus by homing of endogenous cells | In vivo–PCL-HA scaffold (rabbit) |
Tarafder et al, 2018 | CTGF + TGFB3 | None (tissue scaffold) | Remodeling of fibrous matrix into fibrocartilaginous matrix by TGFB3 mechanism, induced recruitment of synovial mesenchymal cells/progenitor cells and meniscal tissue integration through CTGF application | In vitro–loaded fibrin glue scaffold (bovine) |
Tanaka et al, 1999 | TGFB1 | MFCs | Increased collagen and GAG synthesis | In vitro–monolayer |
Pangborn & Athanasiou, 2005 | TGFB1 | MFCs | Increased collagen and GAG synthesis | In vitro–PGA scaffold |
Imler et al, 2004 | TGFB1 | MFCs | Increased collagen and GAG synthesis | In vitro–meniscus explant culture |
Marsano et al, 2007 | TGFB1 | MFCs | Enhanced cell proliferation | In vitro–monolayer |
De Mulder et al, 2013 | TGFB1 | MFCs | Enhanced cell proliferation | In vitro–PU scaffold |
Forriol et al, 2014 | BMP-7 | None | Filled meniscal defect with cellular fibrous tissue | In vivo–intraarticular injection (sheep) |
Bhargava et al, 1999 | BMP-7 (OP-1) | MFCs | Enhanced cell migration/proliferation | In vitro |
Tumia & Johnstone, 2004 | IGF-1 | MFCs | Enhanced cell proliferation, synthesis of proteoglycans and ECM while inhibiting destruction of matrix | In vitro–monolayer culture |
Puetzer et al, 2013 | IGF-1 | MFCs | Increased levels of collagen and GAG synthesis | In vitro–scaffold culture |
Bhargava et al, 1999 | IGF-1 | MFCs | Enhanced cell migration/homing of cells | In vitro–explant culture |
Acosta et al, 2008 | IGF-1 + TGFB1 | None (tissue scaffold) | Enhanced repair of avascular (white-white) zone of meniscus | In vitro–explant culture |
Petersen et al, 2007 | VEGF | None (suture coating) | Failure to enhance repair or cell migration | In vivo–VEGF-coated sutures (sheep) |
Hidaka et al, 2002 | HGF | MFCs | Increased angiogenesis to promote healing response | In vivo–PGA scaffold (mice) |
Nishida et al, 2004 | CTGF | None (scaffold) | Enhanced articular cartilage regeneration | In vivo–hydrogel collagen scaffold (rat) |
Tumia & Johnstone, 2009 | PDGF-AB | MFCs | Increased cell proliferation rate and matrix synthesis/formation | In vitro–monolayer culture |
Marsano et al, 2007 | FGF-2 | MFCs/tissue | Enhanced cell proliferation | In vitro–monolayer culture |
Pangborn & Athanasiou, 2005 | FGF-2 | MFCs/tissue | Enhanced collagen synthesis | In vitro–scaffold culture |
Basic fibroblast growth factor
bFGF is a bioactive protein that acts as both a growth factor and a signaling protein that possesses broad mitogenic and cell survival activities, which play a primary role in angiogenesis, mitogenesis of fibroblasts, tyrosine activation, and inhibition of bone morphogenetic proteins (BMPs). When culturing MFCs in monolayer, Hiraide and colleagues and Kasemkijwattana and colleagues found that addition of bFGF resulted in enhanced cell proliferation. Further in vitro studies have demonstrated that bFGF addition resulted in enhanced native tissue integration into various scaffold models and improved meniscus repair.
Transforming growth factor-beta
The multifunctional cytokine superfamily, that is, TGFB, is involved in a receptor kinase mechanism that initiates a signaling cascade, which activates many downstream substrates and proteins. The role of TGFB isoforms TGFB1 and TGFB3 in the regulation of differentiation, chemotaxis, cell proliferation, and the immune response has been studied extensively in orthopedic research. In vitro supplementation of TGFB1 and TGFB3 to MFCs in monolayer, synthetic scaffolds, and explant tissue culture models have generally yielded positive results. These positive results include increased GAG and proteoglycan synthesis by native cells, enhanced native cell proliferation, regeneration of articular cartilage, and targeted homing of endogenous cells.
Insulin Growth Factor-1
IGF-1 is a member of the insulin-related peptide family that plays an important role in childhood growth with continued anabolic effects in adults. IGF-1 is a primary mediator of the effects of growth hormone, which stimulates systemic body growth and is of key interest in the orthopedic research field for musculoskeletal purposes. Addition of IGF-1 to MFCs in monolayer, scaffold culture, and explant culture in vitro resulted in enhanced cell proliferation, increased GAG and proteoglycan production, and better homing of cells.
Cell-based meniscal regeneration and augmentation techniques in basic/translational science
The cell-based therapeutic potential of human multipotent MSC has long been investigated in the field of meniscal tissue healing and regenerative medicine. This tissue engineering strategy is closer to translation than some might think, and there are even some clinical trials currently underway ( ClinicalTrials.gov Identifier: NCT02033525 ). Currently, groups are investigating several promising cell types and isolation methods to identify ideal MSC sources for meniscus repair. This exploration has produced encouraging but mixed results that are likely due to varying experimental conditions used in each individual investigation. This wide variation has made it difficult to directly compare efficacious outcomes in the field of tissue engineering and regeneration. With an aim to alleviate these difficulties, in 2006, the International Society for Cellular Therapy (ISCT) published a minimal criterion to define human MSCs. First, the MSCs must adhere to tissue culture plastic when maintained in standard culture conditions. Second, the phenotypic profile and epitope expression of MSCs must be at the very minimum: CD105 + , CD73 + , CD90 + while being CD45 − , CD34 − , CD14 − , CD11b − , CD79a − , or CD19 − . Third, MSCs must demonstrate trilineage potential, meaning that they can be differentiated into osteoblasts, adipocytes, and chondroblasts in vitro. In addition, it is thought that these stem cells must be self-renewing and have a high proliferation capacity in the sense that they can perpetually divide/replicate while maintaining an undifferentiated state.
Therefore, this review focuses exclusively on what the authors, in accordance with the ISCT minimal criterion, consider the MSC sources most widely used and most promising in the basic and translational science research setting for cell-based meniscus regeneration: bone marrow-derived mesenchymal stem cells (BM-MSCs), adipose-derived stem cells (ADSCs), synovium-derived stem cells (SDSCs), native MFCs, and progenitor cells, articular cartilage-derived progenitor cells (CPCs).
In the following paragraphs, the authors report on preclinical, in vivo studies with data on meniscal tear augmentation using various cell-based therapies. These data are the focus of Table 2 , which provides the cell type and quantity used, the animal model that was used, the length of time of the study, the measurements of successful treatment outcomes (histology, MRI, macroscopic, formation of neomeniscal tissue, presence of fibrochondrocytes, and so forth), and finally, the limitations for each cell type.
Cell Source | Number of Cells Used | Animal Model | Meniscus Injury Model | Experimental Treatment | Control | Outcome/Results | Reference |
---|---|---|---|---|---|---|---|
SPIO-labeled ADSCs | 2 × 10 6 | Rabbit | ½ anterior meniscectomy of medial meniscus | SPIO-labeled ADSCs | Saline OR unlabeled ADSCs |
| Qi et al, 2015 |
Allogeneic rabbit ADSCs | 0.1 × 10 6 | Rabbit | Longitudinal lesion in avascular zone | Suture + ADSCs suspended in Matrigel | Suture + Matrigel only |
| Ruiz-Ibán et al, 2011 |
Autologous sheep ADSCs | 20 × 10 6 | Sheep | Medial meniscectomy (and ACL resection) | Autologous chondrogenically induced ADSCs | Culture medium |
| Ude et al, 2014 |
Autologous Human ADSCs | 16 × 10 6 | Human | Grade II meniscal tear | Autologous hADSCs + PRP and hyaluronic acid injections | PRP + Hyaluronic acid injections |
| Pak et al, 2014 |
Autologous equine ADSCs | — | Equine | Medial meniscus defect | Autologous ADSCs | Autologous BM-MSCs |
| González-Fernández et al, 2016 |
Human ADSCs | — | Bovine (explant in vitro) | Radial tear on cylindrical explant punch biopsy from inner avascular region of meniscus | Photo-crosslinked hydrogel loaded with ADSCs + TGFB3 | TGFB3 only |
| Sasaki et al, 2018 |
Allogeneic rabbit ADSCs | — | Rabbit | Complete meniscectomy of medial meniscus | PVA/Ch scaffold seeded with ADSCs | Scaffold seeded with articular chondrocytes OR cell-free scaffold |
| Moradi et al, 2017 |
Allogeneic rabbit ADSCs | 5 × 10 4 cells/spheroid (400–500 spheroids) | Rabbit | Partial meniscectomy of the medial meniscus | High-density ADSC spheroid construct (3D culture) | No cells |
| Toratani et al, 2017 |
Autologous SDSCs | 0.25 × 10 6 | Primate | Partial meniscectomy of medial meniscus + insertional ligament of medial meniscus transection | Autologous SDSC aggregate | Intraarticular SDSC injection |
| Kondo et al, 2017 |
Allogeneic SDSCs | 20 × 10 6 | Microminipig | Longitudinal tear lesion in medial menisci | Injection of SDSC suspension + suture | Suture only |
| Nakagawa et al, 2015 |
Allogeneic SDSCs | 50 × 10 6 | Pig | Partial meniscectomy of medial menisci | Intraarticular injection of SDSCs at 0.2 and 4 wk | Intraarticular injection of PBS |
| Hatsushika et al, 2014 |
Syngeneic and allogeneic SDSC | 5 × 10 6 | Rat | Partial meniscectomy of medial meniscus | Intraarticular injection of syngeneic SDCSs, minor immune mismatch model cell transplantation, major immune mismatch model cell transplantation (for histocompatibility) | Intraarticular injection of PBS |
| Okuno et al, 2014 |
Autologous SDSCs | 10 × 10 6 | Rabbit | Partial meniscectomy of medial meniscus | Intraarticular injection of autologous SDSCs in PBS | No cells |
| Hatsushika et al, 2013 |
SDSCs | 0.25 × 10 6 | Rat | Partial meniscectomy | SDSC aggregate | Intraarticular injection of 5 × 10 6 cell suspension in PBS AND 0.25 × 10 5 cell suspension in PBS |
| Katagiri et al, 2013 |
Allogeneic SDSCs | 0.2 × 10 6 cells cultured for 3 wk to make 3D construct | Pig | 4-mm cylindrical defect in medial meniscus | Cultured SDSC 3D cell/matrix tissue construct (scaffold-free) | No treatment |
| Moriguchi et al, 2013 |
Allogeneic SDSCs | 2 × 10 6 | Rabbit | 1.5-mm cylindrical defect in avascular zone of medial meniscus | Allogeneic SDSCs suspended in PBS | PBS only |
| Horie et al, 2012 |
Allogeneic SDSCs | 5 × 10 6 | Rat | Partial meniscectomy of medial meniscus | Intraarticular injection of Luc/LacZ + SDSCs AND BM-MSCs | PBS only |
| Horie et al, 2009 |
Allogeneic/exogenous SDSCs | — | Rat | Cylindrical defect created in meniscus | Intraarticular injection of GFP-positive SDSCs | PBS only |
| Mizuno et al, 2008 |
Allogeneic SDSCs | — | Rabbits | Full-thickness longitudinal incision on medial meniscus | Fibrin gel loaded with SDSCs + CTGF and TBGFB3 | Fibrin alone OR Fibrin + CTGF |
| Tarafder et al, 2018 |
Autologous BM-MSCs | 6 × 10 6 | Sheep | Unilateral medial meniscectomy | Autologous BM-MSCs from iliac crest in HA construct | BMC in HA construct |
| Desando et al, 2016 |
Autologous BM-MSCs | 15–20 × 10 6 | Horse | Meniscal tear | Intraarticular injection of autologous, expanded BM-MSCs into the joint | Surgery only, NO treatment |
| Ferris et al, 2014 |
Autologous BM-MSCs | 0.1 × 10 6 | Rabbit | 4-mm longitudinal tear in avascular zone of medial meniscus | Implantation of autologous BM-MSCs cultured and embedded in hyaluronan/collagen matrix | Suture only, cell-free matrix construct, or PRP |
| Zellner et al, 2013 |
Human BM-MSCs and rat BM-MSCs | 2 × 10 6 | Rats | Partial meniscectomy | Intraarticular injection of human BM-MSCs or rat BM-MSCs | PBS only |
| Horie et al, 2012 |
Human BM-MSCs | 2 × 10 6 | Rabbit | Complete radial tear of medial meniscus at the anterior tibial attachment site | Pull-out surgical repair + human BM-MSCs embedded in a matrix gel scaffold | Pull-out surgical repair (NO cells) |
| Hong et al, 2011 |
Autologous BM-MSCs | 1.5 × 10 6 | Rabbit | 2-mm meniscal tissue punch defect in avascular zone | Hyaluronan-collagen composite matrices loaded with autologous BM-MSCs | PRP loaded in matrices OR autologous bone marrow loaded in matrices OR cell-free matrices |
| Zellner et al, 2010 |
Autologous BM-MSCs | 1–2 × 10 6 | Pig | Radial tear in the avascular zone of the meniscus | Autologous BM-MSCs + sutures and fibrin glue | No treatment OR sutures and fibrin glue alone |
| Dutton et al, 2010 |
Autologous BM-MSCs | 30 × 10 6 cells/mL | Goat | Full-thickness meniscal defect in white-white area of meniscus | BM-MSCs transfected with hIGF-1 + calcium alginate gel | Nontransfected BM-MSCs OR calcium alginate gel alone OR no treatment |
| Zhang et al, 2009 |
Autologous BM-MSCs | 2.5 × 10 6 , then 14 d in culture | Rabbit | Partial meniscectomy of middle ⅓ of meniscus | Autologous BM-MSCs (cultured for 14 d in chondrogenic conditions) loaded into a hyaluronan/gelatin scaffold | Cell-free scaffold OR no treatment |
| Angele et al, 2008 |
BM-MSCs | 0.5 × 10 6 /mL | Rabbit | Partial meniscectomy of medial meniscus | Type I collagen sponge loaded with autologous BM-MSCs | Cell-free collagen sponge OR periosteal autograft OR no treatment |
| Walsh et al, 1999 |
BM-MSCs | ∼1 × 10 6 cells (from bone marrow aspirant) | Rabbit | 1.5-mm full-thickness defect in avascular zone of meniscus | BM-MSCs suspended in fibrin glue | Fibrin glue alone OR no treatment |
| Ishimura et al, 1997 |
Allogeneic BM-MSCs | 0.3 × 10 6 | Rabbit and rat | Explant culture | Allogeneic rabbit BM-MSCs or rabbit MDSC housed in Matrigel | — |
| Ding & Huang, 2015 |
Allogenic horse BM-MSCs | 0.2 × 10 6 | Nude mice | Equine meniscal sections | Allogeneic horse BM-MSCs + fibrin glue subcutaneously implanted into rat | PBS OR fibrin glue alone | BM-MSC group showed increased vascularization with increased total bonding of repair and native tissue | Ferris et al, 2012 |
Autologous BM-MSCs | 11–12 × 10 6 | Sheep | Meniscal tear of medial meniscus | Intraarticular injection of BM-MSC suspension | Intraarticular injection of suspension medium (NO cells) |
| Caminal et al, 2014 |
Autologous BM-MSCs | 10 × 10 6 | Sheep | Complete meniscectomy of the medial meniscus + ACL excision | Intraarticular injection of chondrogenically induced BM-MSCs OR intraarticular injection of basal-culture medium BM-MSCs | Intraarticular injection of basal medium (NO cells) |
| Al Faqeh et al, 2012 |
Allogeneic BM-MSCs | 1 × 10 6 vs 10 × 10 6 | Rats | Meniscal tear + ACL tear + articular cartilage defect | Intraarticular injection of allogeneic GFP-positive BM-MSCs | Sham operation OR saline |
| Agung et al, 2006 |
Autologous BM-MSCs | 10 × 10 6 | Goat | Osteoarthritis (OA) induction through complete meniscectomy of the medial meniscus | Intraarticular injection of autologous BM-MSCs expressing eGFP (retrovirus) suspended in sodium hyaluronan | Sodium hyaluronan alone (NO cells) |
| Murphy et al, 2003 |
Human BM-MSCs | 30 × 10 cells/mL | Rat | Radial cut of the medial meniscus (2 mm × 2 mm excision) | Human BM-MSCs encapsulated in decellularized ECM hydrogel | PBS |
| Yuan et al, 2017 |
Autologous BM-MSCs | — | Rabbit | Total meniscectomy | BM-MSC seeded PCL scaffold meniscus replacement | Cell-free scaffold, sham operation, and total meniscectomy alone |
| Zhang et al, 2017 |
Allogeneic BM-MSCs | ∼4.8 × 10 6 | Rat | Partial meniscectomy (1/2 resection) of the medial meniscus | Allogeneic BM-MSCs cultured in a cell “sheet” from monolayer culture | No treatment |
| Qi et al, 2016 |
Allogeneic BM-MSCs | — | Rat | Meniscal defect | Allogeneic rat-derived BM-MSCs were seeded into a scaffold and cultured for 4 wk and then implanted | Cell-free scaffold OR meniscectomy |
| Yamasaki et al, 2008 |
Allogeneic human BM-MSCs | — | Rat | Meniscal defect | Allogeneic human GFP-positive BM-MSCs cultured in monolayer and then embedded in fibrin glue and transplanted to injury defect | No treatment OR fibrin glue only (NO cells) |
| Izuta et al, 2005 |
Autologous BM-MSCs | — | Rabbit | Partial meniscectomy of white-red zone | Autologous BM-MSCs loaded in a polyurethane scaffold (Actifit) and sutured into the defect | Polyurethane scaffold alone (Actifit) (NO cells) |
| Koch et al, 2018 |
CPCs | 0.1 × 10 6 | Rat (ex vivo) | Radial tear in inner anterior horn | C-PC line 3 + SDF-1 pretreatment OR primary C-PCs + SDF-1 pretreatment | BM-MSCs OR no cell treatment |
| Jayasuriya et al, 2018 |
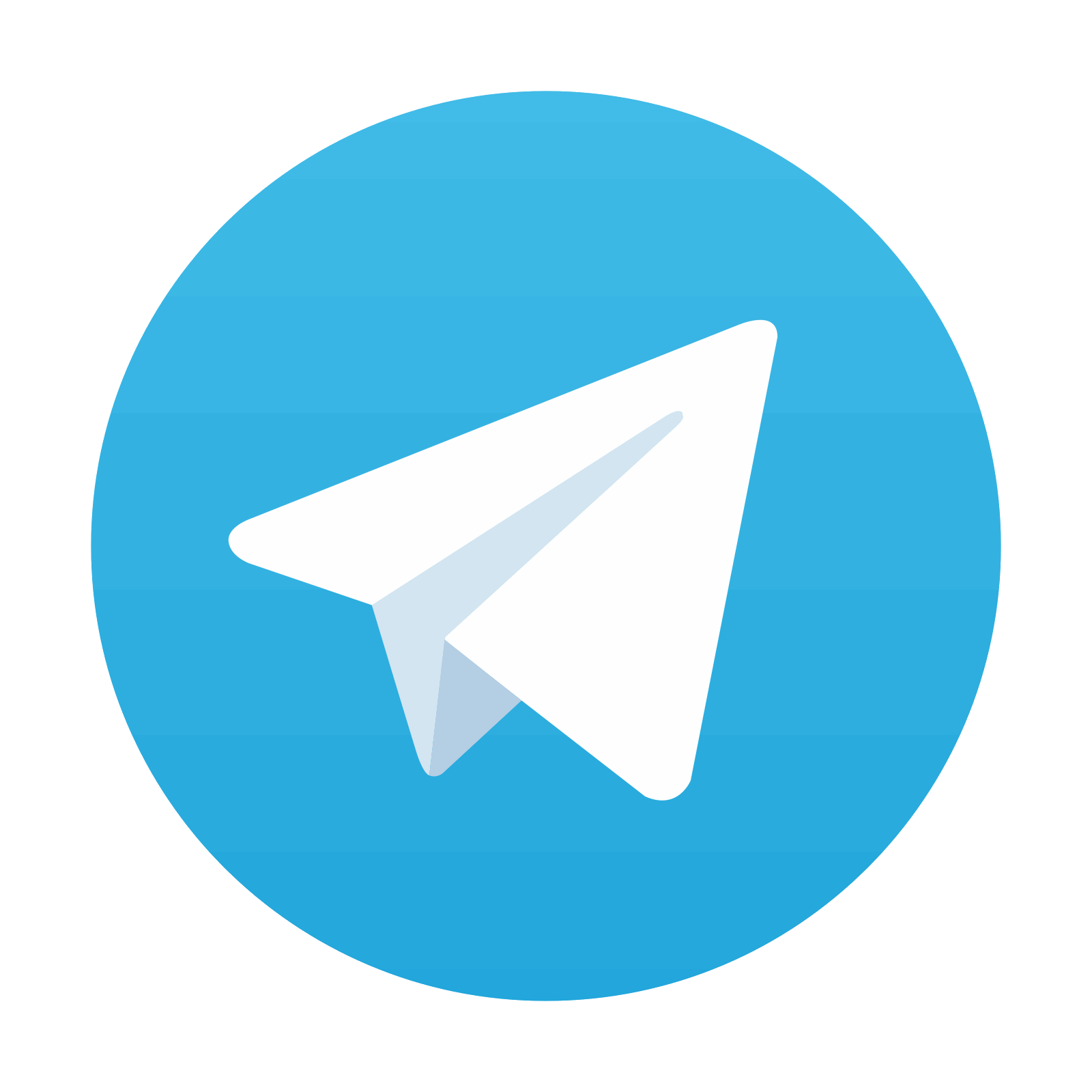
Stay updated, free articles. Join our Telegram channel

Full access? Get Clinical Tree
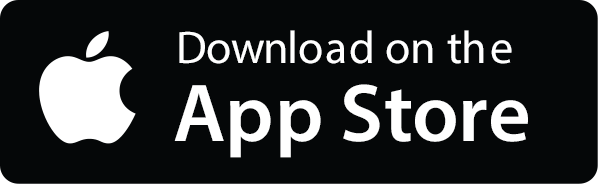
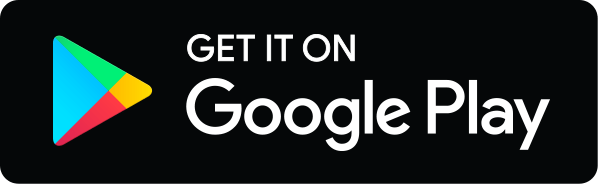
