Definition
Legg-Calvé-Perthes disease (LCPD) is a condition in which the blood supply of the capital femoral epiphysis (head) is disrupted, resulting in epiphyseal osteonecrosis and chondronecrosis with cessation of growth of the ossific nucleus. The necrotic epiphyseal bone is eventually resorbed and replaced by new bone. During the resorptive phase of the healing, the mechanical properties of the femoral head are weakened such that some femoral heads develop a flattened deformity (coxa plana). Over time, the femoral head reossifies, resumes growth with some overgrowing (coxa magna), and remodels to a variable degree of roundness until skeletal maturity.
Incidence
The annual incidence of Perthes disease is variable from one region to another, and regions at higher latitude are reported to have a higher incidence. The lowest incidences have been reported from east Asian countries, with a notable exception of a high incidence in the western coastal region of South India (14.4 per 100,000). In North America, an incidence of 5.1 per 100,000 was reported in British Columbia and 5.7 per 100,000 in Massachusetts. In the United Kingdom, the reported incidences have ranged from 7.9 per 100,000 in Trent, England, to 21.1 per 100,000 in Liverpool. In comparison with these data from 1983, subsequent studies have shown a large decline of incidence in Liverpool. A more recent study comparing the incidence of Perthes disease in the United Kingdom over a 19-year period showed an overall decline in incidence, especially in northern regions. This decline was thought to be linked to improved socioeconomic factors. In light of the data showing changes in incidence over time, a comparison of incidence from one region to another requires caution because some studies were conducted in the 1970s and 1980s. The older studies may or may not truly reflect the current incidence of the disease in the region. In other European countries, incidences of 9.2 per 100,000 in Norway and 8.5 per 100,000 in Sweden have been reported.
Perthes disease can affect children younger than 15 years old, but it is most commonly seen in children between the ages of 4 and 8 years. The male-to-female ratio was found to be 3.3 : 1 in Norway, 5 : 1 in Massachusetts, and 5.2 : 1 in British Columbia.
The disease is of variable severity, and bilateral involvement occurs in approximately 10% to 13% of patients ( Box 17-1 ). The disorder is most prevalent in children 4 to 8 years of age, but it can be seen in children from 18 months of age to skeletal maturity. It is more common in boys than in girls by a ratio of 4 : 1 or 5 : 1.
- •
Onset: between 18 months of age and skeletal maturity (most prevalent between 4 and 8 years of age)
- •
Male sex prevalence: four or five times more likely to develop in boys than in girls
- •
Involvement: bilateral in 10% to 12% of patients
- •
Symptoms: limp exacerbated by activity and alleviated with rest; pain, which may be located in the groin, proximal thigh, knee, or laterally around the greater trochanter; possible history of antecedent trauma
- •
Signs: abductor limp; decreased range of motion of the hip, especially on abduction and internal rotation (decreased range of motion transient early in the disease, persistent later on); flexion and extension less affected
History
LCPD was independently recognized as a distinct entity in 1910 by Arthur Legg of the United States, Jacques Calvé of France, Georg Perthes of Germany, and Henning Waldenström of Sweden ( Fig. 17-1 ).

Legg described the prominent characteristics of the disorder: onset between 5 and 8 years of age, a history of trauma, a painless limp, and minimal or no spasm or shortening of the affected limb. Calvé noted that affected individuals had minimal atrophy of the leg and no palpable hip swelling. He thought that the condition was a result of abnormal or delayed bone formation. Perthes observed the disorder as “a self-limiting, noninflammatory condition, affecting the capital femoral epiphysis with stages of degeneration and regeneration, leading to restoration of the bone nucleus.” Waldenström reported the radiographic changes associated with the disorder in 1909; however, in this early work, he thought the disease was a form of tuberculosis and not a distinct entity.
Etiology
The origin of LCPD is probably multifactorial, and the exact cause is unknown ( Box 17-2 ). It is also possible that LCPD has multiple causes that manifest in a common final pathway of avascular necrosis (AVN). A few studies have found a type II collagen alpha 1 chain ( COL2A1 ) mutation in a small number of families with inherited bilateral femoral head osteonecrosis whose children manifest Perthes-like radiographic changes. COL2A1 mutation has not been reported in sporadic unilateral or nonfamilial bilateral cases so far. Furthermore other COL2A1 mutations with a Perthes-like presentation and dysplastic skeletal features have also been reported. Whether these cases represent a subtle form of skeletal dysplasia rather than true Perthes disease is uncertain.
Some studies suggested that inherited coagulopathies (protein C or S deficiency, factor V Leiden mutation, and hypofibrinolysis) may be the primary cause of most cases of LCPD. Subsequent studies, however, have not corroborated these findings.
Many studies have proposed other etiologic factors. Because the pathologic changes seen in the femoral head are likely the result of an ischemic process, various investigators have implicated a disruption of both the arterial and venous systems. * Other theories of the cause of the disorder include the following: the “predisposed child” concept, based on findings of abnormal growth and development † ; trauma, particularly in the predisposed child ; hyperactivity or attention deficit disorder ; hereditary influences ; environmental factors, including exposure to cigarette smoke ; and the disease as a sequela of synovitis. ‡
* References .
† References .
‡ References .
Coagulation Abnormalities
Several hematologic abnormalities have been implicated in LCPD. Children with hemoglobinopathies such as sickle cell disease and thalassemia commonly have AVN of the femoral head. Avascular changes have also been reported in patients with chronic myeloid leukemia, non-Hodgkin lymphoma, idiopathic thrombocytopenic purpura, and hemophilia. An increase in blood viscosity in patients with LCPD has also been reported.
In 1984 Glueck and associates reported abnormal venous thrombus formation in 5 of 8 patients with LCPD. Deficiencies of proteins C and S and the presence of hypofibrinolysis were observed in these patients. In a second study comprising 44 children with LCPD, 23 had thrombophilia, 3 had hypofibrinolysis, and 7 had elevated lipoprotein(a). Of the 23 patients with thrombophilia, 19 had protein C deficiency and 4 had protein S deficiency. In the general population, a deficiency in protein C or S occurs in only 1 in 15,000 individuals. These findings were further corroborated in a subsequent study by Glueck and associates in which 50 of 64 children with LCPD had coagulation abnormalities. Resistance to activated protein C was the most common thrombophilic trait; 23 children had a low activated protein C ratio compared with 7 of 160 pediatric control patients. It was postulated that thrombophilia may cause venous thrombosis in the femoral head, with venous hypertension and hypoxic bone death resulting in LCPD.
Eldridge and co-workers studied 57 patients and found an increased risk of LCPD with decreasing levels of protein C and a nearly significant risk with decreasing levels of protein S. Beaule and others reported a case of protein C deficiency in a patient with LCPD and recurrent deep vein thrombosis. Szepesi and colleagues studied 47 patients with LCPD and found 5 with the factor V Leiden mutation. The 4 patients with a homozygous pattern for factor V had the most severe form of the disease. The most recent consecutive series from Glueck’s group found higher prevalences of factor V Leiden mutation (8 of 72 in the study group compared with 7 of 197 in the control group) and anticardiolipin antibodies (19 of 72 in the study group compared with 22 of 197 in the control group) in the patients with LCPD. The study, however, did not find an increase in the prevalence of protein C, protein S, and antithrombin III deficiencies. A larger case-control study from the Netherlands also found a higher prevalence of the factor V Leiden mutation (16 of 166 in the study group compared with 16 of 509 in the control group) and a higher prevalence of the prothrombin G20210A mutation (9 of 169 patients in the study group compared with 11 of 512 in the control group.
Some other studies have not found that coagulation abnormalities contribute to the origin of LCPD. Hresko and others prospectively studied 50 consecutive patients with LCPD for factor V Leiden mutation and levels of protein C, protein S, antithrombin III, and lipoprotein(a). The only abnormality found was an elevated level of lipoprotein(a) in 16% of the study group. A more recent case-control study consisting of 119 patients with LCPD and 276 control subjects from Israel also did not find a higher prevalence of the factor V Leiden mutation in the patients with LCPD (7 of 119 compared with 13 of 276 in the control group). Kealey and co-workers reviewed 139 children with LCPD and found no deficiencies of protein C, protein S, or antithrombin III and no resistance to activated protein C. These investigators did find 53 patients (38%) with prolonged activated partial thromboplastin times but did not speculate about the relationship of the finding with LCPD. Koo and associates found no coagulation abnormalities in 26 patients with Legg-Perthes disease.
McDougall and associates conducted coagulation studies on 49 patients with LCPD and did not find frequent abnormalities in protein C or S or lipoprotein(a). The incidence of heterozygotes with factor V Leiden (4%) was within the expected normal range. Gallistl and associates reported that of 44 patients with LCPD, only 1 (2.2%) had a deficiency in protein C activity and only 3 (6.8%) were positive for activated protein C resistance. These prevalences are slightly higher than the expected normal prevalences but significantly lower than the prevalences reported by Glueck and associates. In a study of 61 children with LCPD and 296 control subjects, the only inherited risk factor found by Arruda and associates was mutation in the factor V gene (factor V Leiden). The prevalence of factor V Leiden mutation was 4.9% in patients with LCPD, compared with 0.7% in control subjects. No patient had a prothrombin gene variant. It is unclear why the results of the foregoing studies vary so greatly. Possible reasons for the discrepancies in some of the studies may be the use of small sample sizes, selective patient population, retrospective study design, use of suboptimal controls, and a nonstandardized range of laboratory values for an abnormal coagulation factor level in different age groups.
Further confusion on the pathogenetic role of coagulation abnormities on the development of LCPD comes from a few studies of families with multigenerational transmission of a factor V Leiden mutation. In one family, only 1 out of the 11 members found to be heterozygous for the factor V Leiden mutation (G1691A) presented with LCPD. In another family with 10 members having either heterozygous or homozygous factor V mutation, 3 members had LCPD. Balasa and associates estimated that only 1 of 2777 children (0.036%) with the factor V Leiden mutation develops LCPD. Given that thrombotic events are uncommon during childhood, even in those patients with inherited thrombophilia, the etiologic significance of thrombophilia in LCPD remains unclear. At present, with so many conflicting data, we can conclude only that coagulation abnormalities may have limited etiologic role in the disorder and that future research is needed.
Arterial Status of the Femoral Head
Some evidence based on angiographic and bone scintigraphic studies suggests that the arterial circulation of the femoral head may be the primary site affected in LCPD. Normally, the primary blood supply to the capital epiphysis comes from two anastomotic arterial rings located around the neck of the femur ( Fig. 17-2 ). The medial and lateral femoral circumflex arteries form the extracapsular ring, and most of the blood is supplied by the medial circumflex artery. The major arterial supply to the femoral head is the lateral segment of the arterial ring, which is the terminal branch of the medial femoral circumflex artery. After penetrating the lateral capsule in the posterior trochanteric fossa, this blood vessel becomes the lateral ascending cervical artery. As the vessel advances between the trochanter and the capsule, it courses through a narrow passage, an area particularly constricted in children younger than 8 years of age. In addition to the extracapsular ring, a subsynovial intracapsular ring joins four ascending cervical arterial groups. This intracapsular ring has been found to be incomplete more often in boys than in girls. In most cases, no vessels cross the epiphyseal plate, and minimal blood is supplied through the ligamentum teres.

Angiographic studies have helped demonstrate the disruption of the blood supply to the proximal femur in patients with LCPD. Obstruction of the superior capsular arteries of the femoral head was clearly demonstrated in 11 patients during the first 5 months after onset of symptoms. As the disease process progressed, angiography revealed revascularization. In a single case, blood flow decreased when the hip was extended and improved when the hip was flexed to 30 degrees. A general reduction in blood flow, with a significant decrease in medial circumflex artery flow, has also been observed ( Fig. 17-3 ). Atsumi and co-workers performed angiographic studies and showed interruption of the lateral epiphyseal arteries just as they entered the hip capsule in 19 of 28 hips affected with LCPD. These investigators showed that revascularization was occurring at the same site in most cases. They also found that the arterial flow could be occluded by abduction and internal rotation of the hip. The major source of blood flow to the femoral head in black African neonates is the inferior gluteal artery, an anomaly that could explain why the disease is uncommon in black children.

Technetium scanning has also been used to study the vascular status of the femoral head in LCPD. Scintigraphic examination of the hips of 25 children with transitory synovitis found that 4 of the children had decreased uptake of radioisotope (i.e., decreased blood flow) initially, but 6 weeks later, 3 of the 4 children had normal or increased uptake. The single patient in whom decreased blood flow persisted was later diagnosed with LCPD. The investigators concluded that some patients with transient synovitis have coexisting transient ischemia of the femoral epiphysis and that those with more severe cases or recurrent episodes of ischemia are at greater risk for development of LCPD. Scans have also demonstrated decreased uptake in the upper pole of the femoral head, as well as a reduction in blood flow in the femoral artery on the affected side in the early stages of the disorder.
Venous Drainage of the Femoral Head and Neck
Abnormal venous drainage of the head and neck of the femur has also been noted in patients with LCPD. Venous drainage normally flows through the medial circumflex vein. However, in patients with LCPD, increased venous pressure in the affected femoral neck and associated venous congestion in the metaphysis have been observed, and venous outflow has been found to exit more distally through the diaphyseal veins ( Fig. 17-4 ). In a study of 55 hips, abnormal venous flow patterns were found in 46 of the femoral necks during the initial and fragmentation stages and in just less than 50% of the femoral necks during the restitution stage. Those hips with the most disrupted venous drainage also displayed the most severe radiographic changes. After the hips had healed, venous drainage returned to normal.

Although these abnormalities in venous outflow are a consistent finding in LCPD, it is unknown whether they are a cause of the disorder or a secondary effect of some other factors involved in the pathogenetic process. In a study in which silicone was injected into the femoral neck of a dog model, venous outflow was obstructed, intraosseous pressure increased, and AVN was produced. These results suggest that venous obstruction may play a pathogenetic role in the development of ischemic necrosis.
Systemic Abnormalities of Growth and Development: The “Predisposed Child”
Patients with LCPD share particular growth and development abnormalities, a finding indicating that some children may be predisposed to development of the disease and systemic factors may be involved in pathogenesis. A delay in bone age relative to the patient’s chronologic age is the most commonly observed abnormality and is seen in the early years of the disorder ( Fig. 17-5, A ). For example, the carpal bone age is frequently 2 years or more behind the chronologic age. Children diagnosed with the disease before 5 years of age have shown an increase in bone age delay over the following 4 to 5 years, whereas those diagnosed after 8 years of age had less delay over the ensuing years. A “radiologic pause” in the bone age has also been observed, with particular carpals affected more than others. This phenomenon had previously been referred to as skeletal standstill. Although growth of the triquetrum and lunate is significantly delayed, the capitate and hamate are not affected. In patients with bilateral disease, there is greater delay in bone maturation of the trapezoid, growth of the carpals is more delayed than is growth of the radius and ulna, and the onset of ossification in the carpals occurs later than in patients who have unilateral disease. In the later stages of the disease, bone maturation accelerates until the bone age matches the chronologic age ( Fig. 17-5, B ). Although it is clear that most patients with LCPD have characteristic bone maturation delays, how this delay relates to the pathogenesis of the disease is still uncertain.

The manifestation of discrete abnormalities in the patient’s opposite femoral head is another potential sign of systemic abnormalities in LCPD. Findings include femoral heads that are not as round as in control subjects (with anterior and lateral flattening), and flattening or dimpling of the femoral head in almost half of the patients. Thicker than normal articular cartilage, decreased endochondral ossification at the cartilage-bone junction, and sporadic calcification in the cartilage were reported in a study with a limited number of unaffected femoral heads. One study found significantly decreased center-edge angle and increased acetabular index angle in the unaffected hip of those patients with Stulberg class III, IV, or V affected hips at maturity.
Other growth abnormalities have been reported in children with LCPD. Although the birth weight of children with the disorder was found to be significantly lower than that of unaffected children, low birth weight did not correlate with subsequent short stature. At the time the disorder develops, some children are shorter than expected for their age, a condition that often continues for the rest of their lives. Boys may be approximately 1 inch shorter than their peers, whereas girls may be approximately 3 inches shorter.
After children experience a period of growth arrest, they may undergo a period of accelerated bone maturation. Children with the disorder have also been found to be in the low to normal percentiles when their stature was compared with their chronologic age. Growth studies have reported that soon after being diagnosed with LCPD, patients had a period of slowed growth velocity followed by a catch-up phase. There was normal onset of puberty, and by the time the children were 12 to 15 years of age, their stature and bone age were the same as those of their peers. Another study found that children diagnosed with the disorder before 6 years of age experienced a premature, unsustained acceleration in pubertal growth. In addition, the hands and feet of children with the disorder are often smaller than are those of siblings or peers.
Because of these systemic features of growth delay, the hypothesis postulating the alteration of the insulin-like growth factor (IGF) pathway as a cause of LCPD is interesting. IGF-I is known to stimulate growth and is expressed in various tissues, including brain and skeleton. Given that IGF pathway dysfunction can influence brain and skeletal development, it can potentially explain the delay in skeletal maturation and hyperactive behavior seen in the patients with LCPD. Lower plasma somatomedin C (now IGF-I) levels have been reported in children with LCPD who were small and had immature bone ages. A proposed explanation for the delay in growth is that plasma IGF-I increases in normal children as they grow older, but no such increase is noted in children with LCPD during the initial stages of the disorder. Low levels of serum IGF-I were observed during the first 2 years after the diagnosis of LCPD in a study consisting of 59 consecutive patients. A marked decrease in somatomedin A (now called IGF-II) has also been noted in patients with LCPD. In another study, the serum levels of IGF-I were found to be normal, but the levels of its major binding protein, IGF-I binding protein-3, were found to be decreased in their cohort. These results conflict with another study that found normal IGF-I activity and normal IGF-I binding protein levels. These differences in findings may stem from small sample size, different measurement techniques, and a nonstandardized definition of normal range for different age groups. Because of the conflicting results and the lack of evidence for a cause-and-effect relationship, the significance of the IGF pathway in the origin of Perthes disease remains unclear.
Some studies have reported normal thyroid function in patients with the disorder, whereas others have noted elevated plasma levels of free thyroxine and triiodothyronine compared with levels in control groups. Although the hormonal levels were increased in these patients, they were still within normal limits. A study that compared 139 children with LCPD with 220 healthy control subjects also found no difference in growth parameters and levels of thyroid-stimulating hormone, thyroxine, and IGF between the two groups.
Hyperactivity or Attention Deficit Disorder
Many children with LCPD tend to be very active physically, and some are pathologically hyperactive or suffer from attention deficit disorder. In a study of 68 children treated with recumbency, 48 became extremely hyperactive as they grew older, were in a perpetual state of motion, and were prone to climbing and falling. Another study of behavioral characteristics of children with LCPD found that one third of those children in the series scored abnormally high on psychological profiles identified with attention deficit hyperactivity disorder. Because of its high prevalence in this patient population, hyperactivity may have a role in the origin of the disease. Although its precise role remains to be defined, one can speculate that hyperactivity would be associated with greater mechanical loading of the hip joint and increased subclinical “trauma” that could influence the pathogenesis of the femoral head deformity and its outcome.
Trauma
Other authors have suggested that trauma in the predisposed child precipitates AVN of the femoral head and the development of LCPD. In the developing proximal femur, the major lateral epiphyseal artery must course through a narrow passage, which could make the vessel susceptible to disruption after trauma to this area. The risk of vascular interruption secondary to trauma is increased because these vessels must penetrate the thick cartilage of the femoral head. Despite these findings, the premise that trauma is a causative factor of the disease is particularly difficult to substantiate because frequent, mild trauma is common in childhood.
Hereditary Influences
Some authors have suggested genetic factors as a possible cause of LCPD and have noted the disorder in 44% of four generations of one family and in approximately 3% of siblings born after the index patient. Polygenic inheritance was suggested by the occurrence of LCPD in 0.8% of second- and third-degree relatives. After reviewing their own series of patients and six other published series, Burch and Nevelos concluded that an X-linked recessive factor and an autosomal homozygous allele were present in each genotype of the disease. G-455-A polymorphism of the beta-fibrinogen gene has been associated with LCPD, and passive smoking has been suggested to increase the risk of development of the disorder. Greater numbers of congenital abnormalities (e.g., hemivertebrae, deafness, Rubinstein-Taybi syndrome, imperforate anus, pyloric stenosis, epilepsy, congenital heart disease, undescended testicle, short tibia) have been observed in patients with LCPD. These findings, however, could be associated with genetic or environmental factors, and their significance is unknown. A multifactorial inheritance pattern has also been described in patients with LCPD in which the ratio of affected first-, second-, third-, and fourth-degree relatives to the general population of the same set was 35 : 4 : 4 : 1, respectively. In one report, four members of one family (three brothers and a male cousin) had LCPD, but the authors were not sure whether this was the result of genetic or environmental influences.
Other studies, however, indicate that a genetic component is minor and that there is little if any hereditary influence. Although a few reports exist of the disorder in twins, these cases were selected because of their concordance. In studies of unselected cases, only one of each pair of twins had the disease. In a report on the mating of affected persons who had monozygotic twins, both of whom had LCPD, the risk in siblings was less than 1% and the risk in children of an affected parent was only 3%.
In another review of 310 patients with the disorder, there was no indication of a hereditary factor. Within the study population, no parents and only 1.6% of siblings had the disease, and the incidence among second- and third-degree relatives was the same as that of the general population. The authors explained the high familial incidence of the disease reported in other studies as possibly the result of the unintentional inclusion of patients with familial epiphyseal dysplasias. When these misdiagnosed cases are eliminated, the true incidence of LCPD among first-degree relatives is very low.
Type II Collagenopathy
A few Asian families with multiple members affected by femoral head osteonecrosis in an autosomal dominant fashion have been reported. The affected family members were found to have a missense mutation in the type II collagen gene (replacement of glycine with serine at codon 1170 of COL2A1 ). In the affected children with this mutation, radiographic changes typical of LCPD were reported. In contrast to skeletal dysplasias and other type II collagenopathies, the affected individuals with this mutation did not appear to have overt skeletal abnormalities other than those affecting the hips. The possibility that this condition was a mild form of a skeletal dysplasia, however, has not been completely excluded. It is speculated that the mutation may cause a weakening of the cartilage matrix that may compromise the patency of the blood vessels within the femoral head cartilage with hip joint loading. The mutation has not been reported in unilateral, sporadic, or nonfamilial bilateral cases. Because these types of cases represent most patients with LCPD, this mutation may be a causative factor in a very small subset of patients with familial bilateral disease.
Environmental Influences
Environmental influences have been reported, primarily in Great Britain, as a possible cause of LCPD, which has a high occurrence in particular urban areas. A high incidence of the disease has been reported among children living in the inner city of Liverpool (16.9 per 100,000). In comparison with these data obtained in the period of 1976 to 1981, a follow-up study showed a significant decrease in the incidence to 8.7 per 100,00 in 1990 to 1995. Improvements in socioeconomic status and in nutritional status have been proposed as the factors associated with the decline in the incidence. Low levels of blood manganese were subsequently found in affected children. Another study from the United Kingdom found no increased prevalence of LCPD in urban areas but did note a relationship of the disorder with economic deprivation, a finding suggesting a nutritional influence in the origin of the disorder.
Exposure to smoking has been suggested as an etiologic factor. Patients with LCPD in one study had lower than average birth weights, and 61% of the mothers of affected children smoked, compared with 43% of their control group. In a group of 39 patients with LCPD who were nonsmokers, 62% had secondhand smoke exposure, and 71% had intrauterine exposure. This exposure is known to lower stimulated tissue plasminogen activator activity, thus leading to hypofibrinolysis and possibly to venous occlusion of femoral head vessels.
Synovitis
Synovitis of the hip occurs early in LCPD and may precede radiographic changes. Thus many investigators have studied the incidence of the disorder after synovitis to evaluate the possible role of synovitis. Findings from these studies clearly demonstrate that although synovitis may be the first manifestation of the disease, it is rarely, if ever, the cause of LCPD. In numerous studies, the rate of development of LCPD after an episode of synovitis has ranged from less than 1% to 4%.
Many children exhibit abnormal femoral head vascular hemodynamics during the acute stage of synovitis. However, in most cases normal circulation returns, AVN does not occur, and the patients do not develop LCPD. Although scintigraphy may show abnormal uptake of technetium phosphate initially, complete restoration of uptake occurs within 3 weeks in most children. Magnetic resonance imaging (MRI) was also used to study children with synovitis. Although patterns of low signal intensity on T1-weighted images indicated AVN, findings returned to normal in all cases and the patients’ symptoms resolved. The MRI results may have been related to transient edema of the bone marrow.
Studies have also been conducted to evaluate the position of the hip and its correlation with intraarticular pressure in patients with synovitis. A slight increase in pressure has been noted during the early stages of LCPD. Pressures were moderately increased in patients with idiopathic synovitis and extremely high in those with septic arthritis. The pressure increased markedly when the hip was extended and internally rotated. This positional increase in pressure has been reported by others. This finding suggests that when a patient is immobilized or placed in traction, the hip should always be in a position of at least 30 degrees of flexion.
Pathology
Histopathology
Perthes in 1913 was the first to describe the pathology of the disorder. His findings were based on the femoral head of a child who died accidentally while the hip was in the middle stages of disease progression. In 1914, Perthes’ colleague, Schwarz, provided his own description of a pathologic specimen in which he indicated that the findings were most likely a result of disrupted blood flow to the femoral head.
Although various studies have described the histopathologic changes present during the different stages of LCPD, limited availability of clinical specimens underscores one of the major obstacles in trying to understand the pathophysiology of a condition that has so much variability. Sources of the material include a limited number of whole head specimens from children who died accidentally, specimens obtained by curettage of the femoral head, and material from femoral head biopsies.
The stage of increased density is characterized by areas of necrotic bone with absent or pyknotic nuclei of the osteocytes. Findings also include a necrotic mass of dead marrow and pulverized particles of dead bone in the marrow spaces, termed trummermeh ( Fig. 17-6 ). The articular cartilage is usually thickened, indicating continued proliferation of chondrocytes. Although the superficial and the middle layers of the cartilage appear unaffected, extensive cell death has been reported in the deep layer of the cartilage overlying the necrotic bone. A subchondral fracture is sometimes found in the early phase with blood filling the space between the articular cartilage and the fragmented subchondral bone. Some specimens have demonstrated changes suggesting multiple episodes of ischemia, and at least one specimen showed thrombotic occlusion of the posterior inferior retinacular artery ( Fig. 17-7 ).


In the fragmentation phase of the disease, vascular connective tissue and other signs of repair are found, in addition to persistence of some necrotic bone. Although some investigators have characterized the revascularization process as “creeping substitution,” a more accurate description of the process is uncoupling or imbalance of bone resorption and bone formation with the predominance of the former and the delay of the latter, as reflected by the resorptive changes seen on radiographs in this phase of the disease with a delay in reossification ( Figs. 17-8 and 17-9 ). Loss of height of the femoral head secondary to collapse and resorption of the necrotic bone occurs in the fragmentation phase. The stages of repair are variable in different parts of the femoral head. The growth plate is irregular and may be disrupted, and columns of cartilage often extend into the adjacent metaphysis. These cartilage extensions in the metaphysis appear radiographically as metaphyseal lucencies. Cystic, fluid-filled lesions are also found in the metaphysis and are frequently seen on radiographs.


In the healing phase, reossification takes place and new bone formation, both woven and lamellar, predominates in the femoral head. The trabeculae and marrow spaces regain their normal architecture ( Fig. 17-10 and Box 17-3 ).

Early Stage
Dead trabecular bone and marrow necrosis
Necrosis of deep layer of epiphyseal cartilage
Thickened articular cartilage
Collapsed trabeculae
Subchondral fracture or separation of cartilage and bone junction
Physeal disruption
Cartilage extending from the physis into the metaphysis
Fragmentation Stage
Invasion of vascular granulation tissue in the marrow space
Increased vessels in the cartilage
Increased osteoclasts and bone resorption in the bony epiphysis
Restoration of endochondral ossification in the periphery of the epiphysis
Reossification Stage
New bone forming on old trabeculae
Woven new bone formation
Healing Stage
New bone, woven and lamellar
Return to normal architecture
Pathogenesis of Femoral Head Deformity
The limited availability of clinical samples has prompted the use of experimental models of ischemic osteonecrosis to investigate the pathogenesis of the femoral head deformity. In particular, a piglet model has allowed more systematic investigation of ischemic damage, repair process, and pathogenesis of the femoral head deformity following disruption of the epiphyseal blood supply. A relevant finding from the experimental studies is that the induction of ischemia produces a decrease in the mechanical strength of the necrotic femoral head that makes it prone to deformity from the early avascular phase of the model. The decrease in the mechanical properties of the necrotic femoral head appears to be multifactorial. These factors include necrosis of the deep layer of the articular cartilage, an increase in the mineral content of the necrotic calcified cartilage and the trabecular bone (thereby making them more brittle), and accumulation of microdamage in the necrotic trabeculae ( Fig. 17-11 ). Repetitive loading produces microdamage in the normal bone that is detected and repaired by osteoclasts and osteoblasts. In the necrotic bone, no bone cells are available to detect and repair the microdamage; thus they accumulate and weaken the bone in the avascular phase.

Revascularization of the necrotic region of femoral head with accompanying osteoclastic resorption of the necrotic trabeculae further decreases the mechanical strength of the infarcted femoral head. The imbalance of bone resorption produces areas of radiolucency within the necrotic femoral head and a fragmented appearance of the femoral head ( Fig. 17-12 ).

Because the affected femoral head is weakened in the active phase of the disease, the effect of hip joint loading in the development of the femoral head deformity should be considered. The hip contact pressures associated with various activities in children are unknown. In adults, a femoral head prosthesis equipped with a strain gauge allowed collection of these data in a limited number of patients following total hip replacement. The study revealed that substantial hip contact pressures are generated with normal activities. For instance, normal walking produced hip contact pressure approximately 2.5 times body weight with each step. Running on a treadmill (rate of 8 km/hr) produced a hip contact pressure approximately 4.5 times body weight with each stride. Increasing the speed of walking or running produced greater hip contact pressures. Furthermore, certain supine and prone activities, such as hip extension or straight-leg raising, elevated the hip contact pressures to more than body weight. Although these pressures are well tolerated by a normal femoral head, an infarcted femoral head with a lack of bone cells to repair microdamage and increased resorption may not be able to resist deformation associated with these significant forces, which are repetitive. An experiment using the piglet model of ischemic necrosis showed that weight bearing contributes significantly to the development of femoral head deformity in that model. The animals in the weight-bearing group had significantly greater femoral head deformity compared with the non–weight-bearing group, which had above-knee amputation. Because the femoral head is weakened during the healing process in Perthes disease, avoidance of activities that generate a significant increase in the hip contact pressure during the active phase of the disease seems reasonable. More research is required to define what “significant” loading is and whether current treatment strategies to restrict activities and decrease weight bearing are effective in preventing the deformity.
Findings in the Epiphyseal Cartilage
Studies of cartilage samples from the patients with LCPD and large animal models of ischemic necrosis showed that epiphyseal and physeal cartilage involvement is a pathologic feature of a vascular disruption of the epiphysis. In a histochemical and ultrastructural examination of the epiphyseal cartilage and physis of the lateral portion of the femoral head and neck, a thick region of epiphyseal cartilage was found under the normal articular cartilage ( Fig. 17-13 ). This zone of epiphyseal cartilage was composed of clearly separated sections of hypercellular and fibrillated cartilage with pronounced blood vessels. There also were numerous, asymmetrically oriented large collagen fibrils and varying amounts of proteoglycan granules. The fibrillar area had several distinct findings: (1) increased amounts of proteoglycan, (2) a reduction in structural glycoproteins, and (3) collagen fibrils that differed in size from normal epiphyseal cartilage. The border of the lateral physis, which frequently was asymmetric with a significant decrease in collagen and proteoglycan granules, contained profuse amounts of large lipid inclusions. The investigators speculated that these findings represented a localized manifestation of a generalized, transitory epiphyseal cartilage disorder, comparable to changes observed in the vertebral end-plates in juvenile kyphosis. These investigators concluded that the epiphyseal change was primary, whereas femoral head collapse and necrosis could be caused by deterioration and disarray of the matrix of the epiphyseal cartilage and subsequent abnormal ossification.

In large animal models of ischemic osteonecrosis, induction of ischemia was shown to produce cell death in the deep layer of the epiphyseal cartilage with subsequent degenerative changes in the cartilage matrix. Furthermore, the viable, superficial region of the epiphyseal cartilage was found to highly express a potent angiogenic factor, vascular endothelial growth factor (VEGF). These findings suggest that the epiphyseal cartilage is a site of ischemic damage as well as a site of repair response to the ischemic damage.
Findings Suggestive of Double Infarction
Two investigations suggested that two infarcts of the femoral head were required for LCPD to develop. In an experiment performed on young dogs, a single-infarct surgical procedure did not reproduce the changes typically associated with the disorder in humans. Other experimental studies also found that a single episode of infarction did not reproduce the anatomic changes seen in LCPD. In a canine model, when a second infarction surgical procedure was performed 4 weeks after the first, the resultant changes were comparable to those seen in humans. Examination of femoral biopsy specimens obtained from 51 patients found that 51% of the specimens displayed changes similar to those seen with double infarctions ( Fig. 17-14 ). It is notable that in the piglet model of ischemic osteonecrosis, one episode of ischemia produced by placing a ligature around the femoral head and transecting the ligamentum teres was sufficient to produce pathologic changes resembling the human disease.

Catterall and associates proposed that moderate disease (Catterall groups II and III) involved two incidents of infarction and severe disease (Catterall group IV) had repeated episodes of infarction. This prevailing interpretation of the findings implies that if the cause of the infarction can be stopped or treated early, then the extent or the severity of the disease can be minimized. An alternative interpretation of the double infarction theory is that the disease is caused by one infarction event, but subsequent mechanical loading may further collapse the femoral head, reinjure the vessels in the healing areas of the femoral head, and produce secondary episodes of infarctions. This interpretation implies that avoidance of mechanical overloading and prevention of deformity would be beneficial to the healing process.
Metaphyseal Changes
Four types of metaphyseal changes have been observed: (1) an overabundance of fatty marrow, (2) circumscribed osteolytic lesions with a sclerotic border, (3) a wide physis with disarrayed ossification and columns of unossified cartilage coursing down into the metaphysis, and (4) extension of the physis down the side of the neck of the femur. Three types of changes in the upper femoral metaphysis were found in a pig model of LCPD. In type I, focal thickening of physeal cartilage extended down into the metaphysis. Some of these animals had cystic degeneration of the thickened cartilage. In type II, central disruption of the physis occurred, with resorption and replacement of metaphyseal bone by fibrovascular tissue. Type III had diffuse resorption of the physeal cartilage and resorption of adjacent metaphyseal and epiphyseal bone. These changes occurred 8 weeks after the onset of ischemia and are probably associated with substantial growth disturbance of upper femur. The metaphyseal lucencies seen on radiographs represent unossified cartilage from the growth plate.
Clinical Features
In 1920 Sundt described the clinical manifestations of LCPD; his description still holds true today ( Table 17-1 ). He noted that the onset of the disorder occurred between 2 and 12 years of age (with a peak between 6 and 8 years), that boys were four times more likely to have the disease than girls, and that 10% of patients had bilateral disease. Observations made by Catterall in 1982 supported Sundt’s findings. Catterall reported that the mean age at onset was 6 years (82% of patients were between 4 and 9 years of age) and that boys were 3.7 times more likely than girls to be affected. Sundt also noted that because symptoms were frequently mild, considerable time often passed before the child was seen by a physician. On presentation the child usually had a limp, and some children complained of pain in the hip, thigh, or knee. The primary findings on physical examination were limited range of motion of the affected hip (particularly in abduction and internal rotation) and slight atrophy of the femoral muscles.
Stage | Features | Radiographic Changes |
---|---|---|
I | Stage of increased density | Ossific nucleus initially smaller; femoral head becomes uniformly dense; a subchondral fracture may be seen; radiolucencies appear in the metaphysis |
II | Fragmentation stage | Varying patterns of lucency appear in epiphysis; segments (pillars) of the femoral head demarcate; the femoral head may flatten and widen; metaphyseal changes resolve; acetabular contour may change |
III | Healing or reossification stage | New bone appears in femoral head which gradually reossifies; epiphysis becomes homogeneous |
IV | Healed or remodeling stage | Femoral head is fully reossified and remodels to maturity; acetabulum also remodels |
In 1964 Johannes Meyer described a group of cases in which unusual ossification abnormalities were found in a group of children thought to have LCPD. These hips had delayed and irregular ossification of the capital femoral epiphysis and no deformation of the head over time, and they were usually asymptomatic. Although considerable literature is devoted to Meyer disease or, as he termed it, dysplasia epiphysealis capitis femoris, it is likely that this is a mild variation of LCPD in a younger age group. In fact, Meyer’s original report stated, “There is obviously no question of different diseases.” He noted that many children had the dysplasia type in one hip followed by the usual type of LCPD in the other hip. He postulated that the different manifestations were the result of age-related changes in the blood supply to the femoral head. We concur with Meyer’s opinion that this is not a separate entity, but a mild version of the same process in a young patient.
Symptoms
Patients most often present with a limp, which is usually first noticed by a parent. The limp is exacerbated by strenuous physical activities and alleviated with rest. The limp may be more pronounced later in the day if the child has been involved in extended periods of ambulation. The second most frequent complaint is pain, which may be located in the groin, anterior hip region, or laterally around the greater trochanter. The patient often has referred pain to the knee or anterior thigh that may obscure the diagnosis. The pain is aggravated by increased physical activity and is usually worse late in the day. The child occasionally has night pain.
The patient or parents may recollect an isolated incident of trauma (often a fall or twisting injury) several months earlier, followed by the onset of a limp and hip pain. After a few days, these initial symptoms normally resolve completely. The patient often goes through periods of exacerbation and alleviation, with the symptoms waxing and waning. To relieve the pain, the child may deliberately decrease his or her normal level of activity. If the symptoms are mild, several months may pass before the child is seen by a physician.
The parent often describes the child as being extremely active—in perpetual motion, running and jumping more than other children. Some patients may exhibit attention deficit disorder or may have been diagnosed as pathologically hyperactive. The child may also be smaller in stature than peers. All these details paint the classic portrait of the child with LCPD—small, often thin, extremely active, constantly running and jumping, and limping after strenuous physical activities. Occasionally, however, a youngster with the disorder is just the opposite: overweight and physically inactive.
Sometimes a relative of the patient may also have the disorder, although a family history of LCPD is rare. A strong family history of hip problems (particularly bilateral) may indicate a diagnosis of familial epiphyseal dysplasia ( Box 17-4 ).
Signs
The physical examination should include observation of the child’s activity level. The findings of physical examination vary depending on the stage of the disease and become more pronounced as the femoral head develops fragmentation and deformity. The child has a limp, which he or she often attempts to conceal during the examination. When possible, the physician should indirectly observe the child as the child is walking to the examination room. The child’s limp is normally a combination of an antalgic gait and a Trendelenburg gait ( Fig. 17-15 ). In the stance phase of gait, the child often leans the body over the involved hip to decrease the force of the abductor muscles and the pressure within the hip joint. The Trendelenburg test result is positive on the involved side ( Fig. 17-16 ). Depending on the duration and severity of the condition, the examiner may note varying degrees of atrophy of the gluteus, quadriceps, and hamstring muscles.


During the early phase of the disorder, reduction of hip motion (which is caused by muscle spasm or involuntary guarding related to hip synovitis) varies from patient to patient. By examining the hip very gently, the clinician can usually elicit a greater range of motion because muscle spasm is avoided (the same holds true if the child is examined after a night’s rest). Early in the disease process, only slight loss of abduction and internal rotation is noted. In these patients, the logroll test is positive. Hip abduction is best examined in extension with the patient supine. Dropping the uninvolved leg over the side of the examining table helps stabilize the pelvis ( Fig. 17-17 ). When the examination is done properly, true abduction is less than originally appreciated. The degree of rotational loss is best assessed with the patient both prone and supine. Normally, at this stage of the disease, there is no flexion contracture.

As the disorder progresses, findings on the clinical examination may be significantly different. Patients with mild disease may experience only slight loss at the extreme ranges of motion and may rapidly regain normal mobility of the leg. Patients with more severe disease have greater loss of motion, particularly of abduction and internal rotation. When the hip is flexed, it may go into obligatory external rotation. In severe cases adduction contractures develop and the child may lose all rotation of the hip. The range of flexion and extension, however, is seldom affected.
Clinical Course
Waldenström’s observation that the clinical course of the disease is variable remains true today. He observed that although some children experienced only minor symptoms and minimal changes in the shape of the femoral head, most had a more severe course, resulting in pain while walking and greater loss of limb motion. Waldenström defined the stages of the disease as shown in Table 17-1 . His classification has been modified by most authors to the four stages of initial, fragmentation, healing (reossification), and residual phases. In a retrospective study, we found that the time from first radiographic evidence of disease to the start of fragmentation was a mean of 6 months (range, 1 to 14 months), the fragmentation phase lasted 8 months (range, 2 to 35 months), and the healing stage occupied 51 months (range, 2 to 122 months).
Clinical findings correspond to some degree with the radiographic stages of the disease ( Table 17-2 ). During the early stage of the disorder, radiographs show only increased density of the femoral head, and the patient may experience recurrent aggravation and alleviation of symptoms and signs. There may be only mild limp and pain for a time, interrupted by episodes of moderate discomfort lasting a couple of weeks. During the latter phase, a subchondral fracture is frequently noted on radiographs (the Salter sign), and the patient’s clinical status may worsen.
Stage | Clinical Findings | Radiographic Changes |
---|---|---|
Increased density stage | Limp and pain variable, often mild and intermittent | Increased density of femoral head, with/without subchondral fracture |
Fragmentation stage | Pain and limp may worsen; may lose range of motion | Head shows fragmentation, may lateralize and flatten |
Reossification stage | Limp and pain gradually resolve, range of motion improves | Femoral head gradually reossifies; flattening of head may improve |
Healed stage | Occasional limp; occasional locking, popping | May develop osteochondrotic lesion |
At the beginning of the fragmentation stage the femoral head starts to collapse and may extrude laterally from the acetabulum. The patient’s limp and pain are more pronounced, and there is a greater loss of range of motion of the affected limb. Because the femoral head is deformed, resting the hip usually does not return normal motion to the joint. In mild cases with minimal change in the shape of the femoral head, symptoms and signs may be limited. Patients who have a very brief fragmentation stage are asymptomatic. In more severe cases, however, clinical symptoms and signs progressively worsen throughout the fragmentation phase.
The beginning of the healing stage is characterized radiographically by the development of new bone in the subchondral regions of the femoral head. By this time, pain and limp have usually started to resolve, but some limitation of motion remains. The degree of motion restriction is directly related to the extent of change in the shape of the femoral head. Usually the child gradually resumes normal activities without complaints. Symptoms are normally absent as the femoral head becomes completely reossified.
If reossification in the central segment of the femoral head is significantly delayed, the patient may begin to experience pain after several asymptomatic years. A loose fragment or osteochondritis dissecans lesion may subsequently develop in this soft portion of the head. The child complains of locking and popping of the joint, and crepitus may be present on physical examination.
Natural History of the Disease
Because treatment programs were started as soon as LCPD was identified, no definitive natural history studies exist of persons who were completely untreated. Thus the natural history of the disorder has been difficult to ascertain accurately. We have been forced to surmise the likely natural history by reviewing groups of patients managed by programs that are now regarded as ineffective, with the presumption that these often intense and long-term treatment methods (e.g., non–weight bearing, cast immobilization, use of patten-bottom braces) did not affect the course of the disorder either favorably or adversely. It is improbable that these therapies had no effect whatsoever on patients’ hips; however, we must accept, with some reservations, the findings of these reviews to gain knowledge of the disease process because better alternatives are lacking.
The course of the disease varies considerably from patient to patient ( Box 17-5 ). Most children experience moderate symptoms and endure 12 to 18 months of difficulty, followed by gradual resolution of the symptoms and a return to normal physical activities. At one extreme, some children have minimal, transient symptoms, whereas patients with more severe involvement have severe symptoms that persist into late adolescence or early adulthood.
- •
Disease severity: varies from mild to severe, with most children experiencing moderate symptoms for 12 to 18 months, followed by complete resolution of symptoms and a return to normal physical activities
- •
Patient’s age: most consistent factor affecting course of disease; usually, mild disease in those with early onset (<6 years of age), moderate symptoms in those with onset between 6 and 9 years of age, and most severe course and worst outcome in those with onset at 9 years of age or older
- •
Extent of radiographic changes: varies, with the poorest results seen in those hips with the greatest degree of involvement
- •
Outcome: affected by (in addition to patient’s age at onset) duration from onset of disease to complete resolution: the shorter the duration, the better the final results
The age of the patient at onset of disease is the most consistently reported factor affecting the course of the disorder. In general, the younger the child, the better the outcome will be. The youngest of these children, especially those younger than 4 years of age, are often asymptomatic and have no deformation of the femoral head. These children are often referred to as having Meyer dysplasia, which we consider to be a variant of LCPD. Those with early onset (particularly <6 years) usually experience mild disease, those with onset between 6 and 9 years of age normally have moderate symptoms, and children 9 years of age or older at onset have a more severe course and more frequently have a poor outcome. However, a young age of onset does not ensure a good result. Patients with onset after the age of 12 years have the worst prognosis, with three patterns of femoral head necrosis identified in this group: late-onset pattern, segmental collapse pattern, and destructive pattern. The third pattern, characterized by severe head collapse without reossification, often results in severe symptoms and loss of function in late adolescence.
The extent of radiographic changes also varies, and the poorest results are seen in hips with the greatest degree of involvement. Classification systems have been developed to estimate the severity of disease based on radiographic findings. Legg described a “cap” head and a “mushroom” head, with the cap more common and less severe than the mushroom. Waldenström recognized three types of radiographic changes; the most severe type occurred least often but resulted in the poorest outcome. In Catterall’s four-part classification system, coexisting risk factors increased the chances of a poor outcome. In the lateral pillar classification system, the degree of involvement of the lateral segment of the femoral head is graded as A, B, B/C border, or C, with C the most severe.
Outcome is also affected by the duration of disease from onset of the disorder to complete resolution. The shorter the duration of disease, the better the final results will be. In a series of patients with disease classified according to the lateral pillar system, group A hips healed in 37 months, with a 100% good or excellent outcome; group B hips healed in 50 months, with a 79% good or excellent outcome; and group C hips healed in 67 months, with a 29% good or excellent outcome.
Radiographic Findings
Radiographic Staging of Disease Evolution
Waldenström’s classification of the stages of LCPD based on radiographic changes is summarized in Table 17-1 . Commonly, four radiographic stages—initial, fragmentation, reossification, and healed—are attributed to Waldenström. A modified version of Waldenström’s classification—initial, fragmentation, reossification (healing), and residual stages—is presented in Table 17-3 . The more severe the disease is, the longer the duration of each stage will be, particularly the healing phase.
Group | Lateral Pillar Radiographic Findings * |
---|---|
A | No density change |
No loss of height | |
B | Some density change |
Height ≥50% | |
Central pillar collapse | |
B/C | Thin lateral pillar |
Borderline height | |
C | Height <50% |
Initial Stage
Slight lateralization of the femoral head in the acetabulum and a slightly smaller ossific nucleus resulting from cessation of growth of the capital epiphysis are the initial radiographic signs of LCPD. The apparent widening of the medial joint space may be caused by synovitis and hypertrophy of articular cartilage ( Fig. 17-18, A ). Hypertrophy of the cartilage has been verified in pathologic specimens. Enhanced computed tomography (CT) has also demonstrated early lateralization secondary to swelling of the ligamentum teres. Another sign, capsular swelling, was also reported as an early radiographic change. However, this finding was later shown to be a result of the position of the hip (abducted and laterally rotated) while the radiograph was made, rather than a pathologic condition.

During the first stage of the disorder, other radiographic changes can be observed. In approximately one third of cases, in the earliest phase of the disease a linear fracture is noted in the subchondral area of the femoral head ( Fig. 17-19 ). Known as the Waldenström sign, it is usually best seen on the frog-leg lateral view. On rare occasions the fracture fills with gas on this radiographic projection (presumably because of a vacuum phenomenon that generates intraarticular gas during forced frog-leg positioning). Another indication of early disease is a small increase in the density of the ossific nucleus that can be seen better on lateral views.

Subsequently the femoral head appears progressively more radiodense. This increase in density is thought to be secondary to deposition of new bone on the dead bone trabeculae in the head. However, a more probable explanation is the increased mineral content of the necrotic calcified cartilage and bone. An experimental study showed that it is unlikely that the deposition of new bone occurs in the avascular, necrotic region of the femoral head in the early stages of the disease. Increased radiodensity occurs regardless of the extent of any ensuing femoral head collapse. Additional radiographic findings include clearly delineated cysts and indistinct lucencies in the metaphysis. The end of this stage occurs when lucencies resulting from bone resorption are seen within the ossific nucleus. This radiographic phase normally lasts a mean of 6 months and has a maximum duration of 14 months.
Fragmentation Stage
The second radiographic stage is fragmentation (see Fig. 17-18, B ). This stage is also referred to as the stage of resorption because lucencies develop in the ossific nucleus (evolving rapidly within several months), generally in the medial and lateral aspects of the femoral head. Often a dense fragment in the anterocentral region becomes demarcated from the medial and lateral segments of the femoral head. In more severe disease, no separation exists between the central and lateral portions and it may not exist between the central and medial portions. The end of the fragmentation stage is marked by the appearance of new bone in the subchondral sections of the femoral head. This is the stage when most of the femoral head deformity (flattening) occurs suggesting that the femoral head is mechanically the weakest during this stage. This radiographic stage lasts a mean of 8 months (range, 2 to 35 months). In mild disease, fragmentation may be observed only on a frog-leg lateral view, whereas on the anteroposterior (AP) view a slightly mottled density may be noted. This finding indicates that only the anterior segment of the epiphysis is necrotic. The mildest cases have no actual fragmentation phase, and healing of the femoral head with gradual resolution of the dense areas follows the second radiographic stage.
Reossification (Healing) Stage
The third radiographic stage is the healing phase, which starts with the manifestation of new subchondral bone in the femoral head (see Fig. 17-18, C and D ). Reossification frequently starts in the medial and lateral aspects of the femoral head and proceeds centrally. Normally, the last areas to reossify are the anterior and central segments of the femoral head (demonstrated on the frog-leg lateral view). The lucent portions of the femoral head represent fibrovascular tissue that gradually fills in with woven bone, and over time the new bone remodels into trabecular bone. The healing phase concludes when the entire head has reossified. This radiographic stage lasts a mean of 51 months (range, 2 to 122 months).
In the past it was believed that the shape of the femoral head was minimally altered during the healing stage. We now know that most femoral heads gradually improve during this period and regain their roundness ( Fig. 17-20 ). A few femoral heads, however, gradually flatten, primarily in children with disease onset before age 5 years and whose femoral head is totally affected by the disease.

Residual Stage
The fourth radiographic stage is the residual stage, during which no additional changes are noted in the density of the femoral head. However, the shape of the femoral head may continue to evolve, and only at the completion of skeletal growth is its permanent contour established (see Fig. 17-18, E and F ). After healing, the shape of the femoral head may vary from completely normal to extremely flat and aspherical. If the disease has disrupted growth of the capital physis, gradual relative overgrowth of the greater trochanter may occur during the residual stage.
Joseph and co-workers evaluated a large cohort of untreated patients and quantified the duration of the stages of the disease. These investigators divided the stages as follows: Ia, characterized by increased density without loss of epiphyseal height; Ib, with increased density and some loss of height; IIa, with earliest fragmentation; IIb, with more advanced fragmentation; IIIa, with first appearance of new bone formation; and IIIb, with new bone formation with normal texture over one third or more of the epiphysis. Stage IV hips were fully healed. Median durations of the stages were approximately 4 months for Ia, 3 months for Ib, 4 months for IIa, 4 months for IIb, 7 months for IIIa, and 11 months for IIIb. Joseph and associates found that most femoral head deformity and extrusion, metaphyseal widening, and acetabular deformation occurred between stages IIb and IIIa.
Other Radiographic Findings
Changes in the Metaphysis
Debate continues on the nature and importance of radiographic changes observed in the metaphysis proximate to the affected capital epiphysis. Theories have ranged from metaphyseal necrosis to tongues of fibrillated cartilage stretching deep into the femoral neck to resorption associated with revascularization as healing occurs. These metaphyseal changes have been suggested to have prognostic value; hips with cystic changes were found to be twice as likely to have poor outcomes as hips without cysts.
Later MRI studies corroborated the theory that the lucencies result from physeal cartilage extending into the metaphysis and challenged the idea that the cysts are located entirely within the metaphysis ( Fig. 17-21 ). These studies suggested that most metaphyseal “cysts” seen on plain radiographs are actually located within the epiphysis or physis and that their appearance in the metaphysis is probably a projection artifact. Other investigators also reported that few cysts are actually located strictly within the metaphysis.

The “sagging rope” sign, a radiodense line overlying the proximal femoral metaphysis ( Fig. 17-22 ), is a result of physeal damage associated with significant metaphyseal response. The edge of the rope is in fact the anterior portion of the overlarge femoral head as it projects over the metaphysis.

Changes in the Physis
Patients with LCPD often experience growth disturbance of the proximal femoral physis ( Fig. 17-23 ). Although certain radiographic findings associated with abnormal physeal formation have been described, there are no reliable early radiographic indications of growth interference. MRI has been shown to provide more accurate assessment of the proximal femoral physis than plain radiography. In a study of 23 patients, transphyseal bone bridging seen on MRI was found in 63% of the hips. MRI provided greater sensitivity (94%), specificity (89%), and positive predictive value (94%) than radiography.

Premature physeal closure was reported in one fourth of patients with LCPD ; however, this prevalence is considerably greater than that observed in other studies. Early closure was assumed based on greater trochanteric overgrowth, physeal shape changes, lateral extrusion of the capital nucleus, and medial bowing of the femoral neck.
In patients with evident premature closure of the epiphysis, coexisting overgrowth of the trochanter has not been associated with a Trendelenburg gait. Several deformities related to early femoral neck physeal closure were described. With central closures, the femoral head was round, the neck was short, and there was greater trochanteric overgrowth. With lateral arrests, the femoral head tilted laterally, the medial neck was longer, and there was overgrowth of the trochanter.
Changes in the Acetabulum
In most cases, changes in the shape of the femoral head are accompanied by acetabular changes. When the femoral head protrudes from the acetabulum, the medial wall may form what looks like a second compartment for the head. Referred to as bicompartmentalization, this change was suggested to be an indication of a poor outcome. Bicompartmental changes have also been associated with early closure of the triradiate cartilage. Osteoporosis of the roof of the acetabulum was noted during the initial phases of the disorder, and it peaked in the middle phases. The bone returned to normal when the patient reached skeletal maturity. The position of the femoral head, rather than its shape, has also been proposed to be the most significant factor in growth and remodeling of the acetabulum. Bicompartmentalization of the acetabulum usually resolves during the healing stage of LCPD.
Acetabular retroversion is commonly found in patients with LCPD. One study reported that 21 of 50 hips (42%) had acetabular retroversion in comparison with 7 of 112 hips (6%) in normal controls. Stulberg class III, IV, or V hips had a higher prevalence of retroversion compared with Stulberg class I or II hips. In a study investigating the timing of development of acetabular retroversion, a very low prevalence (1.8%) was found early after diagnosis, whereas the prevalence was 31% past skeletal maturity. In contrast, acetabular retroversion, as evidenced by a positive prominence of the ischial spine sign, was present early in the disease process in 37 of the 41 skeletally immature hips in another study. A prominence of the ischial spine on the affected side, however, may also result from a hip flexion contracture that tilts the pelvis when the examiner tries to obtain an AP pelvis view. Further studies are needed to define the pathogenesis of acetabular retroversion in LCPD more clearly.
Limited Radiographic Changes
Occasionally, radiographic changes seen in the young patient differ in appearance and evolution from the typical changes of LCPD. These hips may have delayed appearance of the capital ossific nucleus followed by development of multiple granular areas of ossification. These changes may be either unilateral or bilateral. The heads gradually ossify and do not progress through the stages of increased density, fragmentation, and healing, and no deformation of the head occurs. These changes have been termed Meyer dysplasia, but they probably represent a different pattern of vascular disruption in an immature epiphysis. In a series of 24 cases we found minimal radiographic changes that were limited to specific segments of the femoral head: 10 in the anterior portion, 7 in the posteromedial portion, 4 in the central portion, and 3 in the lateral portion. These changes developed over time, and all the femoral heads lost some height compared with the opposite femur. These changes were most likely the result of a minimal form of LCPD in which segmental patterns of blood supply to the femoral head dictated which locations were affected. In patients with unilateral disease, small changes have been reported in the opposite hip, such as less than normal roundness of the femoral head with flattening of the anterior segment. A central notch in the femoral head, which is a radiographic projection of the femoral head fovea, is often mistaken for avascular change ( Fig. 17-24 ).

Bilateral Changes
Density changes seen in both femoral heads may or may not represent LCPD. Four distinct patterns of bilateral hip disease have been reported. In type 1, the radiographic changes and their evolution are exactly the same for both hips. This pattern most likely indicates a form of multiple epiphyseal dysplasia, and the physician should examine other joints to verify the diagnosis. In type 2, changes are seen at the same time bilaterally, but fragmentation occurs only in one hip. In type 3, characteristic initial changes are noted in one hip, whereas radiographs show healed changes in the contralateral hip. In type 4, changes occur sequentially in both hips, with a more severe course seen in the hip involved second. True bilateral LCPD appears sequentially in the two hips, as in types 3 and 4.
Magnetic Resonance Imaging
MRI is an accurate imaging modality for the early diagnosis of LCPD and for visualizing the configuration of the femoral head and acetabulum ( Figs. 17-25 and 17-26 ). The varying modalities of MRI, such as perfusion and diffusion MRI and delayed gadolinium-enhanced MRI (dGEMRIC), may offer new insights into the pathophysiology and prognostication of this disorder.


Earlier, there was some equivocation regarding the efficacy of MRI compared with scintigraphy. Noncontrast MRI relies on signal changes from fat present in the epiphysis to detect AVN. Because it takes weeks to months for the fat signal to decrease, false-negative results of noncontrast MRI were reported in few isolated cases that manifested very early ( Fig. 17-27 ). In a study of nine patients in the early stage of Perthes disease, the extent of epiphyseal involvement was clearly visualized on MRI 3 to 8 months after the first symptoms. In a comparative study, MRI was found to be more accurate in the early diagnosis than other imaging modalities, with a diagnostic accuracy of 97% to 99% for MRI compared with 88% to 93% for radiography and 88% to 91% for scintigraphy. MRI has also been reported to provide earlier and more reliable information about the true extent of femoral head necrosis than radiography or scintigraphy. More recently, dynamic gadolinium-enhanced subtraction MRI was shown to be as effective as scintigraphy in delineating epiphyseal necrosis early in the disease process. The subtraction MRI technique facilitates the visualization of areas that lack gadolinium distribution (see Fig. 17-27 ). The ischemic area appears as a widespread absence of enhancement. Subtraction MRI also allows recognition of early reperfusion patterns. In one study, contrast-enhanced MRI better delineated the revascularization patterns in the femoral head than did scintigraphy. Bipositional MRI has been reported to be as effective as arthrography in evaluating femoral head sphericity and containment.

Sequential MRI studies of LCPD established a correlation with the Catterall classification system. Images showed substantial amounts of viable bone medially, laterally, and posteriorly in group II hips, considerable necrosis in group III hips, and physeal involvement with repair extending into the metaphysis in group IV hips. De Sanctis and colleagues proposed a classification based on MRI findings of percentage of head necrosis, lateral extrusion, and physeal disruption. Group A had less than 50% head involvement and group B greater than 50% necrosis. Within group B, the classification ranged from B0 to B3 based on the degrees of lateral extrusion and physeal disruption. Groups B2 and B3 were associated with a poor prognosis.
Scintigraphy
Technetium scanning is a sensitive means of diagnosing LCPD in its early stages, before associated radiographic findings are apparent ( Fig. 17-28 ). Scintigraphy has also been used to classify the severity of the disease, with grade I representing one-fourth epiphyseal involvement and grade IV representing complete involvement. Other investigators have reported that bone scintigraphy provides more accurate information about the extent of the necrosis than does initial radiography, and the scans are able to reveal revascularization and consequently the stage of the disease. § Care must be taken, however, because early bone scans may suggest a more severe condition than really exists ( Fig. 17-29 ).


§ References .
Scintigraphy has also been used to classify revascularization as either recanalization of existing vessels (the A track or pathway) or neovascularization, meaning new vessel formation (the B track or pathway). The investigators also noted that a hip could change from an A track to a B track in a few cases. They defined an A track as an indication of uncomplicated, fast revascularization of the femoral head that can take days and a B track as representing a slower process of new vessel formation that can occur over many months to several years. In one study, the patients on the A track had a favorable prognosis, whereas patients on pathway B generally had a poorer prognosis and often required surgical intervention. That the healing pattern may change from the A to the B pathway makes early prognostication difficult. To apply the scintigraphic classification, a pinhole collimator to magnify the images of the hips is required. A routine static scintigram of entire pelvis or a single-photon emission CT (SPECT) is not adequate to visualize the details of the scintigraphic patterns of LCPD. The application of the classification also requires two serial scintigrams. In terms of the validity, the classification lacks tissue confirmation of the recanalization and neovascularization pathways. Intraobserver and interobserver reliabilities of this classification are moderate. Physiologic events in the acetabulum have also been studied with scintigraphy. Radiographic changes in the acetabulum have been shown to be accompanied by increased uptake of tracer in the lateral portion of the acetabulum.Arthrography
Arthrography clearly shows the configuration of the femoral head and its relation to the acetabulum. An increase in the medial joint space with concomitant lateralization of the femoral head has been frequently observed on plain radiographs. Arthrographic studies have shown that the apparent widening in the joint space results from thickening of the articular cartilage. Changes in the shape of the femoral head also result in lateral shifting of the femoral head. Arthrography can also provide reliable information regarding containment of the femoral head within the acetabulum. The major advantage of arthrography is that the examiner can assess the congruity of the hip in many different positions.
Despite the positive aspects of arthrography, its use in the diagnosis and evaluation of LCPD is controversial. Plain radiography has been suggested to provide adequate information to evaluate femoral head protrusion (particularly if the acetabulum-head quotient is used).
Currently, arthrography is most often used in the assessment of loss of hip containment and hinge abduction of the hip, in which the femoral head “hinges” out of the acetabulum when the hip is abducted. Hinge abduction sometimes occurs early in the course of LCPD, and the longer it remains untreated, the worse the final outcome will be.
Ultrasonography
Ultrasonography is sometimes used in the early stages of LCPD to demonstrate joint effusion and in later stages to assess the shape of the femoral head. Ultrasonography can provide a good profile of the cartilaginous femoral head (comparable with that of arthrography) and allows subsequent observation of deformation of the head without the need for radiographs. A four-stage classification system based on ultrasonography and sonoanatomic criteria was suggested for patients with LCPD.
Sonography has a role in the evaluation of blood flow in the femoral head. Ultrasonography with microbubble contrast enhancement has been used to evaluate the vascularization of the femoral head. Other investigators have shown the ability of Doppler ultrasonography to demonstrate vascular flow in the deep capsular vessels of the femoral head.
Computed Tomography
CT can provide accurate three-dimensional images of the shape of the femoral head and acetabulum. A classification system was developed based on CT findings. In group A, only the periphery of the femoral head is affected; in group B, there is considerable necrosis of the central portion of the head but no posterior involvement; and in group C, the entire head is affected.
Despite these reports, CT is not typically used on a routine basis to evaluate patients with LCPD, and clinical decisions are not usually made on the basis of early CT findings. CT may be of benefit, however, in the later stages of the disease to evaluate pain, locking of the joint, and other mechanical symptoms. CT of the hip may help the clinician distinguish the cause to be an area of incomplete reossification within the femoral head or a true osteochondrotic lesion.
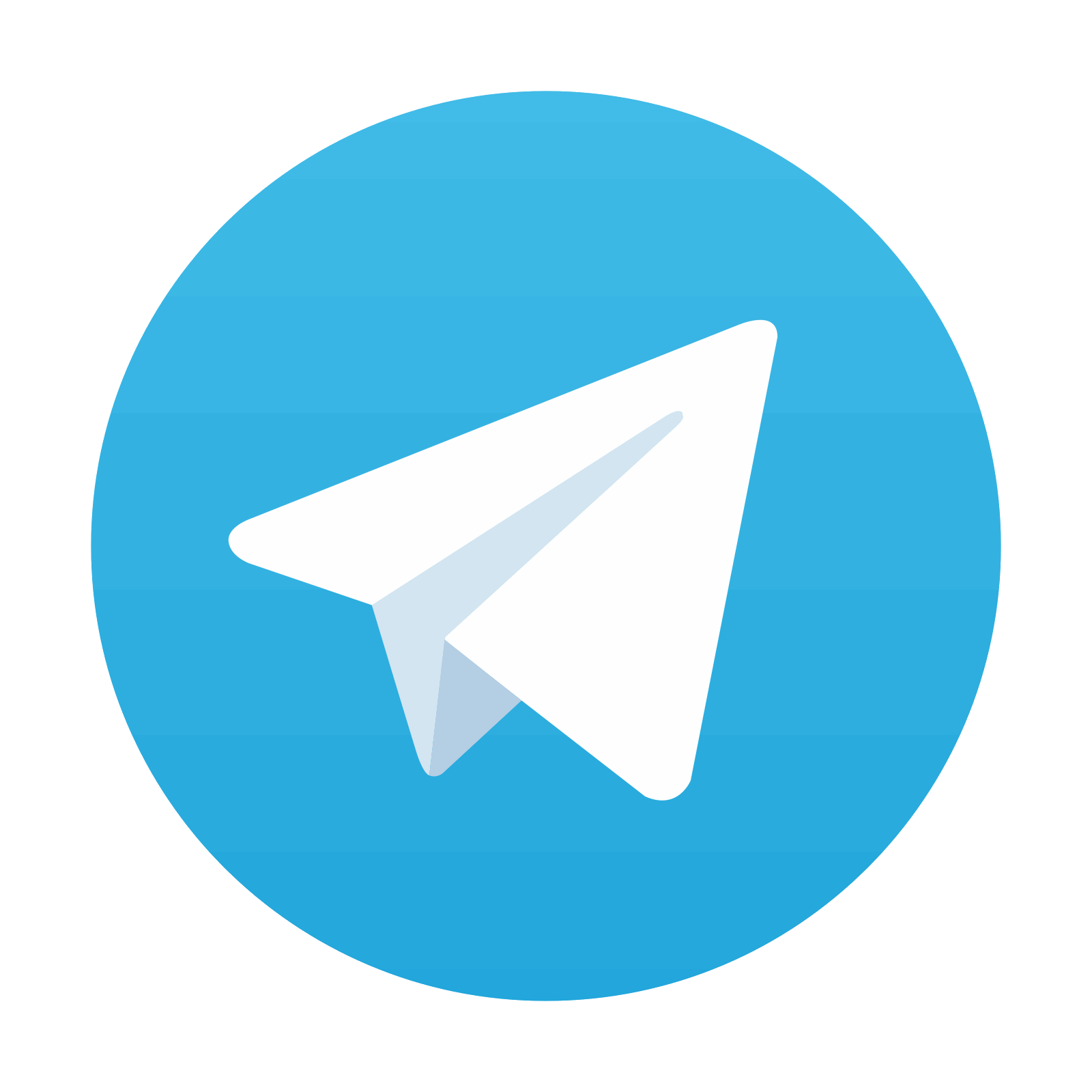
Stay updated, free articles. Join our Telegram channel

Full access? Get Clinical Tree
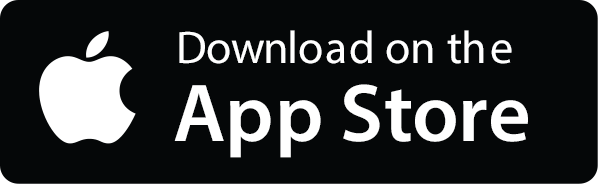
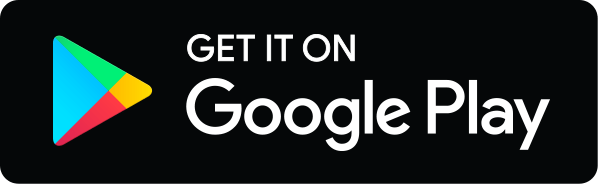