Vitamin D is obtained from cutaneous production when 7-dehydrocholesterol is converted to vitamin D 3 (cholecalciferol) by ultraviolet B radiation or by oral intake of vitamin D 2 (ergocalciferol) and D 3 . An individual’s vitamin D status is best evaluated by measuring the circulating 25-hydroxyvitamin D (25(OH)D) concentration. Although controversy surrounds the definition of low vitamin D status, there is increasing agreement that the optimal circulating 25(OH)D level should be approximately 30 to 32 ng/mL or above. Using this definition, it has been estimated that approximately three-quarters of all adults in the United States have low levels. Low vitamin D status classically has skeletal consequences such as osteomalacia/rickets. More recently, associations between low vitamin D status and increased risk for various nonskeletal morbidities have been recognized; whether all of these associations are causally related to low vitamin D status remains to be determined. To achieve optimal vitamin D status, daily intakes of at least 1000 IU or more of vitamin D are required. The risk of toxicity with “high” amounts of vitamin D intake is low. Substantial between-individual variability exists in response to the same administered vitamin D dose. When to monitor 25(OH)D levels has received little attention. Supplementation with vitamin D 3 may be preferable to vitamin D 2 .
Vitamin D is obtained either by ingestion or cutaneous production. When skin is exposed to ultraviolet B radiation, 7-dehydrocholesterol is converted to vitamin D 3 (cholecalciferol). Dietary sources may provide either vitamin D 3 or vitamin D 2 (ergocalciferol). However, few foods contain appreciable amounts of vitamin D, as such dietary intake is often low. Combining low intake with indoor lifestyle and sun-avoiding behaviors including sunscreen use, it is not surprising that low vitamin D status is endemic. The skeletal health consequences of vitamin D deficiency (calcium malabsorption and skeletal fragility) have long been recognized. More recently it has become appreciated that low vitamin D status leads to muscle weakness, falls, and potentially a multitude of nonskeletal morbidities. This review considers the definition and prevalence, potential health consequences, and approaches to correcting low vitamin D status.
Vitamin D background and assessment
Vitamin D must be metabolized to become physiologically active. Specifically, vitamin D (either D 2 or D 3 ) is converted to 25-hydroxyvitamin D (25(OH)D) in the liver and subsequently to the active or “hormonal” form, 1,25-dihydroxyvitamin D (1,25(OH) 2 D) in the kidneys. Measurement of 25(OH)D is the accepted indicator of an individual’s vitamin D status. This evaluation is not intuitive, as it would seem logical that measurement of the active form, 1,25(OH) 2 D, would be the appropriate measure of an individual’s vitamin D status. It is not rare anecdotally to see health care providers obtain measurement of 1,25(OH) 2 D purportedly to evaluate an individual patient’s vitamin D status. However, measurement of circulating 1,25(OH) 2 D does not provide a useful assessment of an individual’s vitamin D status, as vitamin D deficiency leads to parathyroid hormone (PTH) elevation, which enhances renal 1α-hydroxylase activity thereby promoting conversion of available 25(OH)D to 1,25(OH) 2 D. As 25(OH)D is present in much higher concentration than 1,25(OH) 2 D (ng/mL vs pg/mL) given the enhanced conversion induced by PTH elevation, 1,25(OH) 2 D may be normal even in the setting of low vitamin D status.
The clinical measurement of 25(OH)D has been problematic, with substantial variability present between laboratories. It is not the purpose of this review to detail approaches to and challenges with vitamin D measurement; this topic is reviewed elsewhere in this issue. Suffice it to say that current evaluations find that clinical 25(OH)D measurement has improved, allowing health care providers to have reasonable confidence in clinical 25(OH)D measurements. Moreover, the recent availability of standard reference materials from the National Institute of Standards and Technology seems destined to further improve between-laboratory agreement. However, despite 25(OH)D assay improvements, health care providers must appreciate that assay variability is present for all laboratory results. The analytical imprecision and inaccuracy present in all quantitative medical procedures is due to method, human, and instrument limitations that confound application of rigid diagnostic cutpoint approaches. For example, if one were using a 25(OH)D value of 30 ng/mL to differentiate “low” from “optimal” vitamin D status, it must be recognized that a laboratory result of 29 ng/mL does not differ from 31 ng/mL.
Low vitamin D status: definition and prevalence
A spectrum of vitamin D status has been proposed wherein individuals whose serum 25(OH)D value is less than approximately 10 ng/mL are classified as deficient and may sustain impaired bone mineralization (rickets/osteomalacia), while those with a value less than approximately 30 ng/mL are identified as insufficient ( Fig. 1 ) and may sustain long-term adverse health consequences. However, the cutpoint values selected, and even the verbiage to describe low vitamin D status, remain controversial. For example, terminology including deficiency, insufficiency, inadequacy, and hypovitaminosis has been variously, and interchangeably, applied to describe low vitamin D status. To avoid what seems to be a nonproductive debate, the terminology “low vitamin D status” is used here. Moreover, as noted above, 25(OH)D assay variability and absence of accepted standards has confounded agreement on a single definition of “low.”

Recognizing that controversy exists, there seems to be increasing consensus that circulating 25(OH)D values less than approximately 30 to 32 ng/mL indicate less than ideal vitamin D status. These cutpoint values were suggested based on the long-established role of vitamin D to facilitate calcium and phosphorus absorption with deficiency leading to rickets/osteomalacia. Thus, less severe vitamin D “deficiency” appears to cause calcium malabsorption, leading to secondary hyperparathyroidism with resulting elevated bone turnover and ultimately, bone loss. The 25(OH)D to PTH relationship has been extensively reported, with various studies finding an apparent inflection point at around 20 to 30 ng/mL. Moreover, some work demonstrates improved calcium absorption at 25(OH)D levels within what had previously been accepted as the “normal” range. However, others have challenged this seemingly cardinal tenant of low vitamin D status by reporting that calcium malabsorption does not occur until severe vitamin D deficiency is present, due to PTH-mediated maintenance of 1,25-dihydroxyvitamin D levels. Finally, it has recently been suggested by Heaney and colleagues that the point at which hepatic 25(OH)D production becomes zero order could be used to define the lower end of normal vitamin D status. In this work, the investigators found serum 25(OH)D to rapidly increase as circulating vitamin D 3 (cholecalciferol) increased. When circulating vitamin D 3 exceeds approximately 5.8 ng/mL, the hepatic 25-hydroxylase appears to become saturated and this reaction switches from first order to zero order. Taking this approach would define the lower limit of normal at approximately 35 ng/mL, obviously quite close to the 30 to 32 ng/mL suggested by other end points such as the relationship with PTH.
It is plausible that some of the debate surrounding what value defines “optimal” 25(OH)D status is being confounded by different levels for various tissues and end points; that is, the cutpoint for various nonclassic targets of vitamin D might vary from that for bone. Furthermore, it is possible that the 25(OH)D value for “optimal” physiologic functioning might differ between individuals. Having a range of “normal” for virtually all clinically measured biologic parameters is well known by clinicians. As vitamin D is, in essence, an endogenously produced hormone, it is not surprising that between-individual variability and regulation would exist. In this regard, the skin of humans, and other animals, possesses the ability to regulate cholecalciferol production. Moreover, limited data suggest that variation in vitamin D degradation may exist, in that differences in 24-hydroxylase capacity between individuals may be based on race. Data from a study of adults in Hawaii supports between-individual differences despite abundant sun exposure. In fact, inspection of serum 25(OH)D concentration in that cohort reveals a virtually normal or Gaussian distribution ( Fig. 2 ). Indeed, other studies of highly UV-exposed adults find some individuals with “low” 25(OH)D despite high UV exposure and a fairly broad range of what it would seem logical to define as “normal,” as noted in Fig. 2 . Thus, it seems likely that some of these people with “low” 25(OH)D levels could in fact be “optimal” for them. It is clearly accepted that a range exists for multiple physiologic functions that is considered “normal” for healthy adults; it is not known whether the same should apply to 25(OH)D.

Given these data, it is not surprising that an exact 25(OH)D cutpoint to define suboptimal vitamin D status remains somewhat controversial. Despite this, there is increasing agreement that values less than approximately 30 to 32 ng/mL be identified as “low.” When this cutpoint is applied, low vitamin D status is extremely common worldwide. For example, recent reports classify 52% to 77% of the studied cohorts as “low” using 30 ng/mL as a cutpoint. Even the more restrictive cutpoint of less than 20 ng/mL identifies 18% to 36% as “low” ( Fig. 3 ). It is not surprising that studies report a variable prevalence of low vitamin D status as the studied cohorts differ in age, sex, race, body mass index, and dietary vitamin D intake. Although it is often assumed that some of the variable prevalence of low vitamin D reflects limited availability of sun exposure due to living at higher latitudes, it is of interest that a recent meta-analysis involving 394 studies comprising more than 32,000 subjects found no influence of latitude on 25(OH)D concentration. It seems likely that the absence of an effect based on latitude reflects current human indoor lifestyles, clothing, and sun-avoidance behavior. Moreover, it is probable that these factors are contributing to worsening population vitamin D status. Although measurement issues confound data interpretation, recent National Health and Nutrition Examination Survey data report a decline in mean 25(OH)D concentration from the 1988 to 1994 data collection to that of 2000 to 2004. In summary, despite variation in 25(OH)D methodology, cutpoint selected, and cohort studied, it is clear that low vitamin D status is common worldwide.

Low vitamin D status: definition and prevalence
A spectrum of vitamin D status has been proposed wherein individuals whose serum 25(OH)D value is less than approximately 10 ng/mL are classified as deficient and may sustain impaired bone mineralization (rickets/osteomalacia), while those with a value less than approximately 30 ng/mL are identified as insufficient ( Fig. 1 ) and may sustain long-term adverse health consequences. However, the cutpoint values selected, and even the verbiage to describe low vitamin D status, remain controversial. For example, terminology including deficiency, insufficiency, inadequacy, and hypovitaminosis has been variously, and interchangeably, applied to describe low vitamin D status. To avoid what seems to be a nonproductive debate, the terminology “low vitamin D status” is used here. Moreover, as noted above, 25(OH)D assay variability and absence of accepted standards has confounded agreement on a single definition of “low.”
Recognizing that controversy exists, there seems to be increasing consensus that circulating 25(OH)D values less than approximately 30 to 32 ng/mL indicate less than ideal vitamin D status. These cutpoint values were suggested based on the long-established role of vitamin D to facilitate calcium and phosphorus absorption with deficiency leading to rickets/osteomalacia. Thus, less severe vitamin D “deficiency” appears to cause calcium malabsorption, leading to secondary hyperparathyroidism with resulting elevated bone turnover and ultimately, bone loss. The 25(OH)D to PTH relationship has been extensively reported, with various studies finding an apparent inflection point at around 20 to 30 ng/mL. Moreover, some work demonstrates improved calcium absorption at 25(OH)D levels within what had previously been accepted as the “normal” range. However, others have challenged this seemingly cardinal tenant of low vitamin D status by reporting that calcium malabsorption does not occur until severe vitamin D deficiency is present, due to PTH-mediated maintenance of 1,25-dihydroxyvitamin D levels. Finally, it has recently been suggested by Heaney and colleagues that the point at which hepatic 25(OH)D production becomes zero order could be used to define the lower end of normal vitamin D status. In this work, the investigators found serum 25(OH)D to rapidly increase as circulating vitamin D 3 (cholecalciferol) increased. When circulating vitamin D 3 exceeds approximately 5.8 ng/mL, the hepatic 25-hydroxylase appears to become saturated and this reaction switches from first order to zero order. Taking this approach would define the lower limit of normal at approximately 35 ng/mL, obviously quite close to the 30 to 32 ng/mL suggested by other end points such as the relationship with PTH.
It is plausible that some of the debate surrounding what value defines “optimal” 25(OH)D status is being confounded by different levels for various tissues and end points; that is, the cutpoint for various nonclassic targets of vitamin D might vary from that for bone. Furthermore, it is possible that the 25(OH)D value for “optimal” physiologic functioning might differ between individuals. Having a range of “normal” for virtually all clinically measured biologic parameters is well known by clinicians. As vitamin D is, in essence, an endogenously produced hormone, it is not surprising that between-individual variability and regulation would exist. In this regard, the skin of humans, and other animals, possesses the ability to regulate cholecalciferol production. Moreover, limited data suggest that variation in vitamin D degradation may exist, in that differences in 24-hydroxylase capacity between individuals may be based on race. Data from a study of adults in Hawaii supports between-individual differences despite abundant sun exposure. In fact, inspection of serum 25(OH)D concentration in that cohort reveals a virtually normal or Gaussian distribution ( Fig. 2 ). Indeed, other studies of highly UV-exposed adults find some individuals with “low” 25(OH)D despite high UV exposure and a fairly broad range of what it would seem logical to define as “normal,” as noted in Fig. 2 . Thus, it seems likely that some of these people with “low” 25(OH)D levels could in fact be “optimal” for them. It is clearly accepted that a range exists for multiple physiologic functions that is considered “normal” for healthy adults; it is not known whether the same should apply to 25(OH)D.
Given these data, it is not surprising that an exact 25(OH)D cutpoint to define suboptimal vitamin D status remains somewhat controversial. Despite this, there is increasing agreement that values less than approximately 30 to 32 ng/mL be identified as “low.” When this cutpoint is applied, low vitamin D status is extremely common worldwide. For example, recent reports classify 52% to 77% of the studied cohorts as “low” using 30 ng/mL as a cutpoint. Even the more restrictive cutpoint of less than 20 ng/mL identifies 18% to 36% as “low” ( Fig. 3 ). It is not surprising that studies report a variable prevalence of low vitamin D status as the studied cohorts differ in age, sex, race, body mass index, and dietary vitamin D intake. Although it is often assumed that some of the variable prevalence of low vitamin D reflects limited availability of sun exposure due to living at higher latitudes, it is of interest that a recent meta-analysis involving 394 studies comprising more than 32,000 subjects found no influence of latitude on 25(OH)D concentration. It seems likely that the absence of an effect based on latitude reflects current human indoor lifestyles, clothing, and sun-avoidance behavior. Moreover, it is probable that these factors are contributing to worsening population vitamin D status. Although measurement issues confound data interpretation, recent National Health and Nutrition Examination Survey data report a decline in mean 25(OH)D concentration from the 1988 to 1994 data collection to that of 2000 to 2004. In summary, despite variation in 25(OH)D methodology, cutpoint selected, and cohort studied, it is clear that low vitamin D status is common worldwide.
Low vitamin D status: consequences
Bone
Low vitamin D status has long been associated with osteomalacia/rickets, and a role in osteoporosis pathogenesis via calcium malabsorption and secondary hyperparathyroidism has more recently been suggested. Consistent with an important role of low vitamin D status in osteoporosis, recent meta-analyses find low 25(OH)D to be associated with higher fracture risk. In addition, a dose effect was reported, with greater vitamin D intakes and higher achieved 25(OH)D concentrations providing superior fracture reduction benefit. In summary, while one can debate the cutpoint, low vitamin D status leads to adverse bone consequences.
Muscle Function and Falls
Both genomic and nongenomic effects of vitamin D on muscle have been proposed. Regardless of the mechanism, patients with osteomalacia due to vitamin D deficiency develop muscle pain and weakness that is improved with vitamin D therapy. Muscle biopsy in such people reveals atrophy of the fast twitch (type II) fibers. As type II fibers are first to be recruited to avoid falling, this observation may explain the increased falls risk in vitamin D deficient individuals. Of note, randomized prospective studies find vitamin D to reduce risk of falls by more than 20%. It seems likely that reducing falls contributes in a major way to the fracture reduction efficacy observed with vitamin D. Moreover, similar to the relationship observed with fracture, a higher vitamin D dose provides greater reduction in the risk of falls. The 25(OH)D concentration needed to optimize leg function has been explored, with various cutpoints (eg, 16–24 ng/mL) suggested. A recent review finds 25(OH)D concentrations less than approximately 16 ng/mL to be associated with substantially poorer leg function, but additionally finds values greater than approximately 36 to 40 ng/mL to be optimal.
Cancer
Vitamin D has antiproliferative and prodifferentiating effects on many cell types. It has been proposed that these effects are related to local production of 1,25-dihydroxyvitamin D, thus favorably impacting genes affecting cellular proliferation/differentiation and thereby reducing cancer risk. Consistent with this, an extensive, albeit largely associational, literature exists relating higher latitude, low vitamin D intake, and/or less sunlight exposure to increased risk of, or mortality from, multiple types of cancer. Prospective trials of vitamin D supplementation with cancer as an end point are very limited; the Women’s Health Initiative did not demonstrate a reduction in colon cancer risk, perhaps related to the low daily dose (400 IU) of vitamin D used. However, a smaller prospective study of postmenopausal women found calcium plus vitamin D 3 (1100 IU daily) to reduce overall cancer risk by approximately 60%. To summarize, physiologically logical hypotheses, observational data, and one small randomized trial find low vitamin D status to be associated with higher cancer risk. Additional prospective studies are needed.
Other Conditions
It is likely that vitamin D has immune modulating effects. It has long been recognized that vitamin D deficiency is associated with respiratory infections, which perhaps contributed to the use of cod liver oil in antituberculous therapy. More recently, it has become appreciated that calcitriol enhances monocyte mycobacterial killing, likely by facilitating production of the antimicrobial protein, cathelicidin. Moreover, helper type 1 and 2 cells are vitamin D targets, with vitamin D causing a shift toward an anti-inflammatory profile. Thus, it is not surprising that low vitamin D status is associated with an increased risk of autoimmune and potentially infectious diseases. In addition, inflammation is increasingly being recognized as a contributor to the pathogenesis of various diseases, and vitamin D modulates inflammatory cytokine production.
It has been suggested that endemic low vitamin D status is contributing to the increased prevalence of diabetes mellitus. Multiple potential mechanisms have been proposed, including vitamin D increasing insulin production/secretion. In addition, observational studies associate low vitamin D status with diabetes type 1 and type 2. Prospective studies of vitamin D supplementation are clearly indicated; however, on the whole it appears that low vitamin D status impairs glucose metabolism.
Observational studies report an association between low vitamin D status and cardiovascular disease. Potential mechanisms include a vitamin D effect on the endothelium, vascular smooth muscle, and/or cardiomyocytes, all of which possess the vitamin D receptor. Prospective studies to further evaluate this reported association are needed.
In summary, low vitamin D status has been associated with a variety of diseases, and biologically plausible hypotheses exist to suggest a possible causal role. However, until confirmed by randomized studies, it is wise to be cautious and recognize that association does not prove causation.
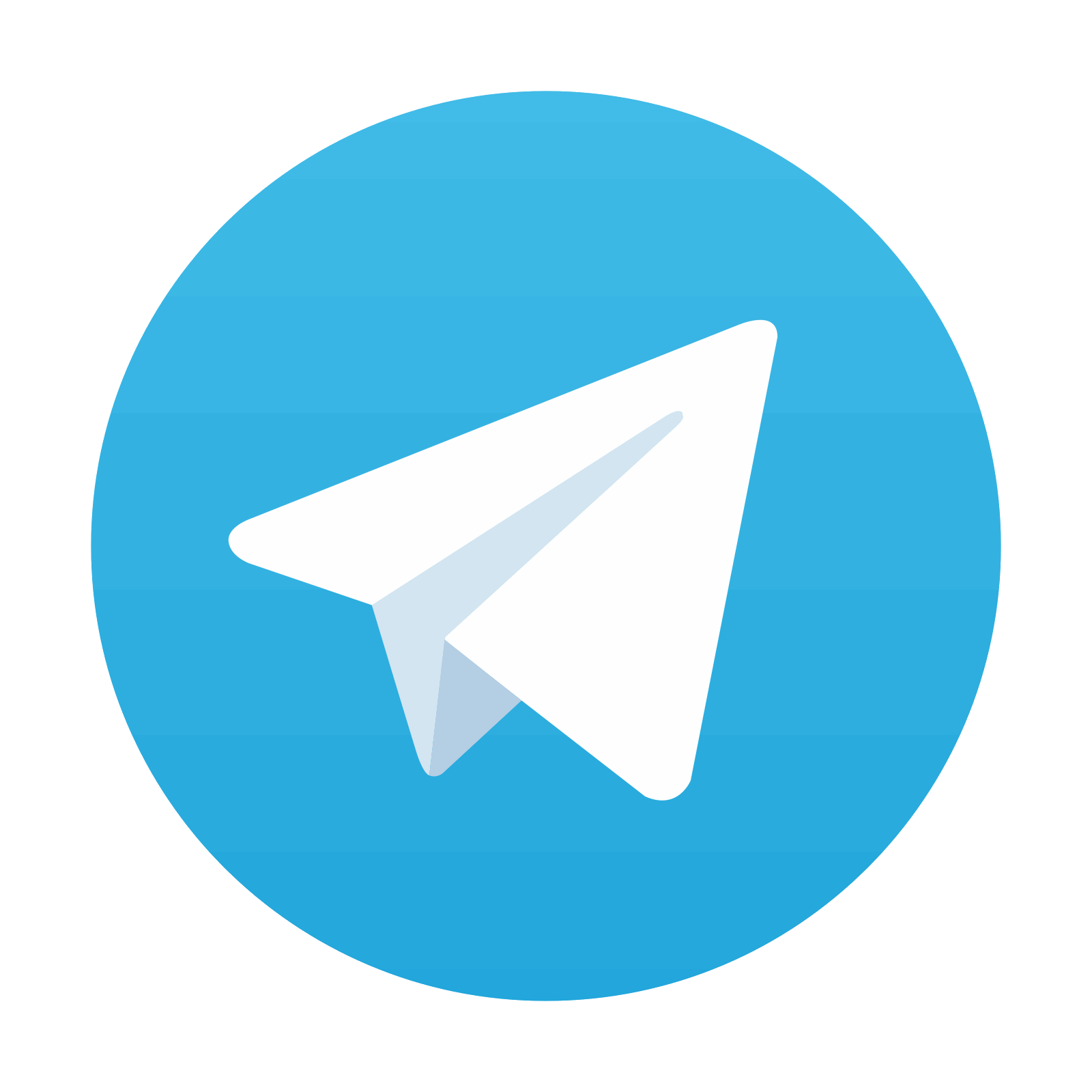
Stay updated, free articles. Join our Telegram channel

Full access? Get Clinical Tree
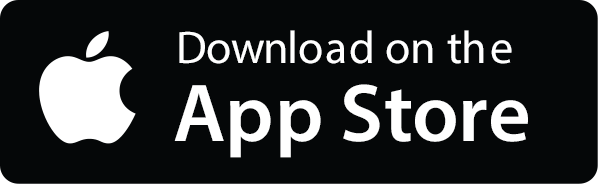
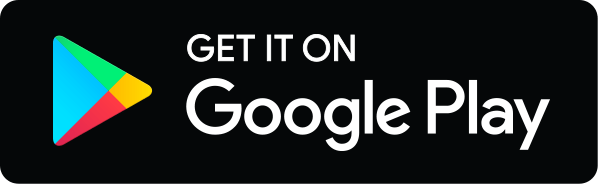