Knee Kinematics Following Total Knee Arthroplasty
Michael T. LaCour, PhD
Milad Khasian, MS
Douglas A. Dennis, MD
Richard D. Komistek, PhD
Following the logic of the “Forgotten Knee,” a common goal of total knee arthroplasty (TKA) is to restore joint functionality as closely as possible to that of a healthy, nonimplanted knee, such that the implant effectively restores overall function to the point where the patient completely forgets they have a replaced knee.1,2,3 In an effort to quantify and analyze joint performance to improve overall TKA designs, substantial research has been done to better understand joint mechanics. This research is commonly done under the assumption that “if components are moving correctly, then the device must be performing well.”3
Simply determining the motion (kinematics) of a TKA is the easy part, as this is just a matter of utilizing proper imaging techniques to extract the desired kinematic parameters. Determining if the components are moving “correctly” is often a debated topic. However, the general consensus is that the motions of a knee following a TKA procedure should closely mimic those of the healthy, nonimplanted knee.3,4
OVERVIEW OF KNEE KINEMATICS
A brief overview of the kinematics of the knee will be provided in this section.
A properly constrained knee has three independent degrees of freedom, two rotational and one translational, yielding flexion and extension about the medial/lateral axis, internal and external rotation about the superior/inferior axis, and translational sliding in the anterior and posterior directions. Under certain conditions, it is also possible for the femur to rotate about the anterior/posterior axis, demonstrating a phenomenon of condylar liftoff,5,6,7 but this is generally considered abnormal. Other forms of motion of the knee joint are also possible, but these are generally negligible compared to the aforementioned three.
Due to soft-tissue constraints in combination with the bone geometry of the knee, it is generally a safe assumption that the normal knee actually functions as a one degree-of-freedom joint (knee flexion/extension), driven by the quadriceps and hamstring muscle groups, and that other motions are dependent on knee flexion.3 More specifically, at full extension and in early flexion, the femur is generally internally rotated with respect to the tibia.8,9,10 As the knee begins to flex (such as with a knee bend or squat activity), the femur begins to rotate externally with respect to the tibia.4,7,11,12,13,14,15,16 Throughout flexion, both condyles of the femur generally roll or slide posteriorly atop the tibia.3,7,10,12 However, the lateral condyle moves more posteriorly than the medial condyle, resulting in more of a pivoting pattern about the medial condyle with a rollback pattern of the lateral condyle, which directly correlates to the external rotation pattern of the femur. In deep flexion, it is possible for both condyles to roll so far posteriorly that they can roll off the edge of the tibial plateau.
The patella bone plays an integral role in transmitting the forces of the quadriceps muscles by increasing the moment arms of the extensor forces and creating a direct link between the quadriceps and the tibial tuberosity. Hence, the patellofemoral joint is an integral part of the knee. Throughout flexion, the patella generally remains in contact with the femur.17,18 In early- and mid-flexion, there is a single region of contact between the femur and patella as the patella tracks within the trochlear groove of the femur. At deeper angles of flexion (greater than 90°), the patella begins to track between the femoral condyles, dividing the contact area into two separate regions. In general, patellar flexion increases with increasing knee flexion,17,19,20,21,22,23 but it does so at a lower rate than the femur relative to tibia.
While kinematic evaluations of the nonimplanted knee commonly document posterior translation of the femoral condyles and external rotation of the femur with respect to the tibia with increasing knee flexion, the kinematic patterns of patients having a TKA can vary considerably. Common differences include reduced posterior translation of the femur, paradoxical anterior sliding of the femur, reverse axial rotation patterns of the femur with increasing flexion, occurrences of femoral condylar liftoff, and abnormal patellofemoral mechanics.4,7,11,24,25,26,27
KINEMATIC ANALYSIS TECHNIQUES
Many different methods have been used to analyze the kinematics of the normal knee and the knee after implantation of total knee arthroplasty. These have included
roentgen stereophotogrammetric analyses,28,29,30,31,32,33 in vitro cadaveric evaluations,34,35,36 quasi-dynamic magnetic resonance imaging testing,13,37,38 noninvasive laboratory motion analysis systems using marker tracking,14,39,40,41,42,43 and in vivo video fluoroscopy.5,26,44,45,46,47,48,49 While each of these techniques certainly is valuable in its own way, it is essential to recognize both the advantages and disadvantages of each technique to accurately interpret the results of a specific analysis.
roentgen stereophotogrammetric analyses,28,29,30,31,32,33 in vitro cadaveric evaluations,34,35,36 quasi-dynamic magnetic resonance imaging testing,13,37,38 noninvasive laboratory motion analysis systems using marker tracking,14,39,40,41,42,43 and in vivo video fluoroscopy.5,26,44,45,46,47,48,49 While each of these techniques certainly is valuable in its own way, it is essential to recognize both the advantages and disadvantages of each technique to accurately interpret the results of a specific analysis.
For example, roentgen stereophotogrammetric analyses, although generally highly accurate and precise,28,29 are also highly invasive and are commonly performed under non-weight-bearing conditions and are quasi-dynamic.30,31,32,33 Cadaveric studies allow for up-close observation of a physical specimen, but they often do not simulate in vivo conditions because the actuators used to apply joint loads are unable to accurately reproduce in vivo motions. MRI testing is conducted under static, non-weight-bearing conditions, which does not represent what is happening during daily dynamic activity. Additionally, while video marker systems excel in capturing high-speed multibody movement, error analyses of video marker evaluations have suggested these systems can induce significant out-of-plane rotational and translational error, owing to motion between skin markers and underlying osseous structures.14,50,51 Finally, video fluoroscopy (single-plane and biplanar) and 3D model-fitting techniques have the advantage of being highly accurate when testing under in vivo, weight-bearing, fully dynamic conditions, but the subjects generally must perform slower-speed activities.5,44,45,46,47,48,49,52
![]() FIGURE 7-1 Subject performing a deep knee bend (A) and normal gait (B) while under fluoroscopic surveillance. |
This chapter summarizes various in vivo kinematic analyses of multiple groups of patients implanted with various designs of fixed- and mobile-bearing TKA and compares their in vivo knee kinematic patterns to those studies of the normal knee. The specific results found herein were collected using video fluoroscopy and three-dimensional-to-two-dimensional (3D-to-2D) registration techniques. Therefore, more specific information on the employed fluoroscopic analysis techniques is presented below.
FLUOROSCOPIC ANALYSIS METHODS
During fluoroscopic study data collection, subjects perform various activities, such as deep knee bend, gait, chair rise, step up, step down, ramps, leg swings, and more, while the subject’s joint is under fluoroscopic surveillance (Fig. 7-1). Fluoroscopic studies of the knee are generally conducted in the sagittal plane. Upon completion of the activities, specific frames of interest (for example, specific increments of flexion or specific phases of the gait cycle) are extracted from the fluoroscopic video and exported into preprocessing software for further analysis. Depending on the type of fluoroscopy unit used, the images may experience distortion, which must be corrected. After the images have been properly processed, they can be exported to a model-fitting software program for 3D-to-2D registration.53,54,55
A more recent advancement in fluoroscopic studies is the implementation of robotic tracking fluoroscope system (TFS) units. These units have automated control systems
that (1) allow the entire unit to follow a patient around a room, and (2) allow the x-ray source and detector to move independently from the rest of the unit to keep the knee (or any joint of interest) in the center of the fluoroscopy video. These robotic units allow for fluoroscopic evaluations of more complex activities, such as ramp activities, several stair steps in succession, and multiple gait cycles, all at more natural speeds.56 Although fluoroscopic studies with TFS units are relatively new, it is hypothesized that these TFS studies will allow patients to conduct activities in a much more natural, unconstrained manner versus traditional C-arm fluoroscopy units, thereby yielding results that more accurately represent a patient’s daily activities.53,56
that (1) allow the entire unit to follow a patient around a room, and (2) allow the x-ray source and detector to move independently from the rest of the unit to keep the knee (or any joint of interest) in the center of the fluoroscopy video. These robotic units allow for fluoroscopic evaluations of more complex activities, such as ramp activities, several stair steps in succession, and multiple gait cycles, all at more natural speeds.56 Although fluoroscopic studies with TFS units are relatively new, it is hypothesized that these TFS studies will allow patients to conduct activities in a much more natural, unconstrained manner versus traditional C-arm fluoroscopy units, thereby yielding results that more accurately represent a patient’s daily activities.53,56
Fluoroscopic analysis and 3D-to-2D registration techniques can be utilized on both implanted and nonimplanted knee images. For implanted knees, the component CAD models must be used. For nonimplanted knees, the bone models must be created from CT, MRI, or other imaging methods. In either case, to find the orientation of a desired knee “component” (implanted CAD model or nonimplanted bone model) from an x-ray image, the fluoroscopic space is virtually modeled within a specialized computer program. This program allows the user to virtually recreate the fluoroscopic space between the x-ray source and the image intensifier, and the models of the knee components are virtually placed in the space between the camera and the image (Fig. 7-2). This allows the user to superimpose (overlay) the CAD model silhouettes on top of the implant component silhouettes from the x-ray image. By matching a 3D model of the knee component to each fluoroscopic frame of interest from the video, the 3D in vivo kinematics can be extracted by interpolating between multiple 2D images (Fig. 7-3).53
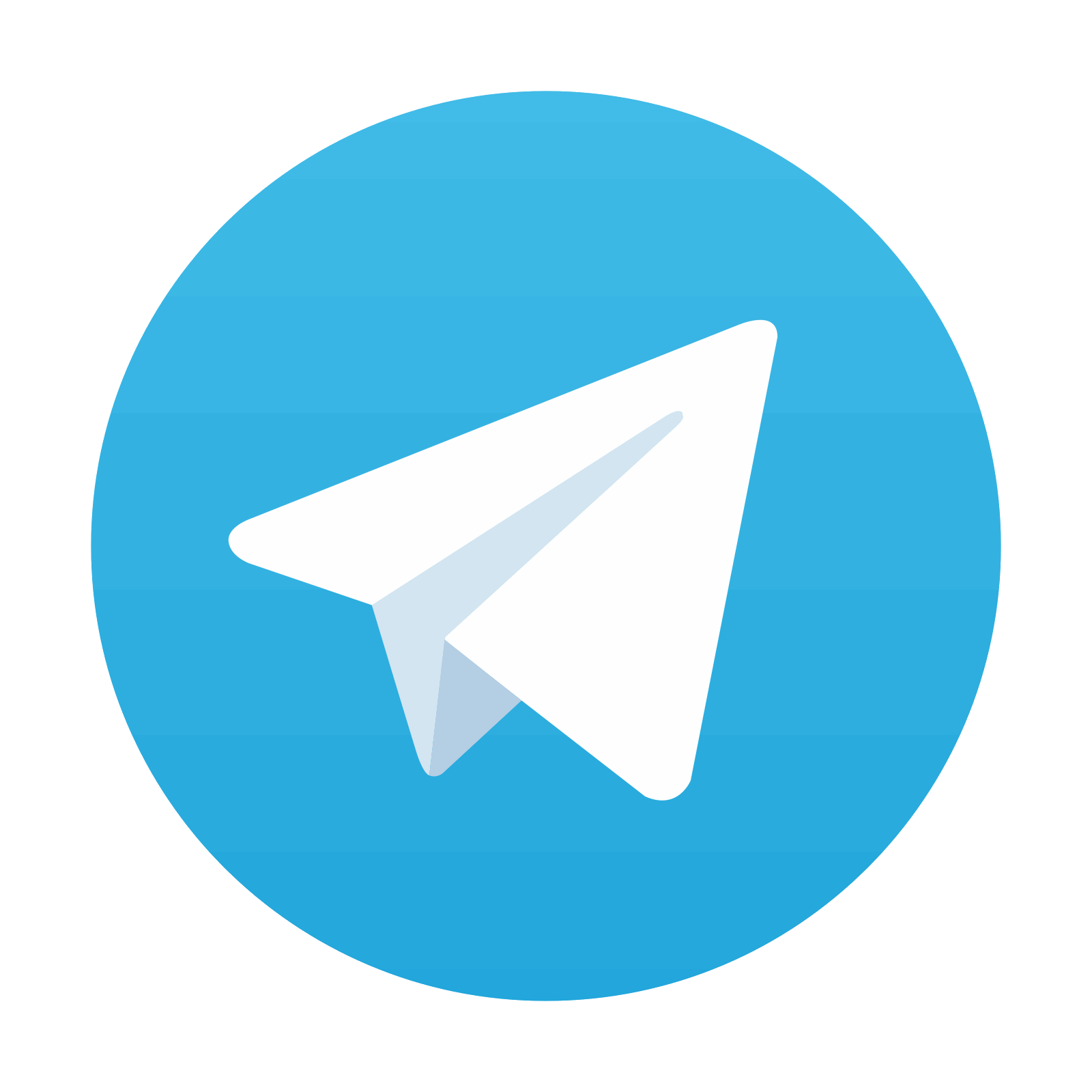
Stay updated, free articles. Join our Telegram channel

Full access? Get Clinical Tree
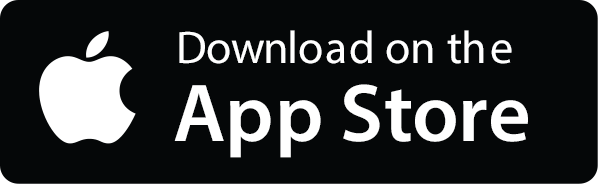
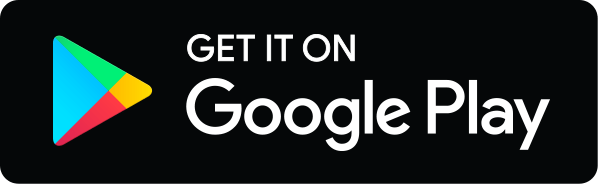