Mast cells arise in the bone marrow, circulate as immature precursors, and develop into functional mast cells after entering peripheral tissues.
The phenotype of mast cells is diverse, plastic, and governed by signals from lymphocytes, fibroblasts, and other elements of the microenvironment.
In healthy tissues, mast cells serve as immunologic sentinels and participate in innate and adaptive immune responses to bacteria and parasites.
Mast cells accumulate in injured and inflamed tissue, where they may amplify or suppress inflammation.
Mast cells have been implicated in multiple immune-mediated diseases, including inflammatory arthritis, although their role as a therapeutic target remains uncertain.
Introduction
Although mast cells are best known for their role in allergy and anaphylaxis, the immune function of this bone marrow–derived lineage far outstrips its participation in immunoglobulin (Ig)E-driven disease. Mast cells are resident broadly in vascularized tissues but cluster near interfaces with the external world in skin and mucosa, as well as in the linings of vulnerable body cavities and near blood vessels and nerves. In these locations, mast cells serve as immune sentinels, equipped with an array of pathogen receptors and an armamentarium of mediators capable of rapidly recruiting immune effector cells. Mast cells also accumulate in sites of tissue injury and chronic inflammation, although their role in such locations remains uncertain. Other functions for this lineage, conserved by evolution for more than 500 million years, continue to be defined.
Circumstantial and experimental evidence implicates mast cells in the pathogenesis of rheumatic diseases. Mast cells reside constitutively in the normal synovium and are found in large numbers in inflamed synovial tissue, whereas mast cell mediators are identified in inflammatory joint fluid. Moreover, models have indicated that mast cells can contribute importantly to the pathogenesis of experimental arthritis. Mast cells have also been implicated in other autoimmune conditions, including multiple sclerosis, bullous pemphigoid, and systemic sclerosis.
Basic Biology of Mast Cells
Development and Tissue Distribution
Mast cells are distinctive in appearance. Ranging in size from 10 to 60 μm and with a centrally located round or oval nucleus, their abundant cytoplasm is filled with multiple small granules. They were named Mastzellen in 1878 by the German pathologist Paul Ehrlich, who believed that they were overfed connective tissue cells ( mästen , in German, means to feed or fatten an animal). Electron microscopy reveals that the plasma membrane of the mast cells exhibits multiple thin cytoplasmic extensions, providing a broad interface with the surrounding tissue ( Fig. 15.1A ). The tissue distribution of mast cells is extensive; within tissue, mast cells tend to cluster around blood vessels and nerves and near epithelial and mucosal surfaces. They are also found in the lining of vulnerable body cavities such as the peritoneum and the diarthrodial joint. Given this localization, mast cells are among the first immune cells to encounter pathogens invading into tissue from the external world or via the bloodstream, consistent with their role as immune sentinel cells.

Mast cells are of hematopoietic origin, arising in the bone marrow and depositing in tissues after migrating through the bloodstream ( Fig. 15.2 ). Unlike other myeloid cells, such as monocytes and neutrophils, mast cells do not terminally differentiate in the bone marrow but rather circulate as rare progenitors (0.005% of leukocytes), bearing the surface signature CD34 + /c-Kit + /FcεRI + . Further developmental details have been worked out most extensively in the mouse. A burst of circulating progenitors late in gestation suggests the possibility that mast cells may populate the tissues primarily during early development, with later recruitment restricted to the inflammatory context, as appears to be the case for certain populations of murine tissue macrophages. After entering the tissues, murine mast cells may mature into classic granulated cells or remain as ungranulated progenitors, awaiting local signals to differentiate fully. Comparison of murine lung and intestine has demonstrated that these tissues use distinct pathways to regulate the constitutive and inducible recruitment of mast cell progenitors, illustrating that mast cell homing is a precisely controlled process.

Once resident in tissues, mast cells may live for many months. Mature mast cells remain capable of mitotic division, although recruitment of circulating progenitors appears to greatly exceed local replication as a pathway to expand the number of tissue mast cells. Mechanisms of reducing mast cell numbers include apoptosis, as demonstrated in tissue mast cells deprived of the cytokine stem cell factor (SCF), a critical survival signal for mast cells. Under certain conditions, mast cells can also emigrate via the lymphatics, appearing in draining lymph nodes much in the manner of dendritic cells. The pathways that license mast cell accumulation in inflamed tissues are incompletely defined but include tissue production of factors such as IL-4 and IL-33 that attenuate mast cell apoptosis.
Mast Cell Heterogeneity: Common Progenitor, Multiple Subsets, Phenotypic Plasticity
Whereas all types of mast cells derive from a common progenitor lineage, the phenotype of fully differentiated tissue mast cells is heterogeneous. Human mast cells are conventionally divided into two broad classes on the basis of the protease contents of their granules (see Fig. 15.2 ). MC TC display rounded granules containing the enzymes tryptase and chymase, whereas the smaller and more irregularly shaped granules of MC T contain tryptase but not chymase. MC TC also express other proteases, including carboxypeptidase and cathepsin G. These subtypes differ in tissue distribution. MC TC tend to be found in connective tissue, such as in normal skin, muscle, the intestinal submucosa, and the normal synovium, whereas MC T predominate in mucosal sites, including the lining of the gut and respiratory tract, although in fact both are present in many locations. Beyond the protease signature, other differences between these subsets include their profile of cytokine elaboration, as well as cell surface receptor expression; however, tissue-specific phenotypic differences are noted within each type as well (i.e., neither MC TC nor MC T are homogenous cellular subsets).
The relationship between MC TC and MC T mast cells is controversial. Are they committed subsets, akin to CD4 and CD8 lymphocytes, or functional states that mast cells assume under the influence of the microenvironment? In mice, where an analogous distinction exists between connective tissue mast cells (CTMCs) and mucosal mast cells (MMCs), evidence for phenotypic plasticity is strong. Both in culture and in vivo, single CTMCs may differentiate into (or give rise to) MMCs and vice versa. Mast cells with intermediate protease expression are found, and serial observations suggest that exposure to an inflammatory stimulus can induce progressive change from one class to another, although whether this occurs at a single-cell level has not been definitively established. Similarly, in murine and human mastocytosis, clonally expanded mast cells display divergent phenotypes depending on the tissue of residence. Immunoreceptors such as FcγRIIb are expressed by human mast cells in some tissues but not others. In mice, gene expression signatures differ between mast cells harvested from different locations, although broad patterns remain similar, distinguishing these cells clearly from other lineages (including basophils). In aggregate, these data favor the hypothesis that mast cells assume their phenotype under the control of local signals but can change their phenotype in accordance with local conditions.
Stem Cell Factor
One of the most important signals from tissue to local mast cells is SCF. The receptor for SCF, c-Kit, is expressed widely on hematopoietic lineages early in differentiation, but among mature lineages only mast cells express c-Kit at a high level. Stimulation of mast cells by SCF promotes maturation and phenotypic differentiation, blocks apoptosis, and induces chemotaxis. It may also activate mast cells directly to release mediators. In both mice and humans, SCF remains an irreplaceable survival signal for tissue mast cells. Accordingly, mice with defects in SCF or c-Kit are strikingly deficient in mature tissue mast cells (examples include W/W v , Sl/Sl d , and W sh strains). Similarly, clonal mast cells obtained from patients with systemic mastocytosis commonly exhibit activating mutations in KIT .
SCF occurs in two alternate forms resulting from differential mRNA splicing: soluble and membrane bound. The importance of this latter form is clear from Sl/Sl d mice, which lack only the membrane-bound isoform yet exhibit very few tissue mast cells. SCF is synthesized by multiple lineages, including mast cells themselves. Expression by fibroblasts is especially important, given the intimate physical contacts observed between fibroblasts and mast cells in situ. The SCF/c-Kit axis mediates cell-cell adhesion between mast cells and fibroblasts independent of the kinase activity of the receptor. Rodent mast cells co-cultured with fibroblasts demonstrate enhanced survival, connective-tissue phenotypic differentiation, and heightened capacity to elaborate pro-inflammatory eicosanoids, effects mediated at least in part by direct contact, including interactions between SCF and c-Kit. The extent of similar regulation in human mast cells is uncertain. Expression of SCF has also been documented on other lineages including macrophages, vascular endothelium, and airway epithelium and is likely a critical pathway by which tissues modulate the local mast cell population.
T Lymphocytes and Other Cells
T lymphocytes exert a profound effect on mast cell phenotype. Severe combined immunodeficiency (SCID) mice that lack T cells fail to develop mucosal mast cells, a defect that can be corrected by T cell engraftment. An analogous observation has been made in humans deficient in T cells due to congenital or acquired immunodeficiency. Intestinal biopsy in these patients shows that MMCs (MC T ) are strikingly reduced, whereas CTMCs (MC TC ) are present in normal numbers. The pathways by which T cells exert this striking effect are not defined, although T cell cytokines such as IL-3, IL-4, IL-6, IL-9, and transforming growth factor (TGF)-β may have profound effects on the phenotype of mast cells matured in culture. By contrast, interferon (IFN)-γ inhibits mast cell proliferation and may induce apoptosis. These observations imply that cells recruited to an inflamed tissue may impact the phenotype of local mast cells. The rheumatoid synovium may well exemplify this phenomenon; normally populated by MC TC mast cells, large numbers of MC T mast cells are identified in the inflamed synovium, typically in regions rich in infiltrating leukocytes, whereas MC TC mast cells reside in deeper, more fibrotic areas of the joint. Interestingly, regulatory T cells (Tregs) can also directly impact mast cell function, including recruitment, receptor expression, degranulation, and cytokine production.
Other cells aside from T cells can interact with mast cells in the tissues. Fibroblasts and mast cells commonly demonstrate close physical interactions. Beyond SCF, fibroblasts elaborate cytokines such as the IL-1 family member IL-33, which can exert effects on mast cell protease expression, effector phenotype, and survival. Dendritic cells have also been implicated in the recruitment of mast cells into inflamed tissues.
Different Functions for MC T and MC TC Mast Cells
The preservation of distinct types of mast cells in multiple species implies distinct roles for these subtypes. However, the understanding of functional differences between MC T and MC TC remains limited. One hypothesis is that MC T play a pro-inflammatory role and MC TC specialize in matrix remodeling. This hypothesis makes sense of (1) the promotion of MC T development by T cells patrolling the tissues; (2) the partition of MC T and MC TC mast cells to inflamed and fibrotic areas, respectively; and (3) the preferential expression of the pro-inflammatory mediators IL-5 and IL-6 by MC T and the profibrotic IL-4 by MC TC . Not all observations fit comfortably into this dichotomy, however. For example, the potently pro-inflammatory anaphylatoxin receptor C5aR (CD88) is expressed on MC TC but not on MC T . Ultimately, too little is known about the actual functional importance of these subsets to permit firm conclusions.
Mast Cell Activation
Immunoglobulin E
The canonical pathway to mast cell activation is via IgE and its receptor FcεRI. With a K a of 10 10 L/M, this receptor is essentially constantly saturated with IgE at typical serum concentrations, to which perivascular mast cells are exposed through direct sampling of the luminal contents. Such binding not only sensitizes mast cells to the target antigen but also helps promote mast cell survival and cytokine production. Crosslinking FcεRI-bound IgE by multivalent antigen, and in some circumstances even by monovalent antigen, induces a brisk and vigorous response. Within minutes, granules within the mast cell fuse together and with the surface membrane, creating a set of labyrinthine channels that allow rapid release of granule contents (see Fig. 15.1B ). This compound exocytosis event, termed anaphylactic degranulation , is followed within minutes by the elaboration of eicosanoids newly synthesized from arachidonic acid cleaved from internal membrane lipids. Alternately, for cell-bound antigen opsonized by IgE, mast cells can disgorge their granules directly upon the targeted cell in a structure termed an antibody-dependent degranulatory synapse . Signals transduced via FcεRI also induce the transcription of new genes and elaboration of a wide range of chemokines and cytokines ( Fig. 15.3 ). Both degranulation and mediator production exhibit partial dependence on a rapid switch of mast cell metabolism in favor of glycolysis. Upon termination of the stimulation event, the surface membrane closes over the granule-formed channels, which subsequently bud off within the cytoplasm, re-creating discrete granules using the original membranes. These granules are then recharged with mediators in a process that occurs gradually over days to weeks.

Immunoglobulin G and Immune Complexes
IgE is only one among many pathways of mast cell activation. One key trigger for mast cell activation in both humans and mice is IgG, acting via receptors for the Fc portion of IgG (FcγR). The importance of this pathway was demonstrated first in mice rendered genetically deficient in IgE. Contrary to expectations, these animals remain susceptible to anaphylaxis mediated through IgG and the low-affinity IgG receptor FcγRIII. The human counterpart of this receptor, FcγRIIa, is equally capable of inducing activation of human mast cells. Human mast cells can also be induced to express the high-affinity IgG receptor FcγRI, rendering them susceptible to IgG-mediated activation.
IgG receptors contribute to involvement of mast cells in antibody-driven diseases. Thus, in the mouse, mast cells participate in IgG-mediated immune complex peritonitis, the cutaneous Arthus reaction, and experimental murine bullous pemphigoid. Activation via Fc receptors also mediates mast cell participation in antibody-mediated murine arthritis.
Soluble Mediators and Cell-Cell Contact
Mast cells coordinate with immune and nonimmune lineages via mechanisms beyond antibody response, including soluble mediators and surface receptors. Examples of such signals include the cytokine TNF and the neurogenic peptide substance P, which can induce mast cell degranulation. Peptide secretagogues (including substance P) and many pharmaceutical compounds that elicit pseudo-allergic reactions (e.g., morphine) activate mast cells via the G-protein-coupled receptor MRGPRX2. Mast cells can be activated by complement, including the anaphylatoxin C5a. Physical contact with other cells can also induce mast cell activation. For example, CD30 on lymphocytes can interact with CD30L on mast cells to induce production of a range of chemokines. Interestingly, ligation of CD30L does not induce release of granule contents or lipid mediators, illustrating the selectivity of response of which mast cells are capable.
Danger and Injury
Mast cells are equipped to recognize danger in the absence of guidance from other lineages via a range of pathogen receptors, including multiple Toll-like receptors (TLRs) and CD48, a surface protein recognizing the fimbrial antigen FimH. These receptors are implicated in the response of mast cells to pathogens and could potentially contribute to mast cell engagement in diseases such as atopic dermatitis, in which skin becomes abnormally colonized with bacteria. Mast cells can also be activated by the complement-derived anaphylatoxins C3a and C5a. Mast cells can respond directly to physical stimuli including trauma, temperature, and osmotic stress. Finally, mast cells can be triggered by danger signals released by injured bystander cells, such as IL-33 and uric acid crystals. Together, these receptors enable mast cell involvement in a broad range of immune and nonimmune processes.
Inhibitory Signals for Mast Cells
As with other immune lineages, mast cells are subject to both negative and positive regulation. Cytokines that inhibit aspects of mast cell function include TGF-β and IL-10. Inhibitory receptors on the mast cell surface include the IgG receptor FcγRIIb and the phosphatidyl serine receptor CD300A. The importance of these receptors is demonstrated in genetically deficient animals. Mice lacking FcγRIIb demonstrate a striking propensity to activation via both IgG and IgE (which binds with low affinity to both FcγRIIb and FcεRI). Ligation of FcγRIIb via IgG directed against allergens may blunt IgE-mediated mast cell activation, accounting in part for the efficacy of immunotherapy in allergic disease. CD300A mediates suppression of mast cell activation by apoptotic debris. Modulating the surface expression of inhibitory receptors is a potentially important regulator of the activation threshold for mast cells in tissues.
Mast Cell Mediators
Granule Contents: Proteases, Amines, Proteoglycans, and Cytokines
Mature mast cells package a range of mediators in their granules that are ready for immediate release through fusion with the surface membrane. The most abundant of these are the neutral proteases, named for their enzymatic activity at neutral pH, but vasoactive amines, proteoglycans such as heparin, and pre-formed cytokines play distinct roles in the biologic consequences of mast cell degranulation. The release of these mediators is not all or none. In addition to anaphylactic degranulation, mast cells may release only a few granules at a time in a process termed piecemeal degranulation . Mast cells can release one type of granule but not another. Alternately, mast cells may be induced to elaborate cytokines and chemokines without any release of granule contents, as illustrated by activation via CD30L, and may release their proteases packaged in microvesicles budded from the cell surface. Thus, although the mast cell is well equipped to release large volumes of pre-formed mediators, it is equally capable of responses tailored to the activating stimulus.
Tryptase
Named for its enzymatic similarity to pancreatic trypsin, tryptase is the most abundant granule protein in human mast cells. It is also a specific marker for mast cells, synthesized in scant amounts by basophils but by no other lineage. The enzyme found in granules is the β isomer, which is the product of two distinct genes and is enzymatically active upon formation of a homotetramer that relies on the scaffolding function of the proteoglycan heparin. Mast cells also synthesize α tryptase, a protein incapable of forming homotetramers and thus enzymatically inactive. Unlike β tryptase, the α isomer is not stored in granules but is constitutively released into circulation, where its function is unknown. The distinction between tryptase isomers is important for diagnostic reasons: as a marker of degranulation, systemic levels of β tryptase constitute a marker of recent anaphylaxis. By contrast, α trypsin levels reflect total body mast cell load and serve as a useful biomarker in systemic mastocytosis.
Tryptase cleaves structural proteins such as fibronectin and type IV collagen and enzymatically activates stromelysin, an enzyme responsible for activating collagenase. Fibrinogen is another substrate, potentially implicating mast cells in prevention of fibrin deposition and blood coagulation in the tissues. Tryptase also promotes hyperplasia and activation of fibroblasts, airway smooth muscle cells, and epithelium. Cleavage of protease-activated receptors such as PAR2 may contribute to some of these activities, although other studies document PAR2-independent tryptase activation of mesenchymal cells. In aggregate, these effects suggest an important role for tryptase in matrix remodeling. A further contribution to the inflammatory milieu is suggested by the capacity of tryptase to promote neutrophil and eosinophil recruitment and to cleave C3, C4, and C5 to generate anaphylatoxins. Interestingly, tryptase can potentially suppress inflammation by cleaving IgE and IL-6.
Chymase
This chymotrypsin-like neutral protease is found in the MC TC subset of human mast cells, packaged within the same granules as tryptase. Like tryptase, chymase can cleave matrix components and activate stromelysin, although it can also activate collagenase directly, suggesting a role in matrix remodeling. Chymase can activate angiotensin I, leading to angiotensin converting enzyme (ACE)-independent activation of the potent vasoconstrictor angiotensin II. Chymase also affects cytokine function, cleaving pro-IL-1β to generate active cytokine and inactivate pro-inflammatory cytokines such as IL-6 and TNF, as well as “alarmins,” including heat shock protein 70 and IL-33.
β-Hexosaminidase
This enzyme is found in lysosomes from many cell types. In mast cells it is abundant in secretory granules, where it is released into the surrounding environment during degranulation. Recent studies have found that this enzyme is capable of degrading bacterial cell peptidoglycan, and release by mast cells plays an important role in the defense against experimental staphylococcal infection in mice.
Vasoactive Amines
Human mast cells are capable of synthesizing and storing the biogenic amines histamine and serotonin, which are implicated in vascular leak. Histamine, by far the more abundant, is a vasoactive amine found in both MC T and MC TC , as well as other lineages. Histamine is involved in the wheal-and-flare response to cutaneous allergen challenge via augmented vascular permeability, transendothelial vesicular transport, and neurogenic vasodilation. These effects are mediated principally via the H1 receptor. Three other histamine surface receptors, H2, H3, and H4, are distributed widely on immune and nonimmune lineages, with effects as diverse as gastric acid secretion, Langerhans cell migration, and B cell proliferation. Another important mechanism underlying mast cell-induced vascular leak is heparin-mediated bradykinin production (as discussed in the following section).
Heparin and Chondroitin Sulfate E
These large proteoglycans enable the ordered packing of mediators within human mast cell granules. Negatively charged carbohydrate side chains complex tightly with positively charged proteins, enabling the accumulation of very high concentrations of β tryptase and other proteases. Heparin, produced exclusively by mast cells, facilitates the activity of tryptase by making possible proteolytic self-activation within the granule and stabilizing the active tetrameric form of this enzyme. Heparin also has a wide range of effects beyond the mast cell. It is potently angiogenic. Heparin binding activates antithrombin III, providing the basis for use as an anticoagulant, while inhibiting chemokines and both classic and alternative pathways of complement activation, as well as the function of Treg cells. The physiologic role of these extra-cellular activities is uncertain. Of more evident in vivo relevance is that the negatively charged surface of heparin enables activation of factor XII. This zymogen then activates kallikrein, an enzyme that in turn cleaves high-molecular-weight kininogen to generate bradykinin, a potent mediator of vascular leak. This mechanism contributes to the antihistamine-resistant edema observed in patients with hereditary angioedema from lack of C1 esterase inhibitor, an inhibitor of activated factor XII and kallikrein.
Pre-formed Cytokines and Chemokines
Mast cells store certain mediators in their granules for rapid release. The first of these to be documented was TNF. In the mouse, this pool of TNF is implicated in the rapid recruitment of neutrophils to the peritoneum during peritonitis. Exocytosed granules appear to release TNF gradually into the environment, enhancing the immunostimulatory effect of the cytokine as TNF-containing granules reach draining lymph nodes, helping promote development of the mature immune response. Other cytokines that may be stored in granules include IL-4, IL-16, IL-17A, basic fibroblast growth factor (bFGF), and vascular endothelial growth factor (VEGF). Stored chemokines include the neutrophil chemoattractants CXCL1 and CXCL2.
Newly Synthesized Mediators: Lipid Mediators, Cytokines, Chemokines and Growth Factors
Beyond pre-formed mediators, activated mast cells generate a range of mediators de novo. These mediators are released minutes to hours after stimulation, broadening and extending the impact of activated mast cells on surrounding tissues.
Lipid Mediators
Within minutes of activation, mast cells begin to release metabolites of membrane phospholipids. This process is rapid because the relevant enzymes, beginning with phospholipase A2, which is responsible for harvesting phospholipids from the outer leaflet of the nuclear membrane, are already present in the cytoplasm and need only to be activated through signals mediated by calcium flux and the phosphorylation of intra-cellular messengers. The hallmark prostaglandin of human mast cells is prostaglandin D 2 (PGD 2 ), which induces bronchoconstriction, vascular leak, and neutrophil recruitment. Smaller amounts of other prostaglandins, as well as thromboxane, are also made. Mast cell-derived leukotrienes (LTs) have similar but generally more potent activity. LTC 4 is the major leukotriene species generated by human mast cells, and, together with its metabolites LTD 4 and LTE 4 , serves as a potent inducer of vascular leak. Smaller amounts of the chemotaxins LTB 4 and platelet-activating factor (PAF) are also generated. The profile of lipid mediators produced by mast cells changes with local environmental signals and the resulting state of differentiation. Thus mast cells from skin generate PGD 2 in excess of LTC 4 , whereas both species are elaborated in roughly equal proportion by mast cells isolated from lung and osteoarthritic synovium.
Cytokines, Chemokines, and Growth Factors
Within hours of activation, mast cells begin to elaborate newly synthesized mediators as the end result of induced gene transcription and translation. The range of such mediators is broad (see Fig. 15.3 ). They include the canonical pro-inflammatory mediators TNF, IL-1, and IL-6; the Th2 cytokines IL-4, IL-5, IL-10, and IL-13; the “alarmin” IL-33; chemotactic factors including IL-8, macrophage inflammatory protein (MIP)-1α, CXCR1, CXCR2, and RANTES (regulation upon activation normal T cell expressed and presumably excreted); and growth factors for fibroblasts, blood vessels, and other cells such as bFGF, VEGF, and platelet-derived growth factor (PDGF). IL-17 is detected in tissue mast cells and can be generated by mast cells stimulated in culture. As noted earlier, some of these cytokines may also be stored pre-formed in granules for rapid release. The panel of mediators generated depends on the state of differentiation, as well as the activating signal, and may occur in the absence of degranulation.
Role of Mast Cells in Health and Disease
The understanding of the role of mast cells in health and disease has been aided greatly by the availability of mice that lack mast cells entirely or are deficient in mast cell–specific products such as heparin or granule proteases. These mice are viable, excluding an obligate role for mast cells in the structure and function of most tissues. Yet when they are under physiologic stress, such as that imposed by experimental models of disease, multiple differences from wild-type mice become evident. In many cases, these abnormalities may be corrected by engraftment with cultured mast cells, directly implicating mast cells in a remarkably broad range of disease processes ( Table 15.1 ). The extrapolation of such experiments to human disease is limited by multiple factors. Most evidently, mice are not humans, and the experimental systems employed typically model at best only certain aspects of the corresponding human condition. Further, many of these mice exhibit “off-target” phenotypes outside of the mast cell lineage, complicating interpretation of the data, especially when results in one mast cell–deficient mouse strain diverge from those observed in another. These discrepancies have occasioned considerable controversy in the mast cell literature. However, together with in vitro experiments using human mast cells, and careful study of individuals in different states of health and disease, animal experiments have contributed greatly to the understanding of mast cell physiology and pathophysiology.
Beneficial to Host | Harmful to Host |
Angiogenesis | Anaphylaxis∗ |
Anxiety control | Arthritis∗ |
Bacterial cystitis | Aortic aneurysm∗ |
Bacterial peritonitis∗ | Asthma∗ |
Bladder epithelium exfoliation∗ | Atherosclerosis∗ |
Bone remodeling | Atopic dermatitis |
Brain trauma | Atrial fibrillation |
Dengue fever | Autoinflammatory disease |
Dermatitis∗ | Bacterial cystitis |
Envenomation∗ | Burn |
Glomerulonephritis∗ | Bullous pemphigoid∗ |
Graft tolerance∗ | Cardiac fibrosis |
Intestinal epithelial barrier∗ | Cardiomyopathy |
Lung infection, bacterial∗ | Chronic obstructive pulmonary disease |
Lung infection, viral∗ | Colitis |
Parasites, intestine∗ | Colon polyps |
Parasites, muscle | Cystic fibrosis |
Parasites, skin | Dermatitis, irritant∗ |
Peptic ulcer disease | Dermatitis, sunburn |
Thromboembolism | Gastritis |
Tumor suppression∗ | Glomerulonephritis∗ |
Wound healing∗ | Gout |
Immune complex peritonitis∗ | |
Ischemia-reperfusion injury | |
Kidney injury∗ | |
Lung fibrosis | |
Malaria | |
Multiple sclerosis∗ | |
Myocardial infarct size | |
Myositis∗ | |
Neurogenic inflammation∗ | |
Obesity∗ | |
Peritonitis, irritant∗ | |
Peritoneal adhesions | |
Pneumonitis | |
Renal fibrosis∗ | |
Renal ischemia-reperfusion | |
Retinopathy∗ | |
Scleroderma | |
Sepsis∗ | |
Tumor angiogenesis |
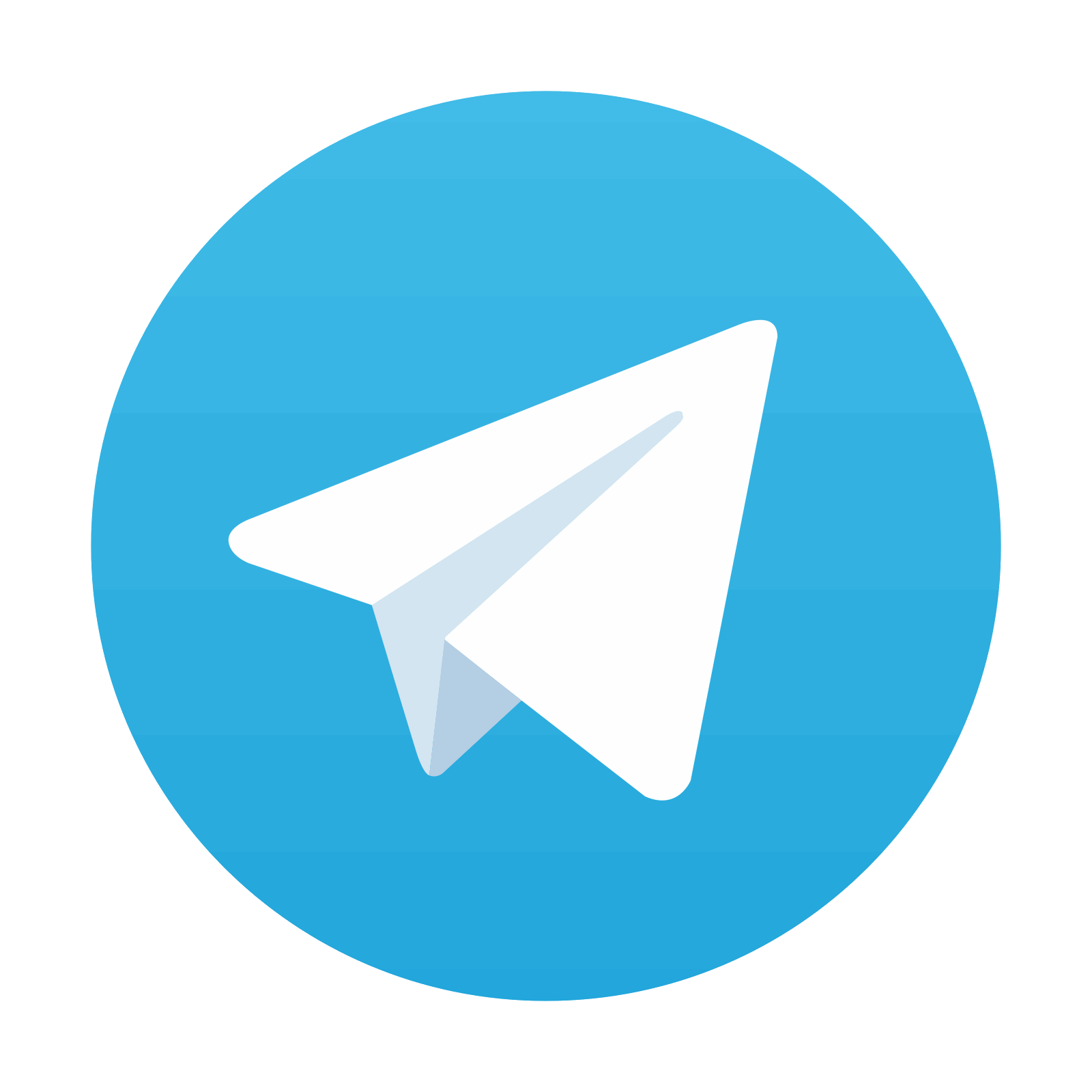
Stay updated, free articles. Join our Telegram channel

Full access? Get Clinical Tree
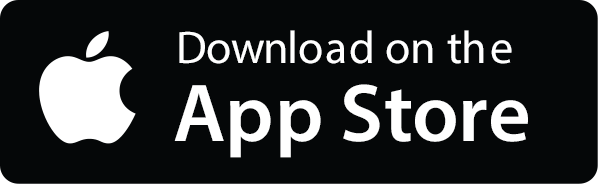
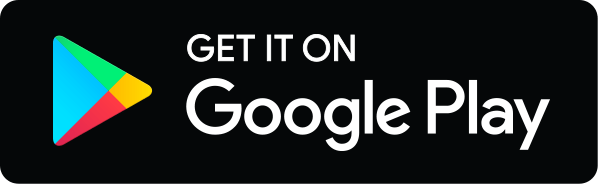
