Introduction
Over the past 80 years, the role of laboratory investigation in rheumatic diseases has progressed from reports of curious in vitro phenomena in diseases defined purely on clinical grounds to sophisticated immunopathology testing that provides information now essential for disease diagnosis. A corollary of this progress is that rheumatology practice has increasingly involved the clinical interpretation of an expanding array of immunopathology tests. To be proficient at this requires two things: a basic understanding of the statistical concepts underlying diagnostic testing and an understanding of the tests themselves.
The statistical concepts commonly referred to in diagnostic testing are sensitivity, specificity, and positive and negative predictive values (see Chapter 6 ). The sensitivity of a test is defined as the proportion of people with disease (true positives) correctly identified by the test. The specificity of a test is defined as the proportion of people without the disease (true negatives) correctly identified by the test. These are fixed characteristics of a test related to its performance in a population where disease status is known and the test result is in question. The positive and negative predictive values of a test are the probability of the disease being present or absent given a positive or negative test, respectively. These deal with the “real life” situation in which the test result is known and the disease status is in question. They are not fixed test characteristics and are heavily influenced by the prevalence of the condition in the test population. In mathematical terms this can be thought of as the probability of the disease being present before testing or the “pretest” probability of disease. Increasing the prevalence or pretest probability of disease in a sample population increases the positive predictive value of a diagnostic test, as demonstrated in eFig. 10-1 . In clinical medicine, this is best achieved by testing only those with a significant likelihood of having the disease based on thorough clinical assessment informed by meticulous attention to history taking, physical examination, and a detailed knowledge of its clinical features.

The second requirement for proficient interpretation of laboratory investigations is a basic knowledge of the assays used and an understanding of the basis and characteristics of the tests themselves, including their strengths, weaknesses, and clinical associations. This chapter will concentrate primarily on immunopathology testing, providing a brief overview of assays commonly used in the immunopathology laboratory and then will review immunopathology tests frequently encountered in rheumatology practice. It will also cover the laboratory assessment of the acute phase response, synovial fluid and urine, and briefly review key issues in diagnostic genetic testing in the evaluation of rheumatic diseases.
Laboratory Methods
Immunofluorescence
The use of immunofluorescence (IF) microscopy for the detection of antibodies in patient serum was one of the first techniques developed in immunopathology. The principle of the test is straightforward. A substrate is mounted on a glass slide and antibodies labeled with a fluorescent tag are used to identify the presence of either antigen in the substrate (direct IF) or of antibody on the substrate (indirect IF) using a fluorescence microscope ( eFig. 10-2 ). Direct IF requires fluorescent-tagged antibodies of known specificity, whereas indirect IF requires fluorescent-tagged antibodies to human immunoglobulin (Ig). The substrate used in either technique can be tissue sections, cultured cells, or even microorganisms (e.g., Crithidia luciliae ). IF has several disadvantages; the most important of these are as follows: it is time consuming, labor intensive, requires skilled operators, and has a subjective component that may cause significant variation in results. For these reasons there has been interest in trying to automate the process.

Enzyme-Linked Immunosorbent Assay
Enzyme-linked immunosorbent assay (ELISA) is a commonly used method for detecting the presence of antibody or antigen in a sample. Although there are multiple forms of ELISA, all rely on high specificity antibody–antigen interactions and the use of an automated reader to detect a color change in wells containing a sample and an enzyme-labeled antibody that causes the color change when mixed with an appropriate substrate ( eFig. 10-3 ). Signal strength (color intensity) is related to the amount of labeled antibody present, which in turn is proportional to the amount of antigen or antibody in the sample. The wells used in this assay are contained on a polystyrene plate in large numbers, allowing for the simultaneous testing of numerous samples.

The most basic form of this assay is the direct ELISA, in which a sample containing an antigen to be detected is coated onto the plate wells and labeled antibody of known specificity is added ( eFig. 10-4, A ). This method is useful if the presence of a particular antigen in a sample is in question.

Indirect ELISA is the most common method and involves adding sample to wells coated with a known antigen. Antibodies in the sample that bind to the antigen are detected using enzyme-labeled antihuman Ig antibodies ( eFig. 10-4, B ). This method is useful if it is the presence of a particular antibody in the sample that is in question.
A third variant is the sandwich (or “capture”) ELISA, in which the plate wells are coated with a “capture” antibody targeting a specified antigen. This method may be used to identify the presence of a specific antigen or antibody using direct and indirect detection methods, respectively ( eFig. 10-4, C, D ). In a direct sandwich ELISA the presence of “captured” sample antigen is detected by the addition of an enzyme-labeled antibody targeting an epitope on the same antigen that does not overlap with that of the capture antibody. In an indirect sandwich ELISA the “captured” antigen is bound by an intermediate-unlabeled antibody, the presence of which is then detected using an enzyme-labeled antihuman antibody. An advantage of this method is that it avoids the potential disruption of conformational epitopes on sample antigen that may occur in indirect ELISA.
A final variant, the inhibition ELISA, utilizes competition for labeled antibody between sample and well wall antigen. The resulting reduction in antibody binding to the well wall is an indirect measure of antigen in the sample ( eFig. 10-4, E ).
The advantages of ELISA methodology are as follows: it is rapid, objective, able to analyze multiple samples simultaneously, and can be fully automated. It can also provide quantitative results and is highly sensitive. Its main disadvantage is that it tends to be less specific than other assays.
Radioimmunoassay
Radioimmunoassay (RIA) is a highly sensitive way to measure the concentration of antigen in a sample. In this assay, a quantity of the antigen of interest is tagged with a radioactive isotope (typically of iodine-125 or iodine-131) and mixed with a known amount of its cognate antibody. Sample is then added and any antigen in the sample matching the radiolabeled antigen will compete for binding to the added antibody—effectively drawing antibody away from the labeled antigen. Bound and unbound antigen are separated, and the amount of radioactivity in the unbound fraction measured. The level of radioactivity in this fraction is proportional to the amount of antigen in the sample. A variant of this, the Farr assay, is used for the detection of high-avidity anti-double stranded DNA (anti-dsDNA) antibodies that have a higher specificity for the diagnosis of systemic lupus erythematosus (SLE) than low avidity antibodies. With the availability of newer methods of antibody identification, RIA techniques have fallen out of favor because of the need to use and dispose of radioactive material.
Immunoblotting (Western Blotting)
In immunoblotting (Western blotting), protein antigens—typically nuclear and cytoplasmic extracts—are separated according to molecular weight by using electrophoresis on a polyacrylamide gel. The separated antigen “bands” are transferred (“blotted”) to a nitrocellulose membrane (or strips) that is then incubated with patient or control serum. Bound antibody is identified by use of labeled antihuman IgG. Antibody present in the patient serum is identified by comparison with the control serum results.
The use of cellular extracts as the source of antigen in immunoblotting has the advantage of not requiring purified antigen for testing, and the separation of proteins by molecular weight allows for the determination of the fine specificities of antibody responses to multisubunit antigens. The disadvantages of this technique are that it is time consuming, relatively expensive, requires the denaturing of proteins prior to gel electrophoresis—and will therefore not detect antibodies against conformational epitopes—and has a poor sensitivity for detecting antibodies against Ro and Scl-70 antigens.
Immunodiffusion and Counterimmunoelectrophoresis
These techniques involve the detection of antibody–antigen binding as a “precipitation band” of immune complexes within a gel, which is most often composed of agar. In immunodiffusion, a known antigen and a sample of patient serum are placed in separate but closely approximated wells in the gel and allowed to passively diffuse toward one another. Radial immunodiffusion is a related technique in which the agar gel incorporates antibody of known specificity and a sample containing antigen is placed in a well within the gel. Antigen diffuses from the sample into the gel and will precipitate as a ring if bound by the gel antibody. The diameter of this ring is a function of the concentration of the antigen in the sample.
The process in counterimmunoelectrophoresis (CIEP) is similar to immunodiffusion. However, the pH of the gel is adjusted such that antigen will diffuse toward the anode and antibody toward the cathode when an electrical field is placed across the gel. The wells are situated so that antigen and antibody will diffuse through each other when the electric field is active. The role of the electric field is to accelerate movement of antigen and antibody and thereby shorten the time to a result.
The materials required for CIEP are relatively inexpensive, and both CIEP and immunodiffusion have high specificity for clinical diagnoses. However, in comparison with other methods (e.g., ELISA) they have a lower sensitivity, their performance and interpretation are more labor intensive and skill dependent, and their turnaround time is longer.
Nephelometry and Turbidimetry
Nephelometry and turbidimetry are techniques designed to measure the turbidity of a fluid sample as a gauge of the amount of particulate matter (e.g., immune complexes) it contains. Turbidimetry measures the amount of light able to pass directly through the sample, whereas nephelometry measures the amount of light scattered by the sample. In both techniques, comparison with standard samples of known turbidity is required. These techniques may be used to identify either antibodies or antigens. Both are automated and relatively simple to perform but are less sensitive than other techniques.
Laser Microbead Arrays
Laser microbead arrays are one of a number of emerging “multiplex” immunodiagnostic techniques that allow the assay of multiple analytes (e.g., autoantibodies) from a single sample. This technique shares some of the principles of ELISA in that it involves the use of labeled “reporter” antibodies to detect the binding of patient antibodies to a known antigen. However, rather than being bound to a well wall on a plastic plate, in the most common form of this assay the antigen is bound to a “microbead” color coded to indicate the antigen it carries, and the “reporter” antibody is labeled with a fluorescent tag rather than an enzyme. Multiple beads bound to different antigens are then mixed with patient serum. Antibodies in the serum will bind to relevant bead-bound antigens, which in turn will be bound by fluorescent-tagged antihuman antibodies. The microbeads are then “read” by lasers of two different wavelengths: one identifying the color of the bead (and hence the antigen it carries) and the other determining the presence or absence of fluorescent-tagged antibody ( Fig. 10-5 ).

The advantages of this method are the number of antibodies that can be measured in parallel, the small volume of sample required, and the speed with which this can be done. The main disadvantage of this method is its reduced sensitivity for some applications.
Tests
Antinuclear Antibodies
The term antinuclear antibodies (ANA) refers to any of a large group of autoantibodies that recognize cellular antigens found predominantly, although not always exclusively, in the cell nucleus. These antibodies are associated with numerous autoimmune diseases, most importantly SLE, but may also be found in infectious diseases, malignancies, and apparently healthy individuals ( eTable 10-1 ). The first observation hinting at the existence of ANA was made in 1948 in a series of patients with SLE. It came in the form of what was termed the LE cell: a bone marrow granulocyte that had apparently ingested nuclear material from another cell. Subsequent investigations in vitro revealed that this phenomenon could be induced in bone marrow from healthy subjects by the addition of plasma from patients with SLE, and that it resulted from an element in the globulin fraction of serum with an affinity for cell nuclei. For several decades, demonstration of LE cells retained a role in the diagnosis of SLE, remaining part of the American College of Rheumatology (ACR) classification criteria for SLE until the 1997 revision.
CONDITION | ANA POSITIVITY (%) | REFERENCES |
---|---|---|
Systemic Autoimmunity | ||
SLE | 97-100 | |
Drug-induced LE * | >99 | |
MCTD | >97 | |
Sjögren’s syndrome | 85 | |
Juvenile SSc | 81-97 | |
JIA (all)/oligoarticular subtype | 39/70-80 | |
JDM | 40-63 | |
Organ Specific Autoimmunity | ||
Autoimmune hepatitis | 44-62 | |
Autoimmune thyroid disease * | 35-45 | |
Infection | ||
EBV † | 44-66 | |
HIV * | 21-23 | |
TB * | 24-33 | |
SBE * | 47 | |
Malignancy | ||
Non-Hodgkin lymphoma * | 26 | |
Epithelial ovarian cancer * | 40 |
Detection.
Indirect immunofluorescence (IIF) was the technique first used to identify the presence of ANA on the nuclei of cells in tissue sections; it remains the gold standard for their detection. Early studies noted that ANA could be detected in any cellular tissue but were most easily shown in those tissues in which the cells were arranged in orderly patterns. For this reason early assays used a variety of tissues in which cells were so arranged, including rat and mouse liver and kidney. The use of these tissues, however, was problematic, as their nuclei were small, did not contain all clinically important antigens—particularly Ro/SSA—and rarely contained mitotic cells required for the expression of some antigens, all of which reduced their sensitivity for the detection of ANA in human disease. Modern IIF techniques use monolayers of cultured human epithelial cells derived from laryngeal carcinoma (HEp2) as the substrate. This cell line addresses many of the shortfalls of rodent tissue, although the detection of Ro/SSA antibodies in some preparations remains poor. For this reason a modified version of these cells, in which the Ro/SSA 60 kDa antigen is hyperexpressed—the HEp-2000 cell line—is used in some laboratories. ELISA and other newer methods, such as multiplex bead-based assays, can also be used for the detection of ANA. As they can only offer testing against a limited number of antigens they have a lower sensitivity for the detection of ANA than IIF and are not currently recommended for ANA screening.
Immunofluorescence patterns.
The cell nucleus contains thousands of antigens, any one of which could theoretically be the target of an ANA. In SLE alone, more than 100 different ANA-target antigens have been reported. The pattern of immunofluorescence produced by ANA in IIF assays is determined in large part by the location of the target antigen within the cell. A recent attempt to harmonize the nomenclature used to describe ANA IF patterns on HEp2 cells divides them into five broad groups based on the location of the target antigen. Within each of these groups there are between two and nine descriptors based on the immunofluorescent appearance. In this nomenclature, any antibody that binds to HEp2 cells is considered to be an “ANA,” although some of the responsible antigens are located exclusively in the cytoplasm (e.g., mitochondria), and therefore their cognate antibodies are not strictly “antinuclear.” Many laboratories report these cytoplasmic patterns as an addendum in reports of ANA assays on HEp2 cells. eTable 10-2 outlines the proposed nomenclature along with the disease association of each pattern and the responsible target antigen. Updated consensus recommendations regarding the assessment of autoantibodies using HEp2 cells take a slightly different approach by dividing the patterns according to those commonly and less commonly seen but the pattern descriptors themselves and their associations are largely the same. Figure 10-6 demonstrates some of the more common IIF patterns seen in clinical practice.

PATTERN TYPE | IF DESCRIPTION | DISEASE ASSOCIATION | TYPICAL TARGET ANTIGEN |
---|---|---|---|
Membranous Nuclear | Smooth membranous nuclear | SLE, Sjögren’s | Lamins A, B, C Other membrane proteins |
Punctate membranous nuclear | PBC | Pore complex proteins | |
Nucleoplasmic | Homogeneous | SLE, DIL, JIA | dsDNA, histones, nucleosome, chromatin |
Large speckled | MCTD | hnRNP, U1-RNP | |
Coarse speckled | SLE, MCTD | Spliceosomes, Sm | |
Fine speckled | Sjögren’s | Ro/SSA, La/SSB, Mi-2, Ku | |
Fine grainy Scl-70-like | SSc | Topoisomerase I | |
Pleomorphic speckled (PCNA) | SLE | 34 kDa auxiliary protein of DNA polymerase | |
Centromere | Limited SSc Sjögren’s, PBC | CENP-A,B,C,D,E | |
Multiple nuclear dots | PBC | PML protein, Sp100 | |
Coiled bodies (few nuclear dots) | Sjögrens, SLE, SSc, Raynaud’s | P80 coilin protein | |
Nucleolar | Homogenous | SSc, SLE, Raynaud’s | 37 kDa phosphoprotein B23, PM-scl protein complex, Th/7-2 and To/8-2 RNA associated proteins |
Clumpy | SSc | U3-snRNP (fibrillarin) | |
Punctate | SSc | RNAP I | |
Spindle Apparatus | Centriole (centrosome) | SSc, Raynaud’s | Pericentrin, ninein, Cep250 |
Spindle Pole (NuMa) (MSA-1) | Rarely seen | Centrophilin | |
Spindle Fibre | ?SLE | HsEg5 | |
Midbody (MSA-2) | SSc, Raynaud’s | MSA-2 antigen | |
CENP-F (MSA-3) | Malignancy | MSA-3 antigen | |
Cytoplasmic | Diffuse | Myositis SLE | Amino-acyl-tRNA synthetases (Jo-1, PL-7, PL-12, OJ, EJ, Sc, KS), Ribosomal P0,1,2 |
Fine Speckled | Myositis | Jo-1 | |
Mitochondrial-like | PBC | M2 (mitochondrial membrane proteins) | |
Lysosomal-like | Unknown | Unknown | |
Golgi-like | Sjögren’s, SLE, RA, overlap CT | Giantin | |
Contact proteins | Rare | Microfilamant proteins | |
Vimentin like | Rare | Unknown |
One of the difficulties of the use of ANA IF patterns in the diagnosis of rheumatic diseases is their lack of disease specificity, as evident in eTable 10-2 . This derives largely from the fact that, with few exceptions, the patterns are not specific for a particular antigen; multiple antigens may cause the same pattern and the same antigen may give different patterns in different patients. For this reason, in clinical practice once the presence of one or more ANA have been identified using a screening method, the elucidation of their antigen specificity is generally performed using more targeted assays (see the sections on antibodies to extractible nuclear antigens and anti-DNA antibodies ).
Presence in healthy individuals.
Although a hallmark of many autoimmune diseases, ANA may be found at low titers (e.g., 1 : 40) in up to 32% of healthy adults and at higher titers (e.g., 1 : 160) in 5%. The titer of ANA required before a sample is considered “positive” varies between laboratories and depends on factors such as the technique and materials used. For IIF, titer cut points of 1 : 80 or 1 : 160 are commonly used. An increased prevalence of ANA in the adult population has been noted in women, the elderly, and in first-degree relatives of those with autoimmune disease. In healthy children, ANA have a prevalence of between 5% and 18%. Most of these are at titers in the range of 1 : 80 to 1 : 320; however, higher titers may occur. In general, they do not have specificity against antigens associated with autoimmune disease, and over time a proportion will decrease in titer or disappear completely. Some may be related to intercurrent infection, which can induce transient autoantibodies. Nonetheless a significant number will persist, raising concern about the possibility of autoimmune disease in evolution; studies in adults have found that circulating autoantibodies may be found several years before symptom onset in SLE ( eFig. 10-7 ). Reassurance regarding this issue is provided by reports of a combined total of over 50 ANA-positive children referred for assessment in whom rheumatological evaluation did not identify inflammatory or immune-mediated disease. In these reports ANA titers were generally less than or equal to 1 : 320, although titers as high as 1 : 2560 were occasionally seen. Over a follow-up period ranging between 3 and 5 years only one child developed autoimmune disease, and that child had features at presentation that were suspicious for such an outcome.

Antibodies to Extractable Nuclear Antigens
Extractable nuclear antigens (ENA) are a group of over 100 antigens derived from ribonucleohistone and nonhistone proteins that can be extracted from the saline soluble fraction of cells. They are important as they encompass a significant proportion of the antigens to which ANA form in rheumatic diseases; the presence of ANA directed toward these antigens is more concerning for underlying clinically significant autoimmune disease than those for which no antigen specificity can be found. Antibodies against some of these antigens are highly specific for particular conditions (e.g., Scl-70 and systemic sclerosis) or associated with particular clinical phenotypes within a disease group (e.g., the myositis specific autoantibodies; see Chapter 26 ), whereas others may be found in a range of diseases. As with any test, their relevance is ultimately determined by the clinical context in which they occur. The nomenclature of ENAs is confusing, as a standard approach to their naming was not used. Some are named according to their function (e.g., RNP), others according to the name of the patient for which they were first described (e.g., Sm, Ro, La), and still others according to the disease in which they are most commonly found (e.g., Scl-70). eTable 10-3 outlines the major clinically relevant ENAs and the disease associations of ANA directed against them.
EXTRACTABLE NUCLEAR ANTIGEN | COMMENTS ON COGNATE ANTIBODIES |
---|---|
Sm (Smith) |
|
Ro (SSA) |
|
La (SSB) |
|
U1 RNP |
|
Jo-1 (histidyl-tRNA synthetase) |
|
Scl-70 (Topoisomerase-1) |
|
PM-Scl |
|
Detection.
Anti-ENA antibodies may be detected by gel precipitation assays such as double immunodiffusion; CIEP; solid phase methods such as ELISA; Western blot-based techniques such as immunoblotting; and by multiplex assays; each has advantages and disadvantages. Although the overall concordance between many of these is satisfactory, there are differences in their sensitivity and specificity for detection of particular antigens. Reasons for this include differences in the source of antigens used in the assays and loss of conformational determinants of some antigens during their preparation or the running of the assays. As no single method can be guaranteed to detect all relevant anti-ENA antibodies, current recommendations are that two methods be used. Whichever approach is adopted, it is essential for the clinician to be aware of the strengths and weaknesses of locally used methods and, in particular, to follow up with the laboratory if there is a discrepancy between the clinical picture and anti-ENA antibody result.
Anti-DNA Antibodies
Antibodies to DNA are another clinically important group of ANA. They may be directed toward antigens on double-stranded (ds) or single-stranded (ss) DNA, be IgG or IgM in isotype, and be of high or low avidity. Avidity describes the net strength of the interaction between an antibody and antigen and is determined by several factors, including the individual affinity of antibody-binding sites for their specific epitopes, the charge carried by the antibody and antigen, and the actual physical interaction between the two. High-avidity IgG anti-dsDNA antibodies are the most clinically useful of this group as they have a high specificity for SLE (97.1% in children ) and are associated with an increased risk of development of lupus nephritis in adults, although this association was not confirmed in children in a large cohort study. They are present in 60% to 90% of adults and 72% to 93% of pediatric SLE patients, making them a more sensitive marker of this disease than anti-Sm antibodies, which have a high specificity but low sensitivity for the diagnosis of SLE ( eTable 10-3 ). In addition, antibodies to dsDNA are one of the few autoantibodies whose levels fluctuate with disease activity; rising levels are frequently associated with disease flares. Although high-avidity antibodies are relatively specific for SLE, lower-avidity anti-dsDNA antibodies may be found in a range of other conditions including Sjögren’s syndrome, rheumatoid arthritis, and autoimmune hepatitis. Antibodies to ssDNA have little diagnostic specificity and are rarely used in clinical practice.
Detection.
One of the principal determinants of the avidity of the antibodies detected in laboratory testing is the assay used. Those available for the detection of anti-dsDNA antibodies include radioimmunoassay (i.e., Farr assay), IF assay using Crithidia luciliae (a flagellate parasite containing circular dsDNA without other nuclear antigens in the kinetoplast), ELISA, and multiplex assays. Of these, Crithidia luciliae and Farr assay detect higher-avidity antibodies, with the greatest specificity for the diagnosis of SLE. Most laboratories, however, use ELISA or multiplex assays, which are less labor intensive, faster, more sensitive, and do not involve the use of radioactive materials. However, they detect lower-avidity antibodies with less specificity for the diagnosis of SLE.
Antinucleosome Antibodies
Chromatin is the main form in which DNA is found within the nucleus. At its most basic level it comprises negatively charged DNA wound tightly around positively charged histone protein octamers in conjunction with a linker histone protein in a repeating pattern that gives chromatin a “beads on a string” appearance on electron microscopy. These macromolecular “bead” subunits are known as nucleosomes and can be the target of ANA. These may be directed toward epitopes on the individual components of the nucleosome (i.e., dsDNA and histones) or to conformational epitopes derived from interactions between these components on the intact subunits only (so-called nucleosome-restricted autoantibodies). In the past 10 years there has been interest in the diagnostic use of antinucleosome antibodies (ANuAs) for the diagnosis of SLE. In adults, ANuAs have similar specificity for the diagnosis of SLE as anti-dsDNA antibodies but greater sensitivity. Although it has been suggested that in the future ANuAs may supplant anti-dsDNA testing in the diagnosis of SLE, their acceptance as a valid diagnostic test is not universal.
Antineutrophil Cytoplasmic Antibodies
Antibodies directed toward antigens in the cytoplasm of neutrophils were first described in 1982 in a series of eight patients with a generalized illness in which necrotizing glomerulonephritis and severe arthralgia or myalgia occurred in all and respiratory symptoms occurred in half—some with pulmonary hemorrhage. These are now recognized as common clinical findings in a group of conditions characterized by predominantly small vessel inflammation and the presence of antibodies directed toward specific neutrophil cytoplasmic antigens—the antineutrophil cytoplasmic antibody (ANCA)-associated vasculitidies (AAVs). The AAVs encompass the following clinical entities: granulomatosis with polyangiitis (GPA, previously known as Wegener granulomatosis); microscopic polyangiitis (MPA); eosinophillic granulomatosis with polyangiitis (EGPA, previously known as Churg–Strauss syndrome), and idiopathic necrotizing crescentic glomerulonephritis (INCGN). As with antinuclear antibodies, the term ANCA does not refer to a single antibody but to a family of antibodies, each directed toward a specific antigen. In the case of ANCA, these antigens are contained within granules in the neutrophil cytoplasm and include proteinase 3 (PR3); myeloperoxidase (MPO); lactoferrin; elastase; cathespin G; and bactericidal/permeability-increasing protein, among others. ANCA directed toward PR3 and MPO are the most clinically relevant as they are strongly associated with the AAVs.
Detection.
IIF was the method by which ANCA were first identified and remains the recommended method for their initial detection. Ethanol-fixed neutrophils are the substrate used and in the presence of ANCA, one of two main IF patterns will be observed: predominant cytoplasmic fluorescence with central interlobular accentuation (c-ANCA) or perinuclear fluorescence, frequently with nuclear extension (p-ANCA) ( Fig. 10-8 ). The p-ANCA pattern is actually an artifact caused by movement of the relevant antigens, which are distributed throughout the cytoplasm in vivo, to a perinuclear position during ethanol fixation. This redistribution does not occur with formalin fixation and may be difficult to discern if ANA are also present. Patterns lacking the typical fluorescent features of c- or p-ANCA (atypical ANCA) may also occur.

As with ANA, the IIF pattern of ANCA provides some guidance regarding their possible antigen specificity. In patients with AAVs, c-ANCA and p-ANCA patterns are generally associated with antibody specificity for PR3 and MPO, respectively, although occasionally the reverse is seen. In unselected patients, these associations are not as strong, particularly for p-ANCA and atypical ANCA, which may occur with ANCA directed toward a number of antigens other than MPO. A positive test for ANCA on IIF therefore requires further assessment to determine the antigen specificity of the antibodies. ELISA assays are commonly used in this situation. Sandwich ELISA and a related assay, the anchor ELISA—in which a protein rather than antibody binds the antigen to the well—may have particular clinical utility in this setting, offering increased sensitivity without loss of specificity. Bead-based multiplex assays have also been developed for this purpose.
Disease associations.
ANCA are the serological hallmark of active AAVs. They are found in approximately 90% of adult patients with active GPA, 75% with MPA, and 30% with EGPA. The prevalence of ANCA during periods of remission or in localized forms of GPA is lower. In GPA the typical finding is that of a PR3-specific c-ANCA with just 10% to 25% having an MPO-specific p-ANCA. This pattern is the converse of that seen in the other AAVs in which PR3-specific c-ANCA is the less common specificity. The prevalence and pattern of specificities of ANCA in pediatric GPA is similar to that in adult disease. As with anti-dsDNA antibodies, ANCA titers tend to fluctuate with disease activity. Although some studies have shown them to be useful in predicting disease flares, a recent meta-analysis found their predictive level to be only modest.
ANCA may be found in a range of conditions other than AAVs, though their low prevalence and lack of correlation with clinical phenotype mean they have little value for their diagnosis. These conditions include inflammatory bowel disease, autoimmune hepatitis, antiglomerular basement membrane disease, rheumatoid arthritis, juvenile idiopathic arthritis, and various infectious diseases, particularly tuberculosis and hepatitis C. Drugs may also induce the formation of ANCA and in some cases lead to the development of AAVs. In general, the ANCA identified in these conditions demonstrate atypical features on IIF, usually with a p-ANCA pattern, and have specificity for antigens other than PR3 or MPO. However, this cannot be relied upon as a distinguishing feature from AAV, as PR3- or MPO-specific ANCA may also occur.
Antiglomerular Basement Membrane Antibodies
Antibodies directed toward the glomerular basement membrane (GBM) were first shown to be pathogenic in humans in 1967. In most cases these antibodies are of the IgG1 subclass and are directed toward the noncollagenous (NC1) domain of the α3 chain of type IV collagen (α3[IV]NC1), found in the kidney basement membrane, lungs, cochlea, and retina. Collectively, the clinical syndromes with which they are associated are termed anti-GBM disease, and they consist of the following: isolated rapidly progressive glomerulonephritis (RPGN); pulmonary hemorrhage or the combination of the two—eponymously known as Goodpasture syndrome. By definition, anti-GBM antibodies can be detected in 100% of patients with anti-GBM disease using a combination of serum and tissue diagnostics. Unlike many autoantibodies, they are not detectable in the serum of healthy subjects, although there are reports of their isolation using specialized techniques for identifying low levels of low-avidity antibodies. They may, however, be detectable using standard assays in the context of polyclonal B cell activation in human immunodeficiency virus (HIV) and hepatitis C infection without evidence of pulmonary or renal disease. They can also be found in up to 10% of patients with AAVs, and may portend a more guarded renal prognosis.
Detection.
Although anti-GBM antibodies can be identified by IIF using normal human or primate kidney as substrate, this method is relatively insensitive—as low as 60% for those with isolated RPGN—and is rarely used. The most commonly used method is ELISA, using bovine or recombinant human α3(IV)NC1. Multiple commercial ELISA kits are available for this purpose, with sensitivities and specificities greater than 90%. It is important to be aware, however, that up to 5% of patients with anti-GBM disease will not have circulating antibodies that can be detected by using commercial assays. Reasons for this include the disruption of conformational epitopes during assay preparation; differences in the epitopes recognized by the antibodies of some patients, rendering them unreactive with bovine preparations; and the predominance of IgG4 subclass or other Ig isotype (e.g., IgA or IgM) antibodies in some cases. Demonstration of antibodies in situ on the glomerular basement membrane by direct immunofluorescence is considered the diagnostic gold standard, and, where practical, a renal biopsy is recommended in all patients in whom the diagnosis of anti-GBM disease is suspected.
Rheumatoid Factor
The report in 1940 of an “agglutination activating factor” in serum from patients with rheumatoid arthritis (RA) that caused clumping of sheep red blood cells (RBCs) sensitized with rabbit anti-sheep corpuscle serum was the first description of the effect of antibodies in RA subsequently known as rheumatoid factor (RF). RF is not a single antibody but a family of antibodies directed toward the Fc portion of IgG. They may be of either low or high affinity. Low-affinity RFs are IgM antibodies that play a role in the clearance, B-cell uptake and complement fixation of immune complexes in the normal immune system and may be seen in the context of infection and in otherwise healthy subjects. High-affinity RF can be of any isotype and is frequently associated with autoimmune disease. High-affinity IgM-RF is the most clinically significant, being the predominant isotype in both RA and the RF-positive polyarticular subtype of juvenile idiopathic arthritis (JIA), and the only type considered in the current classification criteria for these two conditions. The presence of IgM-RF in both is associated with an increased risk of progressive disease, joint damage, and disability. In RA, it is also associated with a greater risk of extraarticular disease. Similar associations have been reported for IgA-RF in both adults and children. Other conditions in which IgM-RF may be found include other rheumatic diseases, notably Sjögren’s syndrome—in which titers of RF are typically very high—and infections, particularly if chronic, secondary, or latent ( eTable 10-4 ).
CONDITION | RHEUMATOID FACTOR POSITIVITY (%) | REFERENCES |
---|---|---|
Rheumatic Diseases | ||
Mixed cryoglobulinemia (MC) | 100 † | |
Rheumatoid arthritis | 66-92 | |
Juvenile idiopathic arthritis * | 2-7 | |
Sjögren’s syndrome * | 46-75 | |
Mixed connective tissue disease * | 57 | |
Granulomatosis with polyangiitis * | 52 | |
Systemic lupus erythematosus * | 14 | |
Infection | ||
Chagas disease (acute/chronic phase) | >90/25 | |
Subacute bacterial endocarditis | 35-68 | |
Leprosy | 53-60 | |
Hepatitis C | 38-76 | |
Epstein–Barr virus | 23 | |
Healthy Subjects | ||
Adults (20-100 years) | 4% | |
Children | 3-8% |
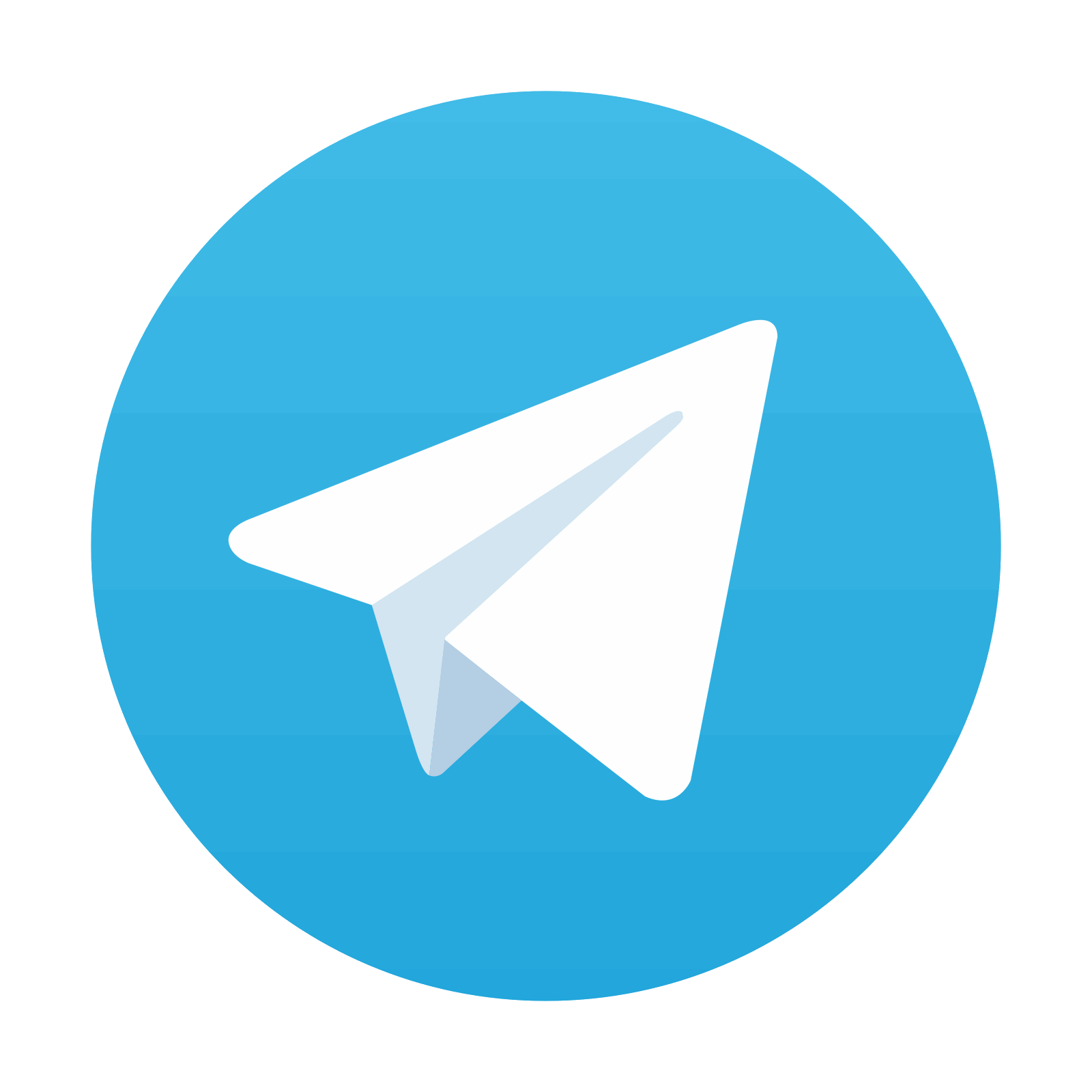
Stay updated, free articles. Join our Telegram channel

Full access? Get Clinical Tree
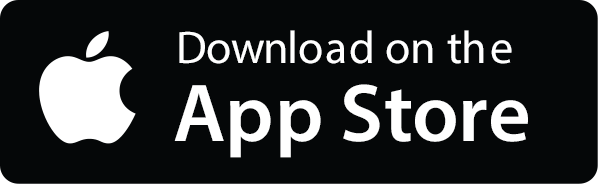
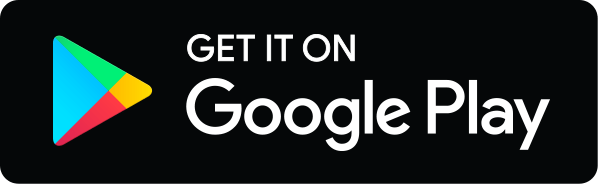
