Lumbar Spondylosis, Degenerative Disk Disease, and Radiculopathy
Brad A. Webb, DO
Jeffrey S. Fischgrund, MD
Jad G. Khalil, MD
Dr. Webb or an immediate family member has received nonincome support (such as equipment or services), commercially derived honoraria, or other non-research-related funding (such as paid travel) from DePuy, A Johnson & Johnson Company and Stryker. Dr. Fischgrund or an immediate family member serves as a paid consultant to or is an employee of FzioMed, Relievant, and Stryker; has stock or stock options held in Understand.com; has received research or institutional support from Smith & Nephew and Stryker; and serves as a board member, owner, officer, or committee member of the Cervical Spine Research Society, and the Lumbar Spine Research Society. Dr. Khalil or an immediate family member is a member of a speakers’ bureau or has made paid presentations on behalf of Johnson & Johnson, Spinewave, and Stryker; serves as a paid consultant to or is an employee of Camber Spine, Innovasis, Johnson & Johnson, and Stryker; has stock or stock options held in Johnson & Johnson, and Medtronic; has received research or institutional support from Centinel Spine, Innovative surgical designs, Johnson & Johnson, Limiflex, Medtronic, and Relievant; and serves as a board member, owner, officer, or committee member of the American Academy of Orthopaedic Surgeons.
Keywords: intervertebral disk degeneration; lumbar facet joint osteoarthritis; spinal nerve compression
INTRODUCTION
Low back pain (LBP) is a complicated disorder and is a leading cause of disability worldwide. The causes of LBP include an extensive differential diagnoses, of which degenerative changes within the lumbar bony anatomy and intervertebral disks play a significant role. Lumbar spine spondylosis can be an associated cause of LBP and is a common condition with an estimated prevalence ranging from 40% to 85%.1 Lumbar spondylosis is due to a degenerative cascade that has an association with intervertebral disk degeneration (IDD) (Figure 1). Mechanical progression and associated disk space narrowing leads to adjacent level pedicle approximation with narrowing of the superior-inferior dimensions of the intervertebral foraminal canal. Laxity of the associated ligaments and vertebral column translate into altered loading mechanics and an altered pressure relationship on the vertebral bone and joint surfaces, which is believed to influence both osteophyte formation and facet joint hypertrophy.2 IDD is a complicated multifactorial process characterized by altered biomechanics of loading, an imbalance of extracellular matrix synthesis and degradation, increased secretion of proinflammatory cytokines, and increased apoptosis and senescence in the nucleus pulposus (NP) cells.3,4 These altered biomechanics lead to further degenerative changes and osteophyte formation. This has the potential to cause lumbar central and foraminal stenosis leading to symptomatic nerve compression and radiculopathy.
LUMBAR DEGENERATIVE DISK
The human spine possesses 23 intervertebral disks that separate the vertebrae and provide flexibility. They account for about 20% to 30% of the length of the spine and increase in size from the cervical to the lumbar regions.5 The disk contains two regions, a more central region—the gelatinous NP, which is surrounded by a fibrous ring, the anulus fibrosus (AF) (Figure 2). Each vertebral body has cartilage end plates,
which are a thin layer of hyaline cartilage tissue that separate the adjacent vertebrae. The composition of the two intervertebral disk regions differs in their cellular types. The NP consists mainly of a high concentration of proteoglycans and water, which are surrounded by a loose type II collagen network. In the NP, the collagen fibrils assume a random orientation and are interspersed in the matrix, which is rich in proteoglycans and water; this is an ideal organization for resisting compressive forces induced with axial loading of the spine.5,6 The AF has a low proteoglycan and water content and a high concentration of type I collagens as well as a small concentration of type II collagens, organized into concentric lamellae.5,6 The AF is described as possessing 20 to 25 lamellae, which are rich in collagen fibrils arranged in a parallel fashion (Figure 3). In each adjacent lamella, the collagen fibrils along the axis are fashioned in the opposite direction as to create an alternating pattern between the lamellae. This arrangement is best suited for resisting the tensile forces seen in both bending and twisting of the spine.7 There are also a set of cells within the disk that help maintain the homeostasis by producing and maintaining proteoglycans, collagen, and matrix cells.
which are a thin layer of hyaline cartilage tissue that separate the adjacent vertebrae. The composition of the two intervertebral disk regions differs in their cellular types. The NP consists mainly of a high concentration of proteoglycans and water, which are surrounded by a loose type II collagen network. In the NP, the collagen fibrils assume a random orientation and are interspersed in the matrix, which is rich in proteoglycans and water; this is an ideal organization for resisting compressive forces induced with axial loading of the spine.5,6 The AF has a low proteoglycan and water content and a high concentration of type I collagens as well as a small concentration of type II collagens, organized into concentric lamellae.5,6 The AF is described as possessing 20 to 25 lamellae, which are rich in collagen fibrils arranged in a parallel fashion (Figure 3). In each adjacent lamella, the collagen fibrils along the axis are fashioned in the opposite direction as to create an alternating pattern between the lamellae. This arrangement is best suited for resisting the tensile forces seen in both bending and twisting of the spine.7 There are also a set of cells within the disk that help maintain the homeostasis by producing and maintaining proteoglycans, collagen, and matrix cells.
The composition of the intervertebral disk varies not only by location, but also with age. The content of water and the proteoglycan concentration within the disk increases when progressing from the AF to the NP. In contrast, the content of collagen within the disk decreases from the outer anulus to the nucleus. With increasing age the proteoglycan and water content of the nucleus decrease. In relation to the level of the spine, the composition of the disk will also vary, with the collagen content of the nucleus being the highest in the cervical disks and lowest in the lumbar disks. There is an opposite trend when evaluating the proteoglycan content.8,9
DISK MATRIX AND CELLULAR CHANGES
The extracellular matrix of the NP contains a high concentration of proteoglycans, with aggrecan the most abundant. In a mature disk, aggrecan possesses both chondroitin sulfate and keratin sulfate chains bound to the same core protein. The synthesized aggrecan interacts with hyaluronan to form the proteoglycan aggregates.10 In healthy disks, the proteoglycans produce a negative charge, which generates an osmotic potential; this potential is converted into biomechanical hydrostatic pressure through the attraction of water.11 The water content of the disk is dependent on both the concentration of proteoglycan and collagen contents. Proteoglycans provide the swelling pressure required to increase the water content and the collagen providing the resistance to swelling.11,12 The functional ability related to the proteoglycan and collagen content has been shown to decline with age. Other proteoglycan aggregate components, such as hyaluronan and matrix proteins, also display concentration and structural changes with age. Hyaluronan is found throughout the disk and its concentration increases with age, but the exact mechanism for the resultant accumulation is not entirely understood.13 Link
proteins are also present throughout the disk, but it is found that their concentration decreases with age in contrast to that of hyaluronan. Along with this, the proteolytic process of the link proteins increases with aging and degeneration.14
proteins are also present throughout the disk, but it is found that their concentration decreases with age in contrast to that of hyaluronan. Along with this, the proteolytic process of the link proteins increases with aging and degeneration.14
Further evaluation of the population of matrix proteins contains a group of both fibrous and cartilaginous connective tissue proteins whose function is similar as found in other sites outside of the vertebral disks. Two main proteins of note are fibronectin and elastin. Little has been reported on the exact function or cause from the degradation of these two proteins. It has been determined that fibronectin increases as we age and will exhibit an increase in degradation fragmentation, with these fragments thought to cause an increase in the catabolic events of disk degeneration.15 Elastin is found in the elastic fibers within the intervertebral disk. Elastin constitutes only about 2% of the dry weight of the anulus; however, it contributes greatly to the overall functioning of the normal healthy disk. The orientation and structure have been evaluated, but it is not clear how degradation of the intervertebral disk influences the structure and function of elastin as well as the overall affect elastin has on the degeneration process of the disk.16
Intervertebral disks are believed to undergo a degenerative cascade, which is proposed to initiate with the introduction of proinflammatory mediators, degenerative enzymes, and a decrease in nutritional support, with genetic and biomechanical influences. This leads to the development of painful tears of the outer disk anulus with end plate separation, leading to a potential compromise in disk nutrition and waste removal. Small annular tears coalesce to form larger radial tears that potentiate disk protrusion and impact the ability of the intervertebral disk to maintain water, leading to subsequent disk desiccation and reduced disk height.17 The loss of disk height leads to mechanical integrity loss, which potentiates progressive disk changes in resorption, and internal disruption.18
Intervertebral disk degeneration results from degradation of matrix macromolecules leading to early features of proteoglycan loss in the regulation of disk hydration and load-bearing properties. Proteoglycan biochemistry, which helps to determine disk osmolarity and hyaluronan content and support proteoglycan aggregation, is important to normal disk function.19
Within the degenerating intervertebral disk, there is a progressive increase in the expression of inflammatory cytokines like interleukin-1 (IL-1) and tumor necrosis factor-α (TNF-α).20,21 It has been shown that TNF-α induces multiple cellular responses in relation to the proinflammatory cascade, which includes the decrease expression of both aggrecan and type II collagen genes. This leads to a decreased accumulation and overall synthesis of aggrecan and collagen, with an increased expression of MMPs and ADAMTS (A Disintegrin and Metalloproteinase with Thrombospondin Motifs) enzymes. Together these cellular responses lead to a loss of extracellular matrix and proteoglycan content within the NP.4
It has been evaluated that proteolysis is considered a major component of the degenerative processes that takes place in the disk. It has been proposed that matrix metalloproteinases (MMPs) play a significant role in the extracellular matrix degeneration, and an imbalance between their activation and inhibition leads to a probable cause of disk degeneration.22 The family of MMPs account for much of the degradation of collagen, aggrecan, and link proteins. The ADAMTS family of metalloproteinases, particularly ADAMTS-4 and ADAMTS-5, has a high affinity for aggrecan and are considered aggrecanases23,24 (Figure 4).
ETIOLOGY OF INTERVERTEBRAL DISK DEGENERATION
A factor that leads to the progression of disk degeneration is the decrease in nutrient supply, which leads to the loss of cell viability and failure to synthesize macromolecules.25 The supply of nutrients is mainly provided by the blood vessels at the disk-end plate interface where the blood vessels penetrate the subchondral space at the level of the vertebral end plate. The nutrients diffuse from the blood vessels, through the cartilaginous end plate and the extracellular matrix of the adjoining disk to provide nutrition to the associated cells.25,26 Declining nutritional transport appears to be a critical event responsible for the changes of the NP cellular matrix. As an intervertebral disk undergoes disk degeneration, the blood supply to the periphery of the disk declines. This leads to a decrease in waste product removal and accumulation of degraded matrix macromolecules, which in turn decreases water content within the central disk27,28 (Table 1).
Abnormal mechanical loads are also thought to provide a pathway to disk degeneration. There have been some evaluations of biomechanical and loading forces that influence intervertebral disk cell and matrix vitality. Studies have shown that intervertebral disks which under go prolonged underloading, overloading, or static loading forces are more susceptible to unfavorable matrix changes and disk degeneration.29,30,31
Several studies suggest that the factors that lead to disk degeneration may have important genetic components. Several studies suggest that factors contributing to disk degeneration may have important genetic components.32 Aggrecan can be more susceptible to disk degeneration as a result of changes to its functional composition, particularly the chondroitin sulfate content. It has been postulated that gene polymorphism within the aggrecan gene, specifically short alleles for chondroitin sulfate, contributes to early disk degeneration.33
LUMBAR SPONDYLOSIS
Osteoarthritis is the outcome of a disease process that results in structural and functional failure of synovial joints. This process has been characterized by damage to articular cartilage, subchondral bone alterations, synovial inflammatory response, and an overgrowth of bone and cartilage.34 In the spine, both the presence of intervertebral disk degeneration
and osteophyte formation at the same vertebral level has been used to describe lumbar spine osteoarthritis, also known as spondylosis. Similar to other synovial joints, degenerative changes of the facet joints occur in the form of degeneration of joint cartilage, remodeling of subchondral bones, osteosclerosis, and bone spur formation. These changes are similar to those in other load-bearing joints. Alignment abnormalities cause dynamic loads to be unbalanced on the facet joints.35
and osteophyte formation at the same vertebral level has been used to describe lumbar spine osteoarthritis, also known as spondylosis. Similar to other synovial joints, degenerative changes of the facet joints occur in the form of degeneration of joint cartilage, remodeling of subchondral bones, osteosclerosis, and bone spur formation. These changes are similar to those in other load-bearing joints. Alignment abnormalities cause dynamic loads to be unbalanced on the facet joints.35
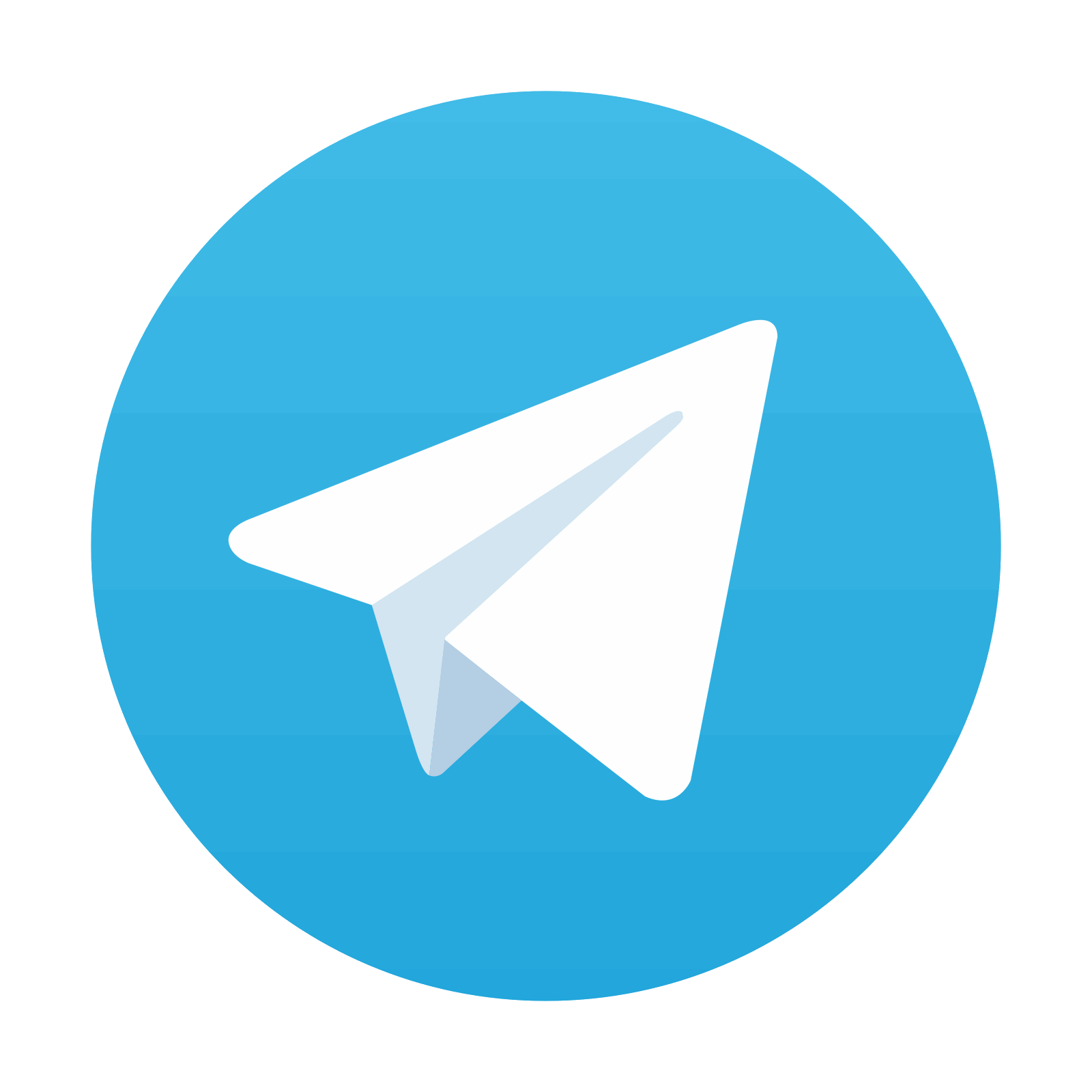
Stay updated, free articles. Join our Telegram channel

Full access? Get Clinical Tree
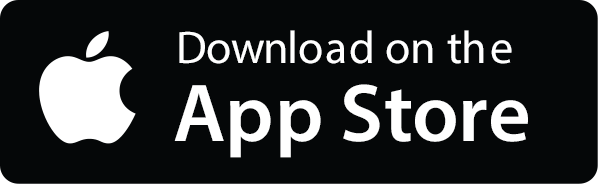
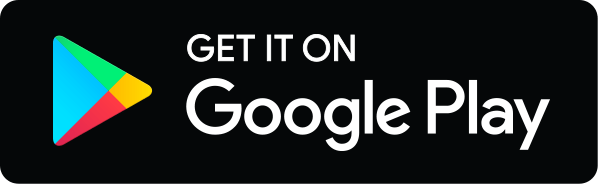