Introduction
This study aimed to assess the transfer accuracy of a digital indirect bonding method for lingual brackets using double vacuum-formed trays in vivo.
Methods
Twenty-five patients in need of lingual orthodontic treatment were consecutively recruited. Bracket placement was performed on ideal setups, followed by fabricating indirect bonding trays through vacuum-forming on 3-dimensional printed models. Transfer accuracy was measured at each bracket after superimpositions of postbonding scans and reference data. One-tailed t tests were used to determine whether bracket deviations were within the limit of 0.5 mm and 2° for linear and angular dimensions, respectively.
Results
A total of 611 lingual brackets were evaluated. Mean linear transfer errors were 0.06 mm, 0.09 mm, and 0.12 mm, with frequencies of deviations within the 0.5 mm limit of 99.7%, 99.8%, and 98.0% for mesiodistal, buccolingual, and occlusogingival dimensions, respectively. Regarding angular measurements, mean transfer errors were 1.28°, 1.73°, and 2.96°, with frequencies of deviations within the 2° limit of 81.0%, 68.9%, and 51.1% for rotation, tip, and torque, respectively. Mean errors fell within the clinically accepted limits for all linear dimensions and rotation but exceeded the limit for tip and torque.
Conclusions
Lingual bracket indirect bonding using double vacuum-formed trays fabricated on 3-dimensional printed models has high transfer accuracy in the mesiodistal, buccolingual, and occlusogingival dimensions and rotation. However, the transfer of tip and torque is less accurate.
Highlights
- •
Transfer accuracy of lingual brackets using vacuum-formed trays was comparable to labial ones.
- •
Lingual brackets were transferred with high accuracy in all linear dimensions and rotations.
- •
Transfer accuracy of tip and torque remained questionable.
- •
Failure bracket could be rebonded with equivalent accuracy using a nonsegmented original tray.
Since its first introduction in 1979, lingual orthodontics treatment has become a popular esthetic orthodontic treatment alternative for highly esthetic-demanding patients. Because of the complex and variable anatomic morphology of the tooth lingual surface; lingual brackets are typically indirectly bonded after precise placement on an orthodontic setup with ideal tooth alignment. , When analog orthodontic setups are used, lingual brackets can be transferred to the patient’s mouth using individual tooth transfer jigs. , Alternatively, silicone or vacuum-formed trays can simultaneously transfer a group of teeth for digital setups. This is because of the digital method’s ability to virtually reposition the brackets and teeth together back to the initial malocclusion state.
The transfer accuracy of indirect bonding trays has an important effect on the lingual orthodontic treatment result to ensure that the final tooth alignment matches the ideal orthodontic setup. Although several articles evaluate bracket transfer accuracy in the literature, most focus on labial brackets and include both in vitro studies and clinical studies. These studies evaluated various tray types, including double vacuum-formed, silicone, and 3-dimensional (3D) printed trays. They generally find high accuracy in transferring linear dimensions, whereas the accuracy of angular dimensions varies among studies, with torque being the least accurately transferred.
In contrast, limited clinical study is available on the accuracy of lingual indirect bonding with the existing study using individual tooth transfer jigs created through an analog process with plaster setup models. The narrower interbracket span and the suboptimal adaptation between bracket bases and lingual tooth surfaces because of anatomic variability may influence the transfer accuracy of lingual bracket transfer trays. Therefore, this study aimed to assess the transfer accuracy of a digital indirect bonding method for lingual brackets using double vacuum-formed trays in vivo. The null hypothesis proposed that bracket deviation values were noninferior to the clinically accepted thresholds of 0.5 mm and 2°.
Material and methods
This clinical study’s protocol was approved by the Hanoi Medical University Institutional Ethical Review Board (approval no. 970). All participants provided written informed consent. The subjects were 25 consecutive patients, including 22 females and 3 males, aged 18-37 years at the beginning of treatment. Thirteen patients underwent premolar extractions, whereas 12 were treated with a nonextraction approach. The prevalence of Class I, II, and III occlusions was 0.72, 0.08, and 0.2, respectively. Most patients presented with mild crowding in both the maxillary (80%) and mandibular (72%) arches, followed by moderate crowding in the maxillary (16%) and mandibular (24%) arches, and severe crowding (4%). The required sample size was estimated based on the effect size of 0.858, calculated from the mean and standard deviation of tip errors of canine brackets, which had the greatest errors in a previous study on double vacuum-formed trays. The result suggested that analyzing 17 brackets of each tooth type (a total of 136 brackets for 8 tooth types) would provide 95% power to identify means to be statistically significantly different from 0.5 mm or 2° through 1-sample t tests at a significance level of 0.05.
The double vacuum-formed indirect bonding tray was designed and fabricated following the protocol described in a case report. Digital diagnostic models were taken using an i500 intraoral scanner (Medit, Seoul, Korea) and imported to Autolign software (Diorco, Gyeonggi-do, Korea). The teeth were segmented, and virtual setups were created according to the treatment plans. Next, virtual lingual brackets were placed on the setup models on the basis of a straight lingual archform, and the tooth-bracket combinations were virtually repositioned back to the initial malocclusion state.
The initial models with ideal bracket positions were exported as standard tessellation language (STL) files to be 3D printed with a Sonic Mighty printer (Phrozen, Hsinchu, Taiwan) using Study Model White 2 resin (Sprintray, Huntington Beach, Calif). These STL files were used as references to compare scanned data after clinical bracket bonding. The indirect bonding trays were vacuum-formed on the printed models using Bioplast foils (Scheu Dental, Iserlohn, Germany) for the inner soft layers and Biocryl foils (Scheu Dental) for the outer hard layers. Each tray was sectioned into 3 parts, including 1 anterior and 2 posterior segments, and ADB lingual brackets (Medico, Gyeonggi-do, Korea) were placed into the trays ( Fig 1 ).

In the clinical bonding procedure, the lingual tooth surfaces were thoroughly cleaned, etched with 37% phosphoric acid for 20 seconds, and dried. Assure Plus primer and GoTo adhesive (Reliance Orthodontic Products, Itasca, Ill) were applied and light-cured for 40 seconds. Next, the outer layers of the trays were carefully removed, followed by the removal of the inner layers so as not to debond the brackets. Finally, the entire arches were scanned with the same intraoral scanner and exported as STL files serving as targets for comparison with ideal bracket positions.
The method for measuring the transfer accuracy of indirect lingual bracket bonding was similar to that used in another study. First, the computer-aided design patch of each bracket that the manufacturer provided was set at the origin of a coordinate system in which the x-axis was parallel to the mesiodistal edge of the main slot, the z-axis was parallel to the buccolingual edge of the main slot, and the y axis was perpendicular to the xz plane ( Fig 2 ). The bracket base was removed from the bracket patch as only the bracket body would determine the future tooth movement. Next, the reference and target data were superimposed to the bracket patch with local best-fit alignment using Medit Design software (Medit, Seoul, Korea). The target data was then combined with the bracket patch, followed by a local best-fit alignment of the combined data to the reference data in which only the tooth surface of the measured tooth and 2 adjacent teeth were included in the alignment. The coordinate of the new position of the bracket patch would be the deviation between the target data and the reference data (N.V.A.).

For each bracket inspection, the deviation described bracket discrepancies in 6 dimensions, including 3 linear translations (mesiodistal, buccolingual, and occlusogingival) and 3 angular dimensions (rotation, tip, and torque). Absolute values were calculated for each deviation to ensure that positive and negative deviation values would not negate each other. To control for measurement reliability, the same operator (N.V.A.) and another operator (N.M.D.) remeasured 56 brackets after a wash-out period of 10 days. Intraobserver and interobserver reliability was analyzed with the intraclass correlation coefficient (ICC).
Statistical analysis
All data analyses were performed with SPSS software (version 23.0; IBM, Armonk, NY). First, the normal distribution of the data was confirmed with the Shapiro-Wilk test. Next, mean values and standard deviations for each dimension were calculated for the total sample and each tooth type, including maxillary and mandibular incisors, canines, premolars, and molars. The bracket deviation values were compared between right and left teeth using 2-sample t tests, demonstrating no significant difference. Therefore, corresponding teeth were combined on the right and left sides for subsequent analysis.
One-sample t tests were used to determine whether the bracket deviation values were within the limits of 0.5 mm for linear and 2° for angular measurements with the level of statistical significance set to α = 0.05. These limits were selected from the accepted professional standards of the American Board of Orthodontics objective grading system. , , , The frequencies of the bracket deviation values within and outside these limits were also computed in each dimension, considering each tooth type and the whole sample.
Results
A single orthodontist (N.V.A.) bonded 611 lingual brackets, with 6 bonding failures during tray removal, resulting in a failure rate of 0.98%. The mean bonding time of the maxillary, mandibular, and 2 arches was 19.6, 22.7, and 36.5 minutes, respectively. Mandibular arch bonding took significantly longer than maxillary arch bonding ( P <0.05). Thirty-four brackets were not bonded at the start of treatment because of severe crowding or poor fitting of brackets within indirect bonding trays.
The intraobserver and interobserver reliability was excellent, with ICCs of 0.944 and 0.929 for linear deviation and ICCs of 0.977 and 0.953 for angular deviation, indicating high reliability of the best-fit alignment and measurement procedures.
The means and standard deviation of discrepancies between the final bracket positions and the intended digital setups are shown in Table I and Figure 3 . For the whole sample, the linear transfer errors were 0.06 ± 0.07 mm, 0.09 ± 0.09 mm, and 0.12 ± 0.12 mm for the mesiodistal, buccolingual, and occlusogingival dimensions, respectively. Regarding angular measurements, the transfer errors for the whole sample were 1.28 ± 1.18° for rotation, 1.73 ± 1.78° for tip, and 2.96 ± 3.03° for torque.
Tooth type | n | Linear dimension | Angular dimension | ||||
---|---|---|---|---|---|---|---|
MD (mm) | BL (mm) | OG (mm) | Rotation (°) | Tip (°) | Torque (°) | ||
Incisor | 184 | 0.052 ± 0.085 ∗ | 0.073 ± 0.114 ∗ | 0.080 ± 0.084 ∗ | 1.122 ± 1.052 ∗ | 1.588 ± 1.708 ∗ | 1.762 ± 1.604 ∗ |
Maxillary | 97 | 0.048 ± 0.080 ∗ | 0.071 ± 0.144 ∗ | 0.082 ± 0.092 ∗ | 1.203 ± 1.122 ∗ | 1.788 ± 1.931 | 1.848 ± 1.725 |
Mandibular | 87 | 0.057 ± 0.091 ∗ | 0.074 ± 0.069 ∗ | 0.077 ± 0.075 ∗ | 1.031 ± 0.967 ∗ | 1.365 ± 1.395 ∗ | 1.666 ± 1.462 ∗ |
Canine | 88 | 0.073 ± 0.066 ∗ | 0.066 ± 0.051 ∗ | 0.077 ± 0.061 ∗ | 1.489 ± 1.298 ∗ | 2.500 ± 2.734 | 2.387 ± 2.307 |
Maxillary | 47 | 0.075 ± 0.073 ∗ | 0.073 ± 0.056 ∗ | 0.078 ± 0.068 ∗ | 1.463 ± 1.302 ∗ | 2.547 ± 3.314 | 2.721 ± 2.657 |
Mandibular | 41 | 0.071 ± 0.057 ∗ | 0.057 ± 0.044 ∗ | 0.075 ± 0.053 ∗ | 1.519 ± 1.309 ∗ | 2.445 ± 1.905 | 2.003 ± 1.781 |
Premolar | 144 | 0.061 ± 0.050 ∗ | 0.106 ± 0.083 ∗ | 0.190 ± 0.154 ∗ | 1.447 ± 1.334 ∗ | 2.032 ± 1.736 | 4.047 ± 3.773 |
Maxillary | 74 | 0.061 ± 0.051 ∗ | 0.099 ± 0.070 ∗ | 0.197 ± 0.173 ∗ | 1.346 ± 1.180 ∗ | 2.094 ± 1.891 | 3.397 ± 3.178 |
Mandibular | 70 | 0.061 ± 0.049 ∗ | 0.113 ± 0.095 ∗ | 0.182 ± 0.132 ∗ | 1.553 ± 1.480 ∗ | 1.967 ± 1.567 | 4.735 ± 4.228 |
Molar | 195 | 0.057 ± 0.054 ∗ | 0.095 ± 0.082 ∗ | 0.130 ± 0.126 ∗ | 1.198 ± 1.086 ∗ | 1.306 ± 1.054 ∗ | 3.540 ± 3.281 |
Maxillary | 99 | 0.055 ± 0.042 ∗ | 0.074 ± 0.063 ∗ | 0.118 ± 0.106 ∗ | 1.273 ± 1.207 ∗ | 1.400 ± 1.184 ∗ | 3.139 ± 2.833 |
Mandibular | 96 | 0.059 ± 0.064 ∗ | 0.116 ± 0.093 ∗ | 0.142 ± 0.144 ∗ | 1.121 ± 0.945 ∗ | 1.209 ± 0.898 ∗ | 3.952 ± 3.657 |
Total | 611 | 0.059 ± 0.066 ∗ | 0.087 ± 0.091 ∗ | 0.121 ± 0.124 ∗ | 1.276 ± 1.178 ∗ | 1.734 ± 1.781 | 2.958 ± 3.026 |
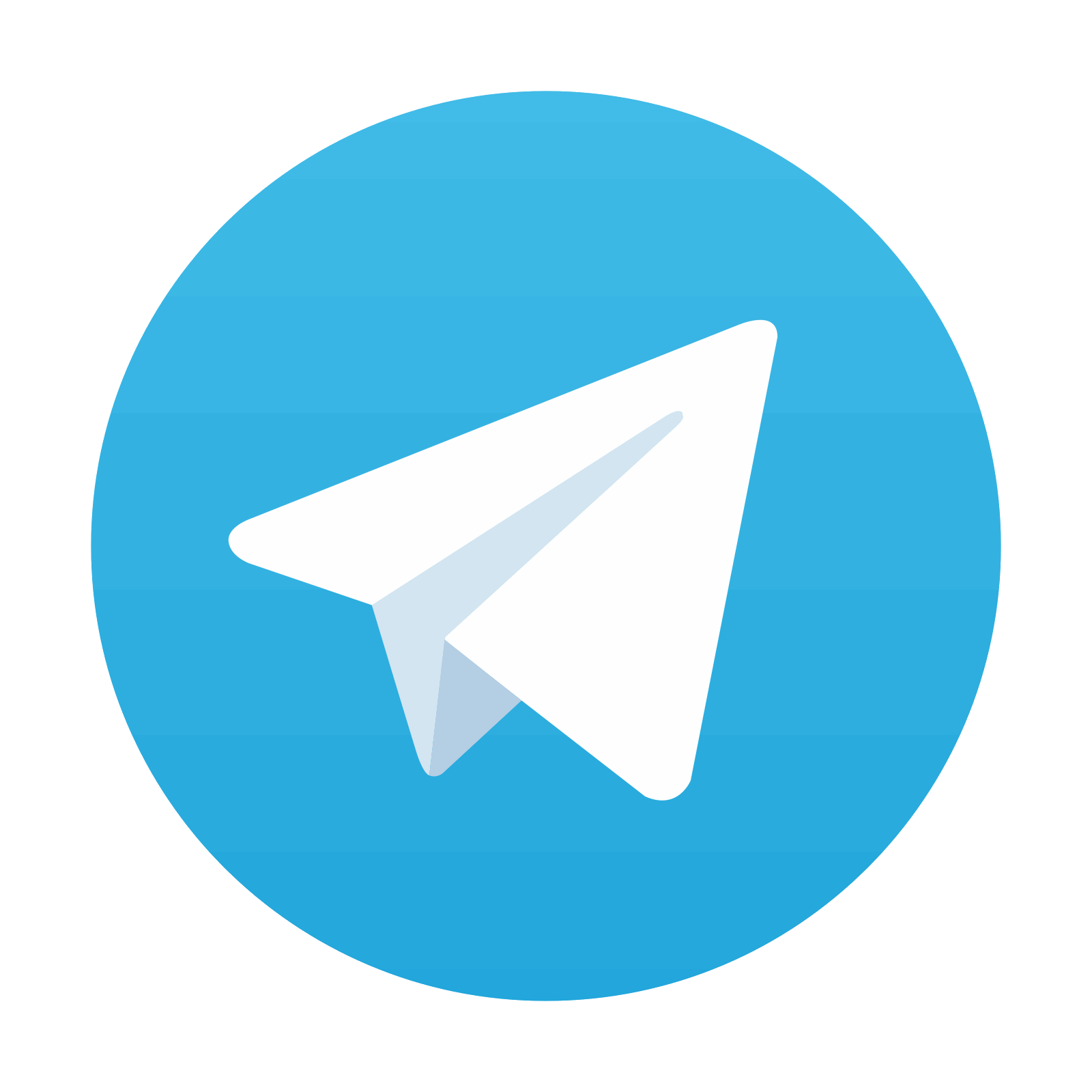
Stay updated, free articles. Join our Telegram channel

Full access? Get Clinical Tree
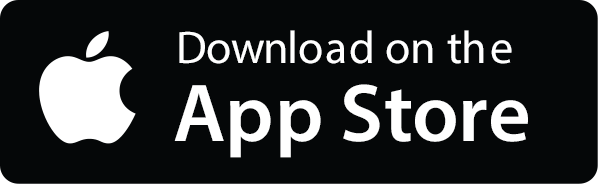
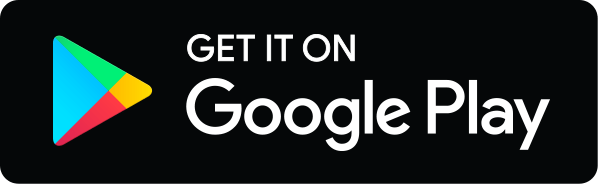
