Introduction
Anterior cruciate ligament (ACL) rupture can be a devastating injury. Even after reconstruction, there is notable risk of knee osteoarthritis development, risk that is increased if ACL tear is accompanied by meniscal injury. Lifetime costs to society of an ACL-injured individual may also be substantial, estimated at around $38,000 for individuals undergoing reconstruction and $88,500 for individuals undergoing rehabilitation. Given the risks associated with ACL injury even after treatment, there has been significant focus on developing prevention programs that may be able to reduce ACL rupture rates; however, there have been mixed results in terms of yielding favorable outcomes. This is likely because mechanisms of ACL rupture remain poorly understood.
Though a wide range of risk factors for ACL rupture have been proposed, this chapter will focus on biomechanical mechanisms associated with injury. While there is no consensus on ACL injury mechanisms, the literature indicates that ACL injury may be the result of a combination of factors, including some of those discussed later.
Anterior Tibial Translation
The ACL is largely considered to be the primary restraint to anterior translation of the tibia. Illustrating this point, Butler et al. found that the ACL gives an average of 86% of resistance to applied anterior tibial displacement. Studies utilizing in vitro loading of cadaveric knees and in vivo strain measurements found that anterior tibial translation appeared to have a large effect on ACL strain at low flexion angles (30 degrees or below). Markolf et al. reported mean ACL force to be as high as 150% of applied force at full extension and 100% near 30 degrees flexion.
Quadriceps activation is one potential source of anterior tibial translation. Nunley et al. and DeFrate et al. found that at lower knee flexion angles, the patellar tendon is oriented anteriorly relative to the tibia, meaning a quadriceps activation could create an anteriorly directed force on the tibia via the patellar tendon. Nunley et al. further proposed that this mechanism may be one reason for the disparity in ACL injury frequencies between females and males, reporting that female subjects had a patellar tendon tibial shaft angle 3.7 degrees higher on average than that of male subjects.
Ground impact can create joint compressive force in the knee, and some authors have suggested that this force may play a role in generating anteriorly directed force on the tibia. Due to the posterior tilt of the tibial plateau, a joint compressive force would lean anteriorly and cause anterior tibial translation, especially when the knee is near full extension. Podraza and White have also suggested that a ground reaction force traveling up the lower limbs, rather than quadriceps activation, may play a role in ACL strain during deceleration. This is because landing with an extended knee, largely considered to be high risk for ACL injury, was not found to be correlated with a greater knee extension moment in their study and was therefore not correlated with greater quadriceps activation. However, despite this apparent lack of correlation between knee extension and quadriceps activation, quadriceps activation is nevertheless essential to maintaining extension during ground impact. Caution should therefore be taken before ruling out quadriceps activation as a source of anterior tibial translation and ACL injury.
While anterior tibial force is largely accepted as an influential factor in ACL rupture, the addition of loads in other planes may further increase ACL strain and risk of injury. Berns et al. found that adding valgus torque to anteriorly directed tibial force generated more ACL strain than anterior force alone. Markolf et al. reported that anterior tibial force together with internal tibial torque in a knee near full extension generated the highest ACL force out of several loading combinations tested in the study.
Hamstring Co-Contraction
The elasticity of the musculature surrounding the knee, including the hamstrings, can passively protect the joint. However, passive protection alone may be insufficient, as joint laxity has been cited as a potential risk factor for ACL injury. In fact, Hashemi et al. proposed that the “inciting event” for ACL injury is a delayed coactivation of the hamstrings and quadriceps and that this lack of muscular protection makes inherent knee laxity a critical factor in anterior tibial translation. Several authors have suggested that active hamstring contraction may offer protection against potentially antagonistic forces on the ACL. This is because hamstring contraction generates a posterior shear force on the tibia and increases posterior cruciate ligament strain, simultaneously reducing the strain on the ACL caused by anteriorly directed force. However, this stabilizing mechanism is limited to certain knee flexion angles (roughly 15–60 degrees) and is notably ineffective at or near full extension, despite potentially increased hamstring moments at lower flexion angles upon landing. Pandy and Shelburne have suggested that this limitation is due to the small angles at which the hamstring muscles attach to the tibia at this level of extension, reducing the total amount of applicable posterior shear force regardless of increased hamstring activation. In fact, activating the hamstrings at knee flexion angles lower than 15 degrees may be counterproductive—the flexion moment created by the activated hamstrings must be offset by increased quadriceps activation to maintain extension, potentially generating even higher anteriorly directed tibial force.
Anterior Tibial Translation
The ACL is largely considered to be the primary restraint to anterior translation of the tibia. Illustrating this point, Butler et al. found that the ACL gives an average of 86% of resistance to applied anterior tibial displacement. Studies utilizing in vitro loading of cadaveric knees and in vivo strain measurements found that anterior tibial translation appeared to have a large effect on ACL strain at low flexion angles (30 degrees or below). Markolf et al. reported mean ACL force to be as high as 150% of applied force at full extension and 100% near 30 degrees flexion.
Quadriceps activation is one potential source of anterior tibial translation. Nunley et al. and DeFrate et al. found that at lower knee flexion angles, the patellar tendon is oriented anteriorly relative to the tibia, meaning a quadriceps activation could create an anteriorly directed force on the tibia via the patellar tendon. Nunley et al. further proposed that this mechanism may be one reason for the disparity in ACL injury frequencies between females and males, reporting that female subjects had a patellar tendon tibial shaft angle 3.7 degrees higher on average than that of male subjects.
Ground impact can create joint compressive force in the knee, and some authors have suggested that this force may play a role in generating anteriorly directed force on the tibia. Due to the posterior tilt of the tibial plateau, a joint compressive force would lean anteriorly and cause anterior tibial translation, especially when the knee is near full extension. Podraza and White have also suggested that a ground reaction force traveling up the lower limbs, rather than quadriceps activation, may play a role in ACL strain during deceleration. This is because landing with an extended knee, largely considered to be high risk for ACL injury, was not found to be correlated with a greater knee extension moment in their study and was therefore not correlated with greater quadriceps activation. However, despite this apparent lack of correlation between knee extension and quadriceps activation, quadriceps activation is nevertheless essential to maintaining extension during ground impact. Caution should therefore be taken before ruling out quadriceps activation as a source of anterior tibial translation and ACL injury.
While anterior tibial force is largely accepted as an influential factor in ACL rupture, the addition of loads in other planes may further increase ACL strain and risk of injury. Berns et al. found that adding valgus torque to anteriorly directed tibial force generated more ACL strain than anterior force alone. Markolf et al. reported that anterior tibial force together with internal tibial torque in a knee near full extension generated the highest ACL force out of several loading combinations tested in the study.
Hamstring Co-Contraction
The elasticity of the musculature surrounding the knee, including the hamstrings, can passively protect the joint. However, passive protection alone may be insufficient, as joint laxity has been cited as a potential risk factor for ACL injury. In fact, Hashemi et al. proposed that the “inciting event” for ACL injury is a delayed coactivation of the hamstrings and quadriceps and that this lack of muscular protection makes inherent knee laxity a critical factor in anterior tibial translation. Several authors have suggested that active hamstring contraction may offer protection against potentially antagonistic forces on the ACL. This is because hamstring contraction generates a posterior shear force on the tibia and increases posterior cruciate ligament strain, simultaneously reducing the strain on the ACL caused by anteriorly directed force. However, this stabilizing mechanism is limited to certain knee flexion angles (roughly 15–60 degrees) and is notably ineffective at or near full extension, despite potentially increased hamstring moments at lower flexion angles upon landing. Pandy and Shelburne have suggested that this limitation is due to the small angles at which the hamstring muscles attach to the tibia at this level of extension, reducing the total amount of applicable posterior shear force regardless of increased hamstring activation. In fact, activating the hamstrings at knee flexion angles lower than 15 degrees may be counterproductive—the flexion moment created by the activated hamstrings must be offset by increased quadriceps activation to maintain extension, potentially generating even higher anteriorly directed tibial force.
Knee Flexion Angle
Several studies have found that low knee flexion angle (roughly 30 degrees or below) is associated with ACL strain and injury. For example, utilizing a unique combination of magnetic resonance imaging (MRI), biplanar fluoroscopy, and motion capture, Taylor et al. found that ACL strain was greatest when the knee was near full extension during both walking and jump landing tasks. Yu et al. have suggested that young females tend to utilize a low flexion landing strategy when compared to young males, which may be one reason for the ACL injury rate disparity between females and males.
Low knee flexion angle may facilitate other mechanisms in straining the ACL. As aforementioned, the patellar tendon has been found to be oriented anteriorly relative to the tibia at low knee flexion angles. Quadriceps activation could therefore create anteriorly directed force on the tibia via the patellar tendon as knee flexion angle decreases. Additionally, knee joint compressive force caused by ground impact could cause anterior tibial translation when the knee is near full extension, especially given that increased knee flexion upon landing has been correlated with decreased ground reaction force. Low knee flexion angle may also limit the protective effects of hamstring co-contraction, given that the hamstrings attach to the tibia at small angles when the knee is near full extension, inhibiting production of high posterior shear force. The quadriceps must also be at an increased activation state to maintain extension, producing increased strain on the ACL.
Knee flexion motion may also play a part in ACL strain. In one particular study, Yu et al. found that knee flexion angular velocity at initial foot contact during a stop-jump maneuver was negatively correlated with ground reaction force upon landing, ground reaction force being potentially deleterious to the ACL via a joint compressive mechanism.
Hip Flexion Angle
The effects of hip flexion angle on ACL strain have not been as well explored as the effects of knee flexion angle, but the existing literature largely suggests that landing on a more extended hip or with decreased hip flexion motion may be associated with higher risk for potentially antagonistic forces on the knee. When the hip joint is flexed, the musculature surrounding the knee may be able to passively produce a posterior tibial force, thereby counterbalancing the anterior tibial force that may strain the ACL. An extended hip may shorten the hamstrings and therefore preclude knee stability.
Shultz et al. used position sensors to find higher anterior tibial force in subjects who exhibited less hip flexion change and more knee flexion change during a drop jump landing, change referring to the difference between peak and initial angles. These results may potentially align with the findings of Yu et al., who have suggested that active flexion motion, distinct from flexion angle, may influence ground impact force. Specifically, hip flexion angular velocity at initial foot contact during a stop-jump task was found to be correlated with decreased peak posterior and vertical ground reaction forces as well as decreased anterior tibial shear force upon landing. Kipp et al. have similarly suggested that active sagittal plane flexion may allow for muscles associated with the knee joint to better absorb landing impact force and other rotational torques.
However, it is important to note that increased hip flexion should not currently be ruled out as a potential risk factor for ACL rupture. In a video analysis study of subjects injuring their ACLs and controls safely engaging in comparable maneuvers, Boden et al. found that a larger hip flexion angle may be high risk for ACL injury. Kipp et al. found that rapid flexion of the hip was related to higher knee abduction torques in a land and cut task. Given the conflicting nature of findings concerning the effects of hip flexion angle on the knee, further study may be necessary to more definitively determine the role of hip flexion in ACL injury.
Knee Valgus/Varus Orientation
In addition to sagittal plane kinematics and heightened quadriceps force during loading, moments in the frontal plane may be a potential mechanism for noncontact ACL injury. The precise planes of knee motion that lead to noncontact ACL injury are not completely clear and remain a controversial issue, the importance of the frontal plane mechanism is often disputed in the literature. Studies have demonstrated that sagittal plane knee angles near full extension combined with a large quadriceps muscle force greatly increase ACL loading; thus many clinicians support the sagittal plane injury mechanism as the predominant factor in noncontact ACL injury. Additionally, Grood et al. demonstrated that movement purely in the frontal plane or transverse plane has less of an effect on ACL strain and that, alternatively, the medial collateral ligament (MCL) demonstrates primary restraint against valgus loading. It has been shown that a valgus force adequate to cause a complete rupture of the MCL does not cause similar injury to the ACL. While women have been shown to demonstrate high knee valgus motion and torque during athletic maneuvers and these movements are associated with higher risk for ACL injury, men also suffer from ACL injury without reduced valgus motion, indicating that additional mechanisms may be a factor. The analysis of bone bruise location from MRIs has also demonstrated that valgus force may not be the primary mechanism for ACL injury. Bone bruises, frequently associated with high-pressure collision between the tibia and femur during ACL rupture, can lend insight into potential injury mechanisms depending on specific compartment locations of the bruises. Using three-dimensional modeling and numerical optimization on MRIs of knees in which bone bruising was apparent on both medial and lateral aspects of the tibia and femur, Kim et al. observed a slight valgus rotation. Wittstein et al. found that the most common tibial contusion pattern following noncontact ACL injury was to have both medial and lateral contusions, with 89.3% of males and 84.4% of females demonstrating bruising in both compartments. Other studies have more specifically demonstrated that bone bruises on the lateral femoral condyle and posterolateral tibia occur with ACL injury, indicating that lateral tibial and femoral compression with medial compartment offloading occurs during injury. Posterolateral tibial plateau bone bruises could be the result of internal tibial rotation, external femoral rotation, valgus, and/or anterior tibial translational motion during injury.
Conversely, Hewett et al. have suggested that an external knee valgus moment is a predominant predictor for ACL injury. Using a laboratory-based prediction algorithm, Hewett et al. demonstrated that females with an increased knee abduction moment when landing from a drop vertical jump were at increased risk of ACL injury. However, using this same algorithm, Goetschius et al. calculated peak knee abduction moment and determined that it was not associated with noncontact ACL injury in the female athletes who participated in the study. Studies using video analyses as well as in vivo biomechanical data have shown that increased knee valgus loads and overall increased movement in the frontal plane may be associated with increased risk of ACL injury. More specifically, video analysis of ACL injuries has suggested that the knee valgus collapse mechanism may be a predominant risk factor. This mechanism, demonstrated by an excessive valgus knee angle during loading as well as an externally rotated tibia relative to the femur and internally rotated hip, is typically predominant among females. The “Q,” or quadriceps, angle is the angle between the force vector of the quadriceps muscle group and the alignment of the patellar tendon and may increase the horizontal component of the quadriceps muscle force, thereby possibly exposing the knee to greater loads. Using videographic analysis, Krosshaug et al. found that dynamic knee valgus collapse was the most common mechanism for ACL injury among both female handball and basketball athletes. However, it is difficult to determine the exact point of ACL rupture using videographic analysis. In the referenced study, the authors noted that subjects landed in a neutral position and then progressed into valgus during the loading phase. Most importantly, the authors noted the presence of valgus knee collapse after the estimated time of rupture. Therefore valgus collapse could be the result of the knee giving way after ACL rupture, rather than the mechanism for injury. Additionally, Nguyen et al. demonstrated greater consistent hip internal rotation moment with concomitant knee external rotation moment during the first 40% of a jump landing. This may not necessarily produce any valgus force on the knee; however, in the coronal plane, there is the appearance of increased valgus after the time of injury.
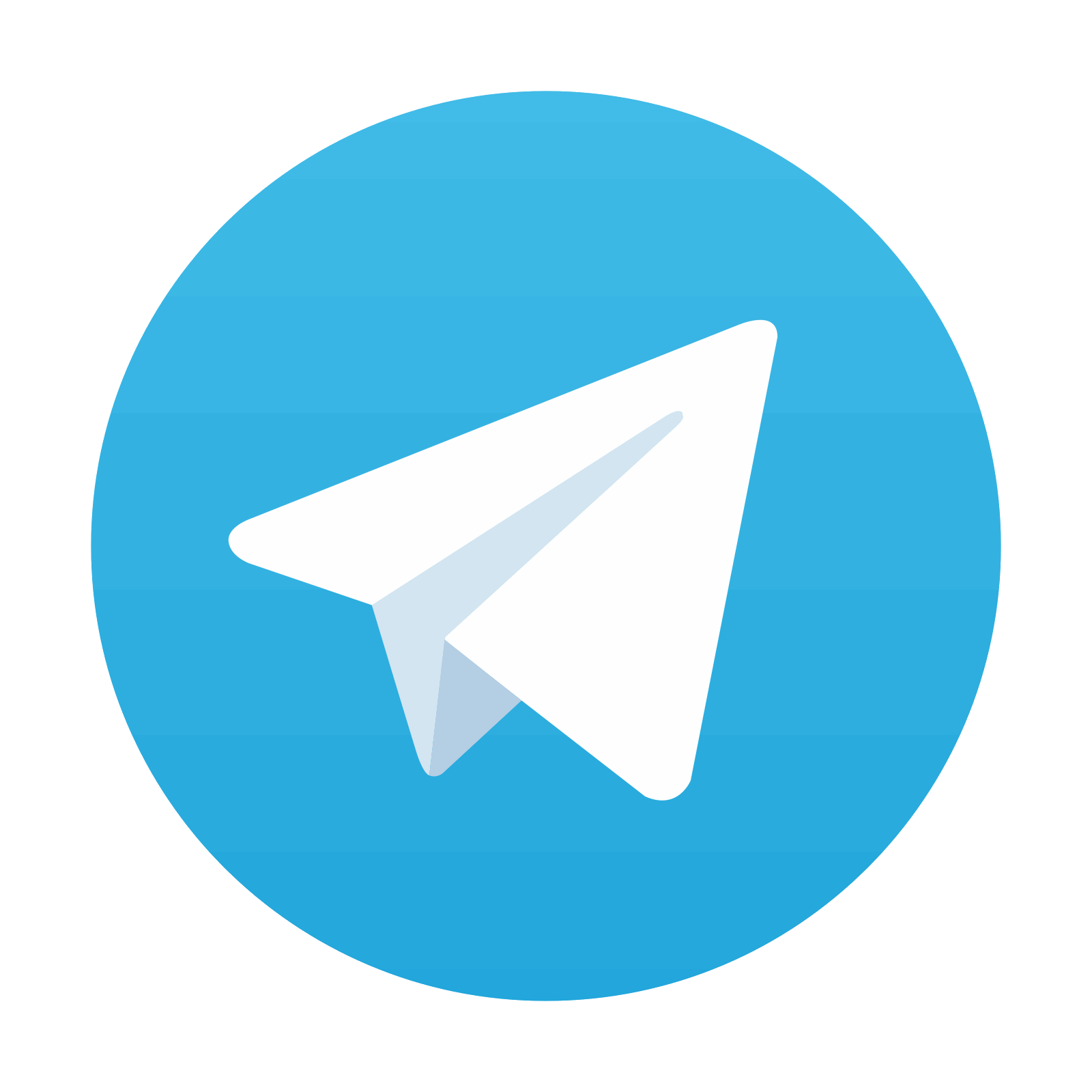
Stay updated, free articles. Join our Telegram channel

Full access? Get Clinical Tree
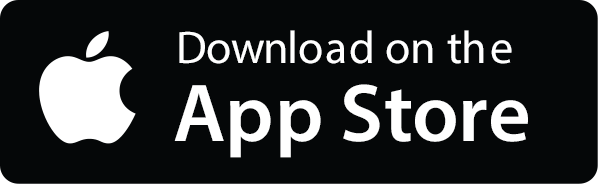
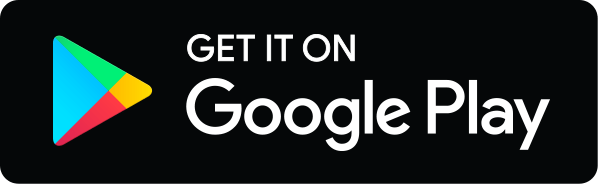