CHAPTER OBJECTIVES
At the end of this chapter, the learner will be able to:
Differentiate between the components of the lymphatic and the venous system.
Relate the function of each lymph system component to the formation of lymphedema.
Diagnose lymphedema according to cause, pathophysiology, and stage.
Differentiate between lymphedema and chronic venous insufficiency.
Define the components of Starling’s Law and describe their role in lymphatic flow.
Select the appropriate compression therapy for a patient with peripheral lymphedema.
Design an exercise program for a patient with lymphedema.
Discuss the principles of manual lymphatic mobilization, as well as the indications and contraindications.
Educate patients on skin care and strategies to prevent recurrent lymphedema complications.
Lymphedema is a chronic inflammatory condition that develops as a result of lymphatic insufficiency. Lymphatic insufficiency occurs from a decrease in reabsorption or a decrease in transport capacity of the lymphatic system. It can be primary malformation of the lymph system or an acquired condition due to obstruction or damage to the system (TABLE 5-1).
Primary Lymphedema Causes | Onset | Secondary Lymphedema Causes | Onset |
Inherited genetic mutations resulting in abnormal development of lymph vessels:
Genetic mutations with autosomal dominant pattern:
| Variable age onset depending upon the gene involved* | Damage to lymph nodes and/or lymph vessels that results in decreased lymphatic reabsorption and transportation (mechanical insufficiency) Trauma Radiation therapy Tumor obstruction Infection CVI | Any time after damage to lymph nodes and vessels: Either soon after lymph node dissection or months, years, or decades later Depends on patient medical history, co-morbidities (number of lymph nodes removed, radiation therapy, chemotherapy, venous pathologies, and obesity), and general health |
Milroy’s disease (congenital lymphedema) FOXC2 VEGEFR3 SOX18* | Onset during infancy* | Surgery Trauma Radiation therapy Tumor obstruction (cancer) Infection CVI | Depends on patient medical history, comorbidities, and general health |
Meige’s disease (lymphedema praecox) MIM153200* | Onset in childhood or around puberty; may begin in early 20s or 30s | ||
Lymphedema tarda | Onset at 35 years or later |
The lymphatic system is interrelated with all of the other systems of the body. Its primary roles include conducting immune system surveillance, assisting the cardiovascular system to maintain fluid homeostasis, and aiding the digestive system in the breakdown of long-chain fatty acids. The immunological functions involve both the immediate response to pathogens and the long-term resistance to repeated exposure to pathogens.
The body’s immunological function is performed by the lymphoid organs, including the spleen, thymus, tonsils, Peyer’s patches located in the small intestine, bone marrow, and lymph nodes (FIGURE 5-1).
FIGURE 5-1
Anatomy of the immune system
Spleen provides lymphocyte proliferation, immune surveillance, and immune response; removes old or damage platelets and blood cells from the blood; removes debris and foreign matter from the blood. It has other functions not related to the lymph system that are not discussed here.
Thymus secretes thymosin and thymopoietin, hormones that enable T-lymphocytes to become activated against specific pathogens; it does not directly fight the antigens.
Tonsils capture and remove pathogens in the airway during inhalation.
Peyer’s patches, structurally similar to tonsils, are located in small intestines. They destroy bacteria in the intestines and generate “memory lymphocytes” for long-term immunity.
Bone marrow, also called myeloid tissue, is responsible for production of all the cells of the immune system through the process of hemopoiesis. The stem cells become common myeloid stem cells or common lymphoid stem cells that further differentiate into red blood cells, platelets, and white blood cells.
Lymph nodes are the biological filter station for antigens present in lymph fluid; responsible for purifying and draining lymph fluid.

The lymphatic system is a body-wide network of superficial and deep vessels connected by perforating vessels, similar to the venous system. It is comprised of lymph capillaries, lymphatic precollectors, collectors, ducts, and trunks (FIGURE 5-2). The superficial vessels, located directly under the skin and above the fascia, drain the dermis and subcutaneous tissues. The deep vessels are located below the fascia and drain all the tissue deep to the fascia. There are connecting vessels that perforate the fascia to transport the fluid from the deep vessel system to the superficial vessel system, which is the opposite of how the venous system functions. The lymphatic vessels are similar to arteries in that the larger collecting vessels have smooth muscle in the wall, and they are similar to the veins in that the collecting vessels contain valves that allow for unidirectional flow of the fluids.
The superficial system begins with the lymphatic capillaries that appear much like a fishnet underneath all of the skin (FIGURE 5-3). The capillaries are the smallest-diameter vessels and thus have the largest numbers. As the diameter of the more proximal lymphatic vessels increases, the number of lymphatic vessels decreases. Lymph capillaries have anchoring filaments that attach to the basement membrane between the dermis and epidermis (FIGURE 5-4). The lymph capillaries are more permeable than the vascular capillaries and therefore can absorb larger molecules of protein and fat. Each lymph capillary is composed of a single layer of overlapping flat endothelial cells, and the overlapping junction is called the inlet junction. The capillary does not contain valves, thus allowing the fluid to move in any direction within the capillary system.
FIGURE 5-3
Lymphatic capillaries that intertwine with the arterial and venous capillary bed Molecules and fluid flow from the arterial capillary bed into the tissue spaces (black arrows) and from the interstitial spaces into the initial lymphatic capillary, termed reabsorption (green arrows). Lymph fluid then flows from the initial lymph capillaries into slightly larger vessels, termed precollectors. Fat and protein molecules that are too large to enter the venous capillaries must be reabsorbed by the initial lymphatics or initial lymph capillaries.

FIGURE 5-4
Anchoring filaments of the lymphatic capillaries The initial lymphatic capillary is connected to the basement membrane by anchoring filaments. When the interstitial pressure gradient (ie, concentration of protein molecules) is high, tension is exerted on the anchoring filaments, which results in pulling the single layer of overlapping endothelial cells. This tension opens the filament and allows the fluid to enter the initial lymphatic capillary where the pressure is low.

The capillaries merge and become precollectors, which have a larger diameter and therefore a lower pressure, thus facilitating movement of the fluid from the capillary to the precollector. The precollectors also have the overlapping endothelial anatomy that allows them to absorb interstitial fluid, and they have both a limited number of valves and a limited smooth muscle structure that allows them to initiate transportation of the collected fluids.
All of the precollectors merge to become larger diameter vessels, termed collectors. The collectors first bring the lymph fluid to the lymph nodes and then carry the remaining fluid to the larger lymphatic trunks. The anatomical structure of the collectors is similar to the construction of the veins in that they have three distinct layers (intima, media, and adventitia). They have well-defined valves that permit flow to go in only one direction—toward the heart. Within the lymph collector, the vessel segment between two valves is termed the lymphangion (FIGURE 5-5). Each lymphangion has an autonomic-driven resting contraction rate of approximately 10–12 contractions per minute.1 This is further discussed under physiology. The superficial lymphatic collectors are located in the subcutaneous fatty hypodermis and they follow a direct route toward the regional lymph nodes. The deep lymphatic collectors follow the pathway of larger blood vessels. Lymph collectors drain lymph fluid from specific areas of the body, creating lymphatic “territories” that drain to specific regional lymph nodes. These lymphatic territories are defined by lymphatic watersheds that tend to delineate the body into specific collection and drainage patterns (FIGURE 5-6). Although there is some cross-connection between the territories via the watersheds, most of the fluid flows directly to its own regional lymph nodes. If there is fluid overload in one area, some of the fluid can be diverted to another region via the watershed connections.
CLINICAL CONSIDERATION
The removal of any regional lymph nodes results in a reduced ability for the immune system to respond to foreign pathogens and an increased risk of developing infection.
FIGURE 5-6
Lymphatic watersheds The body is divided into lymph territories that allow drainage of the lymph flow from specific body regions to specific regional lymph nodes. The deep vessels do not cross between watersheds (areas of collection); however, there are some superficial vessels that cross the watershed boundaries and thereby divert lymph fluid from one quadrant to another when there are conditions of overload. The superficial pathways that cross between watersheds can be encouraged with manual lymphatic mobilization to direct more flow to open areas; however, it is usually inadequate to remove the majority of the fluid in the affected quadrant.

The lymph nodes (estimated to be about 700) are located in clusters throughout the body (FIGURE 5-7). The lymph node is bean-shaped and surrounded by a dense fibrous capsule (FIGURE 5-8). The collectors converge at the convex side of the lymph nodes where they are termed afferent lymph vessels. The fluid moves through the cortex of the node, into the medulla, and exits on the concave side via efferent vessels that then become trunks. The number of efferent vessels is less than the number of afferent vessels, resulting in a decreased transportation rate through the lymph nodes, and thus allowing the immune system cells time to phagocytose the pathogens that are in the lymphatic fluid.
CLINICAL CONSIDERATION
Lymph nodes become enlarged when they try to contain and destroy pathogens such as bacteria or cancer cells.
FIGURE 5-7
Clusters of lymph nodes throughout the body Lymph nodes are located strategically throughout the body, in conjunction with the superficial anastomoses that allow fluid to cross the watershed boundaries. The lymph system naturally diverts fluid across the watershed during times of overload; however, in lymphedema it is insufficient to manage the total volume of fluid needing to be transported. Manual lymphatic mobilization stimulates these anastomoses to increase the rate of flow.

FIGURE 5-8
Lymph node anatomy A greater number of afferent vessels bring fluid to a lymph node and a lesser number of efferent vessels leave the lymph node. This anatomical arrangement permits a slower rate of lymph transport through the lymph nodes and thus allows time for the immune system to phagocytose bacteria, waste products, and dead cells. For individuals with cancer who undergo a lymph node dissection, the rate of flow through the regional lymph node is automatically decreased. This sets the stage for potential lymphatic congestion in the affected quadrant and may lead to lymphedema.

When the superficial and deep collectors converge, the vessels are termed trunks. The trunks are similar in construction to the collectors except they are larger in diameter and contain more smooth muscle in the vessel wall (FIGURE 5-9). The trunks are named as follows: right and left lumbar trunks, gastrointestinal trunk, jugular trunk, supraclavicular trunk, subclavian trunk, peristernal trunk, and bronchial-mediastinal trunk. The right and left trunks and the gastrointestinal trunk converge in the anterior area of T-11-L2 to form the cisterna chyli, which is the beginning of the thoracic duct. The thoracic duct ascends cephalically along the anterior vertebral column and perforates the diaphragm.
CLINICAL CONSIDERATION
Diaphragmatic breathing provides a force to promote lymphatic drainage from the thoracic duct into the venous angle. This results in a negative pressure gradient within the thoracic duct, therefore promoting distal-to-proximal lymph transport.
The only direct connection between the lymphatic system and the venous system occurs where the thoracic duct (on the left) and the right lymphatic duct enter the venous angles. The venous angle is formed by the convergence of the internal jugular and subclavian veins (FIGURE 5-10). This is the anatomical termination of what is described as the lymphatic system.
FIGURE 5-10
Venous angle The venous angle is formed by the junction of the internal jugular vein and the subclavian vein. The thoracic duct on the left and the right lymphatic duct insert directly into this junction, which is termed the venous angle. The venous angle flows into the brachiocephalic vein.

The purpose of the lymphatic capillaries is to absorb interstitial fluid and molecules, and when the fluid enters the capillaries, it is termed lymphatic fluid. The endothelial cell junctions overlap, creating inlet valves, so that when the interstitial protein concentration increases, tension on the anchoring filaments causes the inlet valves to open and allows absorption of the larger fat and protein molecules. These inlet valves remain open as long as the interstitial pressure (a result of plasma protein concentration) remains higher than the pressure within the lymph capillary.
CASE STUDY
INTRODUCTION
Mr. CB is an 80-year-old male who presents with bilateral lower extremity edema (left greater than right) that has recently worsened and is preventing him from playing golf. Past medical history includes history of myocardial infarct with residual cardiomyopathy (15 years ago), chronic renal insufficiency, Type 2 diabetes for which the patient takes oral medications, history of gout in the left great toe, and history of prostate cancer that was diagnosed 6 years ago and is being treated by “watchful waiting,” meaning there is no treatment except yearly monitoring of PSA levels (FIGURE 5-11A, B).
DISCUSSION QUESTIONS
What questions are appropriate to ask the patient in order to obtain the subjective history?
What additional objective information is needed to determine the cause of the edema?
What tests and measures are indicated to determine the plan of care for this patient?
Fluid movement between the capillaries (arterial, venous, and lymphatic) and the interstitial spaces is governed by Starling’s Law of Equilibrium. This law describes how the osmotic and hydrostatic pressures in the capillaries and interstitial tissue determine the direction of fluid movement. The irony of this equation is that equilibrium is never really achieved because of the dynamic nature of the human body, which does not allow the pressure gradient to equal zero; therefore, fluid is always moving, or in physiologic terms, fluid is constantly being filtrated and reabsorbed at the capillary bed (FIGURE 5-12).2,3
The difference between the net hydrostatic pressure and the net osmotic pressure is the force that determines the direction of fluid flow (TABLE 5-2). When the fluid flows from the interstitial space into the capillaries, it is termed reabsorption and can occur at both the venule and the lymphatic capillaries. When the fluid flows from the capillaries into the interstitial space, it is termed filtration. Equilibrium occurs when an equal amount of fluid moves from the arterial capillaries into the interstitial space and out of the interstitial space into the venous and lymphatic capillaries. Hydrostatic pressure is the pressure exerted by the blood on the capillary walls. Because the pressure is higher on the arterial side than on the venule side, fluid is pushed out of the capillary bed into the interstitial space. Osmotic pressure is the force created by large molecules such as plasma proteins, and it opposes hydrostatic pressure. Plasma protein molecules attract water, so that in a normal system they facilitate reabsorption of the fluids back into the venule.4
Capillary bed forces—Forces responsible for direction of fluid movement at the capillary bed membrane. There are 4 forces: 2 hydrostatic and 2 oncotic pressures that affect trans-capillary fluid exchange at the capillary bed. |
Hydrostatic pressure (HP)—Pressure exerted on the vessel wall by the fluid (water). |
Capillary hydrostatic pressure (HPc)—Pressure from water that drives fluid out of the capillary (ie, filtration); is highest at the arteriolar end of the capillary and lowest at the venular end. |
Interstitial hydrostatic pressure (HPi)—Pressure generated by the volume of water in the interstitial spaces. As more fluid filters into the interstitium, both the volume of the interstitial space and the hydrostatic pressure within that space increase. |
Net hydrostatic pressure (net HP)—Difference between the capillary HP (HPc) and the interstitial HP (HPif). |
Osmotic pressure (OP)—Pressure exerted by plasma protein molecule concentration that is required to prevent the flow of water across a semipermeable membrane via osmosis. |
Capillary osmotic pressure (OPc)—Pressure that drives fluid into the capillary bed. The capillary membrane is readily permeable to ions; therefore, the osmotic pressure within the capillary is determined by the presence of plasma proteins (that are relatively impermeable to the capillary membrane). This pressure is referred to as the “oncotic” pressure or “colloid osmotic” pressure because it is generated by colloids. Albumin generates about 70% of the oncotic pressure, which is typically 25–30 mmHg. |
Interstitial osmotic pressure (OPi)—Pressure of the interstitial fluid that depends on the interstitial plasma protein concentration and the reflection coefficient of the capillary wall. The more permeable the capillary barrier is to proteins, the higher the interstitial oncotic pressure. This pressure is also determined by the amount of fluid filtration into the interstitium. |
Reabsorption—Process by which fluid moves from the interstitial spaces into the capillary bed. |
Filtration—Process by which fluid moves from the capillary bed into the interstitial spaces. Starling’s hypothesis states that “the fluid movement due to filtration across the wall of a capillary is dependent on the balance between the hydrostatic pressure gradient and the oncotic pressure gradient across the capillary.” The four Starling’s forces are:
The balance of these forces allows calculation of the net driving pressure for filtration. Under normal physiological conditions at the capillary bed, the exchange of fluids, nutrients, cellular debris, and waste products is primarily due to diffusion and filtration between the blood and tissues. Net filtration of fluid across the capillary membrane is governed by the balance of filtration forces and reabsorption forces. The direction of fluid movement is dependent upon the difference between hydrostatic pressure (HP) and osmotic pressure (OP). HP is the same as capillary blood pressure, which varies along the length of capillary, and under normal circumstances that pressure is lower in the venous end and higher in the capillary end. |
Fluid is forced OUT of arterial end of capillary bed when: Net hydrostatic pressure (net HP) = HPc – HPif > Net osmotic pressure (net OP)OPc– OPif
|
Fluid is forced INTO the venous end of the capillary when: Net hydrostatic pressure (net HP) = HPc – HPif < Net osmotic pressure (net OP)OPc– OPif
To determine the resultant amount of fluid movement across the entire capillary bed: Net filtration pressure = difference between:
If the resultant difference is positive, the fluid is moved into the interstitial spaces. If the resultant difference is negative, the fluid is reabsorbed into the capillary bed. The one component missing from Starling’s Law of Equilibrium is the lymphatic capillary. If the lymphatic capillary is healthy and functioning normally, the lymphatic capillary will reabsorb the protein molecules that escape at the arterial end of the capillary bed. If this process is functioning, there is no net buildup of interstitial pressure. However, if there is lymphatic insufficiency or overload, the normally negative pressure gradient within the initial lymphatic becomes positive (overload of protein molecules), at which point the initial lymphatics can no longer absorb the protein molecules. The result is the accumulation of protein molecules within the interstitial spaces. This will alter both the HPif and OPif pressure gradients of the capillary bed. Protein molecules attract water and are too large to enter the venous capillary. With a positive pressure in the lymphatic capillary preventing reabsorption into the lymphatic system, the protein molecules plus water accumulate in the interstitial spaces and eventually cause the skin to stretch, resulting in visible edema. Filtration is normally not affected. |
The blood capillary wall is a semipermeable membrane, thus a force is required to move the larger protein molecules in and out of the capillary bed. At the arterial end where the net hydrostatic pressure is higher than the net osmotic pressure, the molecules are forced out of the capillary bed and into the interstitium. However, at the venule end the net osmotic pressure is higher than the net hydrostatic pressure so the pressure is not sufficient to move the molecules from the interstitium into the capillary bed. Therefore, the molecules are reabsorbed into the lymphatic capillary at the overlapping endothelial junction. When this process is in balance so that fluids are adequately moved, no edema occurs. When there is an imbalance, for a variety of reasons and in either direction, edema develops. All edema pathology can be related to a problem with either filtration or reabsorption. When this happens, there is a transportation dysfunction—the freeway is clogged. The challenge to the clinician is to determine the pathology that prevents adequate flow of the fluid back into the central vascular system.
Primary lymphedema is a result of congenital malformations of the lymphatic system, although the consequences may not be observed in the early years (FIGURES 5-13 and 5-14). Secondary lymphedema is a result of acquired damage to the lymph vessels or nodes and subsequent impaired reabsorption and/or transportation of lymphatic fluid (FIGURE 5-15). Refer to TABLE 5-1 for more details of both types.
FIGURE 5-14
A. Lymphedema praecox (anterior view). B. Lymphedema praecox (posterior view) Anterior and posterior views of Stage II primary lymphedema as a result of cancer and its treatment. Note that the contour of the left lower extremity is proportionate even though the size discrepancy is obvious.

A frequent cause of secondary lymphedema is chronic venous insufficiency (FIGURE 5-16).5,6 The sequence of events is as follows: The venous system becomes compromised and cannot accommodate the normal amount of venous flow. The lymphatic system, when healthy, can compensate because it can increase its rate of lymphangion contraction up to 10 times its resting volume and transport the fluid normally carried by the venous system.1 For edema to develop in patients with chronic venous insufficiency, one of two scenarios must occur. Either the lymphatic and venous load exceeds the maximum transport capacity of the lymphatic system or the lymphatic system becomes impaired and cannot transport the usual fluid load. The lymphatic impairment may be caused by incompetent lymphatic valves due to distended vessels.7,8
FIGURE 5-16
Chronic venous insufficiency with secondary lymphedema Secondary lymphedema is frequently a consequence of chronic venous insufficiency. The hemosiderin stains (a byproduct of autolysis of hemoglobin released from red blood cells trapped in the interstitial tissue) are characteristic of chronic venous insufficiency. The pedal edema on the dorsum of the foot is also characteristic of secondary lymphedema.

In the United States, the most recognized cause of lymphedema is related to cancer and its treatment (FIGURE 5-17).9 The location and the severity of oncology-related lymphedema are influenced by the area affected, the location and total dosage of radiation, the extent of lymph node dissection, obesity, and presence of co-morbidities.10,11 Clinical presentation can vary drastically and treatment has to be individualized. Other causes of secondary lymphedema include trauma, infection, surgery in which the lymphatic vessels are severed or damaged, and systemic disorders (eg, liver, kidney, or cardiac disease) (FIGURES 5-18 to 5-20). However, any pathology that results in damage to the lymphatic system with decreased transport capacity will result in chronic secondary lymphedema, and clinical presentation will be essentially the same with variations only in relation to the severity of the damage.
FIGURE 5-17
Lymphedema as a result of cancer and radiation (head and neck cancer) Lymphedema as a result of radiation can occur in any body part where the lymphatic vessels are damaged and the flow is interrupted. Note the changes in the skin, termed dry desquamation, in which the epidermal layer is sloughing much like superficial sunburn.

FIGURE 5-18
Secondary lymphedema due to trauma The tight, orange-peel texture of the lower leg skin is a result of lymphedema that has developed after a lateral ankle injury. Any trauma that disrupts the lymphatic flow, limits joint range of motion, and alters gait cycle can result in lymphedema formation.

FIGURE 5-19
Secondary lymphedema due to orthopedic surgery Secondary lymphedema is common after lower extremity surgeries that interfere with lymphatic flow through the popliteal or inguinal lymph nodes, such as total knee or total hip replacements. Early mobilization, isometric exercises, and compression with short stretch bandages are recommended to prevent or treat this type of edema. Reduction of the edema through these strategies will subsequently result in better functional outcomes.

FIGURE 5-20
Secondary lymphedema due to systemic disorder Bilateral lymphedema may be indicative of a systemic disorder (eg, congestive heart failure as in the case of this patient). The erythema of both lower legs is suggestive of cellulitis, which would be treated with antibiotics. Compression with short stretch bandages is recommended after both the congestive heart failure and the cellulitis have been treated.
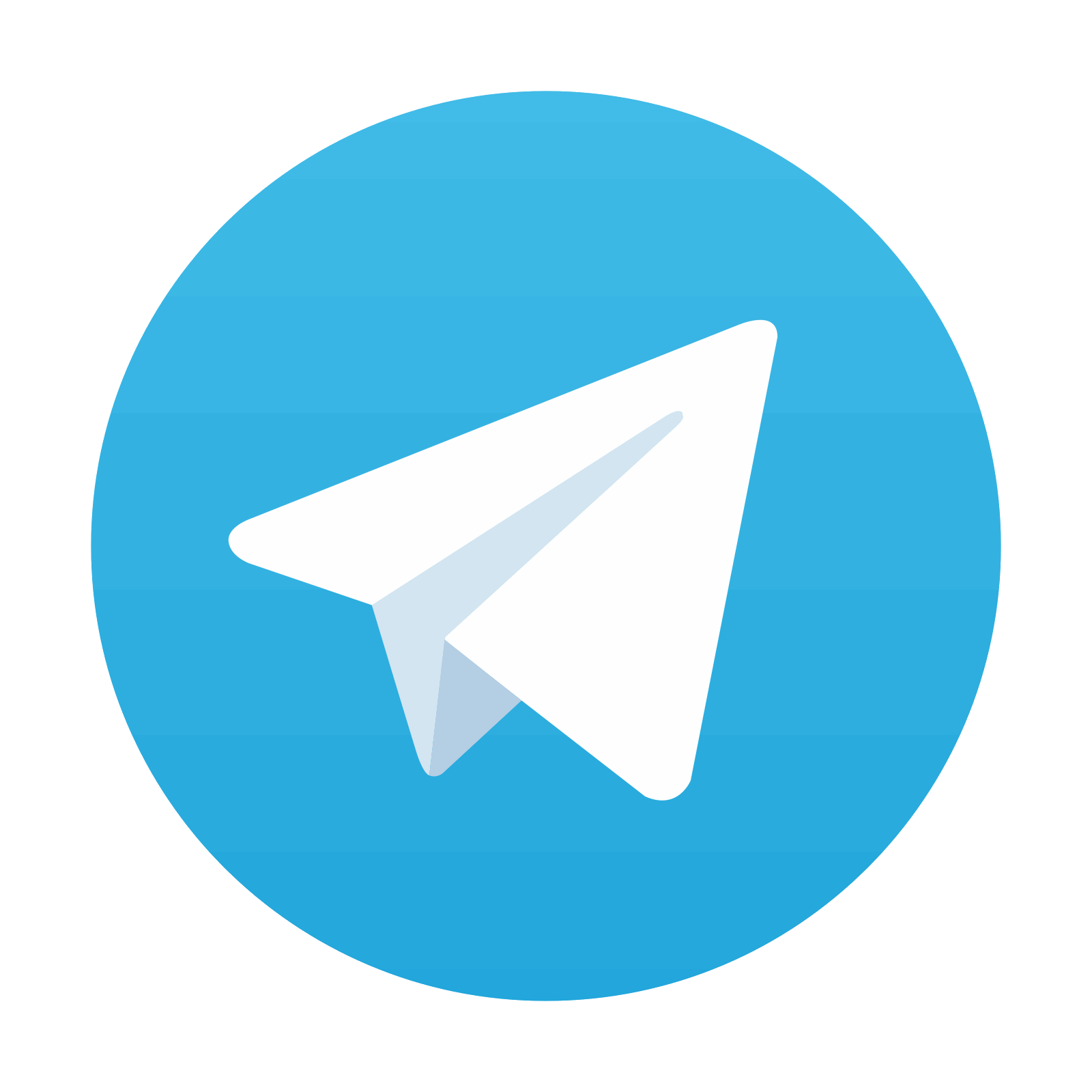
Stay updated, free articles. Join our Telegram channel

Full access? Get Clinical Tree
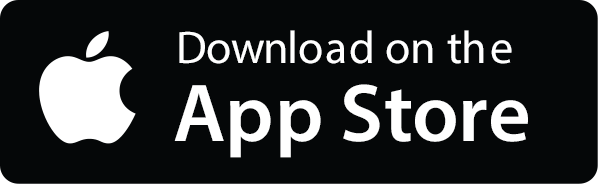
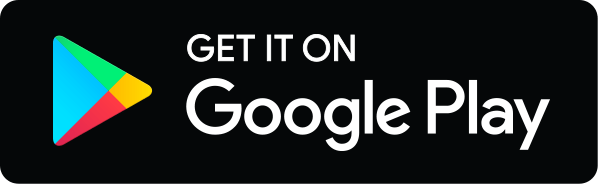
