Key Points
- •
Robotics is taking an increasingly larger role in the delivery of health care.
- •
The field supports all phases of medicine from acute care to rehabilitation.
- •
This technology is making battlefield medicine safer and more efficient.
- •
This industry is growing and changing the economics of health care.
- •
With the advent of artificial intelligence and other advancements, robots can offer increasingly more sophisticated capabilities for direct patient care and for health systems infrastructure.
The advancement of technology has grown exponentially in the commercial and public sectors over the last several decades, with health care being no exception. Robotics, in particular, serves as a pivotal tool in improving the delivery of high-quality, personalized health care in the realm of battlefield medicine, rehabilitation therapy, and functional assistance using exoskeletons and orthoses.
Robot-assisted rehabilitation can complement conventional therapy methods by increasing the amount of time patients can participate in therapeutic exercises, reducing burden on therapists, improving secondary health conditions, and personalizing the recovery process. The MIT Manu, one of the first robotic devices designed for neurorehabilitation, was shown to improve overall function in the upper extremity following stroke by guiding patients to trace patterns on a screen with an end-effector device. Since then, several functional robotic devices for upper and lower extremity training have been introduced to the market, which similarly work through guided facilitation of movement and sensorimotor functional training. These include, but are not limited to, wearable exoskeletons, grounded exoskeletons, and end-effector devices. Robotics supports repetitive exercises for motor relearning, which encourages plasticity while also reducing therapist burden, and these machines may be a useful tool in telerehabilitation for patients who cannot access traditional rehabilitation facilities. In a study following patients with spinal cord injury of C5 or lower, the use of a lower extremity exoskeleton was shown to reduce spasticity, as measured by patients’ own subjective reports as well as the Modified Ashworth Scale. Furthermore, use of the Indego exoskeleton, which was comprised of hip, knee, and bilateral ankle-foot orthoses units, decreased patients’ Borg Scale of Perceived Exertion while walking indoors.
In this chapter, we will explore how the growing field of robotics in rehabilitation has affected both practitioners and patients, examine its role in acute and battlefield medicine, as well as take a look at the major leaders in the industry and future developments in the area, including artificial intelligence.
How Robotics will Affect the Practice of Medicine for Practitioners
The use of robotics serves as a complementary tool to traditional therapy methods, the delivery of which is often limited by time, cost, and availability of staff. For example, early mobilization has been widely accepted as a method for improving outcomes and functional mobility for critically ill patients in the intensive care unit. In a study examining mild patients with to moderate intracranial hemorrhage, early out-of-bed mobilization was seen to be beneficial in improving functional independence measure motor scores and reducing length of stay in the stroke center compared with those patients who received only in-bed rehabilitation. Unfortunately, mobilizing every patient may not be possible due to lack of trained personnel. Robotics such as exoskeleton-assisted walking devices can help assist with this often-difficult task. Studies have shown that exoskeletons can contribute to significant health benefits, such as cardiovascular health, bowel function, bone health, and quality of life, by aiding patients with spinal cord injury with early mobilization.
Although acute rehabilitation can offer patients in-person therapy 3 hours a day, patients often spend the rest of the day inactive, despite being encouraged to continue self-directed exercises taught by their therapists. Robotics may serve as a useful adjuvant to in-person therapy by encouraging additional mobility and may even be utilized to increase therapy time in group settings through robotic-assisted group training. In doing so, robotics can improve provider productivity and decrease delays to initiation of rehabilitation. Studies have shown in poststroke populations, robotic-assisted therapy is comparable in improving upper limb functional outcomes to conventional therapy. In a study of patients after stroke, robotic therapy reduced upper limb impairment in patients with both chronic and subacute stroke, with evidence of improvement in several kinematic parameters, including speed. Additionally, robotics can help practitioners monitor improvement during therapy by collecting data throughout recovery. Information such as progress with range of motion, precision, accuracy, and kinematic data across multiple joints can be useful with adjusting the intensity and focus of therapy.
Telerehabilitation is a growing field, especially in the era of the SARS-CoV-2 pandemic, where compliance with in-person therapy may not be feasible or safe. Faced with the challenge of providing accessible care, practitioners are looking for ways to augment traditional routes for delivery of care. Robotics can increase access to therapy for patients in remote areas with limited resources, while also increasing overall therapy time. Specifically, home-based rehabilitation with the use of end effectors, and exoskeletons can be utilized for highly repetitive exercises while also giving the patient a sense of control over their rehabilitation progress in a remotely supervised setting. Robotic upper limb rehabilitation devices designed for in-home use have been shown to be similar in usability and performance compared with their commercial counterparts. In a study of rural veterans following unilateral or ischemic stroke, the use of in-home robotic rehabilitation devices, in conjunction with remote monitoring by a therapist, resulted in an overall increase in upper and lower extremity mobility, as well as perceived independence. In the maintenance step of therapy, commercial gaming systems such as Nintendo and PlayStation have been utilized to encourage continued activity and self-directed therapy.
Overall, robotics can complement conventional therapy by standardizing training environments, increasing access to care, and reducing burden on therapists.
How Robotics will Affect the Experience of Health Care for Patients
In the field of rehabilitation, robotics plays a significant role in neurorehabilitation, functional training, and assistive technology. For patients undergoing rehabilitation, these technological advancements have the potential to not only improve functional outcomes but also provide social and psychological benefits as well.
The incorporation of biorobotics in the field of prosthetics assists patients in experiencing a more natural setting with the use of sensory integration and feedback. Incorporating visual, auditory, and tactile feedback improves user experience through an engaging and stimulating process. The use of biofeedback provides a method through which patients can decrease compensatory movements and work on spatial, cognitive, and visual integrative skills training. Furthermore, the utilization of sensors to monitor position and movement, and respond in real time, aims to mimic a realistic environment. Robotic training can enhance kinematics and improve function through the use of exoskeletons and robotic end effectors. Several studies have shown that utilization of robotic rehabilitation devices can enhance motor outcome after stroke through biofeedback and repetitive task training.
The incorporation of virtual reality and augmented reality in rehabilitation training can also complement traditional therapy. In fact, utilizing a gaming interface may be highly motivational and encourage patients to participate in therapy to improve fine movements to aid in activities of daily living. Robotics are ideal for consistent, repetitive motion training and functional exercises during intensive therapy following central nervous system (CNS) damage, while also being engaging for the patient. Studies have shown that augmentation of therapy with robotics may give patients a sense of control over their progress, thereby improving their sense of independence and mood. Neuromodulation, vibration, and continuous passive motion may be passive modalities that can address spasticity and improve range of motion.
Brain-computer interfaces are an emerging technology with the capability of amplifying and translating brain signals to produce a desired outcome on an external device. Several studies have shown the effectiveness of brain-computer interfaces in motor retraining for CNS injuries, by aiding neuroplasticity and bypassing damaged tissue. With this technology, electrodes can be placed over the cortex to record neural signals, which are then converted to a prosthetic output. In other cases, intracranial implants transmit electroencephalographic signals to external devices. Neurostimulation and cortical activation in both sensory and motor pathways using neuroprosthetics provide a promising future for modulating neuroplasticity. The continuing development of neuroprosthetics will hopefully serve as a tool for patients with neurologic disorders to regain previously lost function.
The Growing Industry of Robotics—Taking a Look at Some of the Major Companies
Therapy Robots
Bionik, the developer of InMotion Arm therapy, has been used in the inpatient setting for several years to improve range of motion, strength, speed, and coordination in the upper arm following stroke and other CNS injuries. Furthermore, this tool can be used to decrease spasticity by activating muscles that otherwise would not be used. According to the developer, patients can achieve a minimum of 600 movements per hour with the assistance of the InMotion arm device. With the success of the inpatient model, Bionik is in the process of developing an in-home device.
Ekso Bionics, pioneers in wearable exoskeletons, has been creating robotics to help with gait training in patients with limited lower extremity mobility or paralysis. EksoGT and EksoNR exoskeletons can allow previously nonambulatory patients to stand and walk and are approved by the Food and Drug Administration for gait training in patients with strokes, traumatic brain injuries, and spinal cord injuries. The company has several products, ranging from load carriers to SmartAssist software for pregait training. A unique feature of this device is its ability to adjust power to either side of the body, thereby making it easier or more difficult for the patient to move the limb.
Hocoma, a rehabilitation technology company out of Switzerland, has added several wearable exoskeletons to the market as well, including its products Erigo, Lokomat, and Andago. These devices aid with early mobilization by improving muscle strength and joint range of motion. Erigo allows for bedbound patients to be slowly verticalized, while simultaneously performing cyclical leg movements with functional electrical stimulation at the lower limb. Small studies have shown this device to be a safe, useful tool for early sensorimotor rehabilitation and vestibular rehabilitation in patients after stroke.
Augmented Feedback
Hocoma also has delved into the realm of augmented feedback therapy with its product Valedo, a wearable sensor. Placed on various points on the low back, the device claims to reduce low back pain by encouraging range of motion, lumbar stabilization, and general movement therapy. The device provides real-time feedback in an encouraging and motivating setting, which has been shown in small studies to be similar to conventional exercise led by physical therapists in terms of adherence, improvement in disability, and movement control.
Prosthetics
Össur Touch Solutions, formerly known as Touch Bionics, is a world leader in prosthetic technologies, manufacturing prosthetics for upper and lower limb amputees. Its products range widely from multiaxial, myoelectric, microprocessor, and high-performance carbon prostheses for amputees of all activity levels. The company manufactures knees, feet, legs, and hands, as well as liners, sockets, and bracing.
Robots in Acute Medicine
Robots in medicine can fulfill a wide range of functions to assist doctors, nurses, and other medical staff and to enhance the delivery of care. Hospital robots can distribute medications and laboratory specimens around the hospital. Pharmacy robots can process, return, and restock medications, helping to reduce costs and errors in hospitals. Robotic systems are also being designed to disinfect devices and equipment in health-care settings.
Aethon has developed a robot, TUG, made specifically for hospitals. It uses a built-in map and sensors to navigate hospital halls and communicates with elevators, fire alarms, and automatic doors via Wi-Fi. TUG can deliver medicine, meals, and supplies through the hospital and to hospital units. It also uses biometric security and pin codes to transport lab specimens safely.
There are three main types of robots currently in use in the surgical field—active, semi-active, and master-slave systems. Active systems work autonomously, while remaining under the control of the surgeon, and undertake preprogrammed tasks. Semi-active systems allow for a surgeon-driven element to complement the preprogrammed element of these robot systems. Master-slave systems (of which the da Vinci and ZEUS platforms were forerunners) lack any of the preprogrammed or autonomous elements of the other systems. They are entirely dependent on surgeon activity. Surgeon hand movements are transmitted to laparoscopic surgical instruments, which reproduce surgeon hand activity inside the patient’s body.
Some of the most used medical robots are artificial intelligence–assisted surgical robots, which can analyze data from preoperative medical records to physically guide a surgeon’s instrument in real time during a procedure. Such surgical robots are used in neurologic, orthopedic, and laparoscopic procedures, being operated both locally and remotely. Robot-assisted operations are often minimally invasive, offering an alternative to open surgeries, which have more associated risks and require a longer period of recovery.
How Robots can Serve in Battlefield Medicine
Approximately 86% of all battlefield deaths occur within the first 30 minutes after injury. Therefore, the ability to rapidly locate, diagnose, and provide appropriate initial treatments are vital to improving the historical outcomes of battlefield injuries. Before attending to the injured, frontline medics must quickly locate the soldier and extract him or her from the battlefield, often under fire. This can take up costly minutes, as well as expose medics as targets.
Military robotic combat casualty care has three main goals: safely extracting the injured soldier from harm’s way, rapidly diagnosing life-threatening injuries, and delivering lifesaving interventions. In the short term, extraction robots will decrease the risk to the soldier and combat medic by safely moving wounded war fighters out of the line of fire. In the longer term, teleoperated and autonomous surgical robots will deliver surgical care on the battlefield as well as during transport to military treatment facilities.
The US military recognized the potential of stationing trauma surgeons remotely, allowing them to tend to the wounded via a robotic platform. This would allow for a timely response while reducing mortality and morbidity of service personnel on the battlefield.
Therefore, the US Department of Defense funded the development of unmanned medical treatment systems for the battlefield. The goal of these “Trauma Pods” was to stabilize injured soldiers within minutes after a battlefield trauma. The trauma pod could assist a surgeon in administering lifesaving medical and surgical care prior to evacuation, as well as during transport. With the help of the trauma pod, a surgeon could conduct all the required surgical procedures from a remote location using a system of surgical manipulators. The system’s actions could then be communicated wirelessly to the surgery site. Automated robotic systems would provide necessary support to the surgeon to conduct all phases of the operation. The Trauma Pod system also included x-ray capabilities for fluoroscopic guidance, if needed, for interventions. The trauma pod system was successfully teleoperated by a surgeon to perform a bowel anastomosis and an iliac artery shunt placement on a phantom patient with no human assistance. However, Trauma Pod never moved into final animal trials, which would have included control of hemorrhage as well as airway and intravenous line insertion, due to a command transition and funding issues from federal budgeting processes.
Telecommunication and robotic limitations that restrict robust intervention at a distance are areas of continued military research and development. As these limitations are overcome, medical robots will provide robust casualty extraction and battlefield care that will save the lives and limbs of our soldiers.
The Defense Advanced Research Projects Agency, or DARPA, founded a prosthetics program in 2006 for Wounded Warrior amputees and military personnel with spinal cord injury. These programs have funded the ongoing research and development of neuroprosthetic technology, such as the LUKE arm, a highly advanced bionic arm with the capability of mimicking dexterous upper limb movements. The LUKE arm is a multi-articulated prosthesis controlled by multiple input devices, including surface electromyography electrodes and wireless foot controls. Furthermore, it is the only commercially available prosthesis with a powered shoulder in addition to 10 other powered joints, with the capability of refining highly specific movements and regulating pressure and sensation.
Artificial Intelligence and Robotics (And Brain-Computer Interface)
Many patients who are seen for rehabilitation have undergone functional changes that require adaptation. The most common example of functional change is seen with patients after stroke who suffer from hemiparesis, dysphagia, and/or aphasia. In addition to medication management, therapy is used to treat and assist patients in adapting to their new physiologic changes.
Artificial intelligence is used to collect and interpret data, then subsequently deliver a unique and tailored response through a robot. An example of this is Google’s Street View, which took billions of pictures of cars, and based on the types of cars within communities, were able to determine the community’s income, race, education, and political affiliations.
In rehabilitation, artificial intelligence and robotics are used in the form of exoskeletons, prosthetics, social robotics, and smart environments. An applicable example of artificial intelligence and robotics with use of a brain computer interface is the National Aeronautics and Space Administration’s X1 powered lower limb exoskeleton. The X1 was introduced as a potential therapeutic tool for the leading cause of neurologic disability, stroke. The X1 uses electroencephalogram (EEG), electromyogram, and goniometers to establish a brain computer interface. Decoding of the goniometers, electromyograms, and EEGs allowed the exoskeleton to reconstruct gait kinematics of the hip and knee joints in the hemiparetic patient.
Brain-computer interfaces have also been used to control robotic and prosthetic arms. The brain-computer interface records neural activity through an EEG or electrocorticography, decodes the neural activity through a computer, and finally sends these decoded commands to a robot to perform an action. Of note, EEGs are placed on the external surface of the cranium leading to limited topographical resolution and frequency range, whereas electrocorticographs are placed on the cortical surfaces of the brain allowing for better topographical resolution and improved accuracy.
The Economics of the Robotic Industry
The robotic industry continues to grow and integrate within our health-care system. Robotics can assist with diagnosis, prognosis, and treatment in rehabilitation by analyzing and interpreting live data to assist with rehabilitative therapy. In rehabilitation, we see the use of robotics to address therapy for postneurologic deficits such as hemiparesis, amputees with prostheses, and gait dysfunction. These advancements come at a premium cost. Examples of rehabilitation robots are therapeutic robots, assistive robots, exoskeleton robots, and prosthetic robots. A study performed by McCabe et al., which focused on comparing robotics and functional electrical stimulation and motor learning in patients with upper extremity dysfunction poststroke, noted that the cost of using robotics with motor learning was significantly more expensive when compared with functional electrical stimulation plus motor learning or motor learning alone. There was also no difference in treatment response across the different interventions.
In contrast, the US Department of Veterans Affairs’ Robotics Study analyzed the economics of robot-assisted therapy for long-term upper limb impairment after stroke and found at 36 weeks there was no significant differences between the intensive therapy group, robot-assisted therapy group, and usual care group. Thirty-six-week averages were $12,364 for the intensive therapy group, which included 50 individuals; $12,679 for the robot-assisted group, which included 49 individuals; and $19,098 for the usual care group, which included 19 individuals. Due to the number of individuals in the usual care group, the 36-week average was skewed. The study ultimately concluded that the robot-assisted therapy group used less Veterans Affairs care and had a lower average cost when compared with the intensive therapy group.
The global medical robots market is projected to reach $25.4 billion by 2028 from $ 9.2 billion in 2022. This market includes therapeutic, surgical, rehabilitation, and hospital automated robots. It is expected to grow at a compound annual growth rate of 18.5% from 2022 to 2028.
Robots of the Future
Many ideas regarding the future of robotics are currently in development. One idea theorizes that surgically placed electrodes within the brain could allow for neural spikes to be interpreted almost immediately. This would allow for accurate recording of neural activity, which could result in improved decoding and robotic output. There is also plenty of room for growth within the realm of brain-computer interfaces. The future of brain-computer interfaces will focus on improving function, reliability, safety, and convenience. Those who have lost the ability to control their neuromusculoskeletal system, such as patients with spinal cord injuries, amyotrophic lateral sclerosis, or locked-in syndrome, will be able to control a neuro-prosthesis with their brain waves. Instead of only decoding brain signals, future brain-machine interfaces will also be able to induce a signal back to the brain, allowing for CNS neuroplasticity to restore motor function.
References
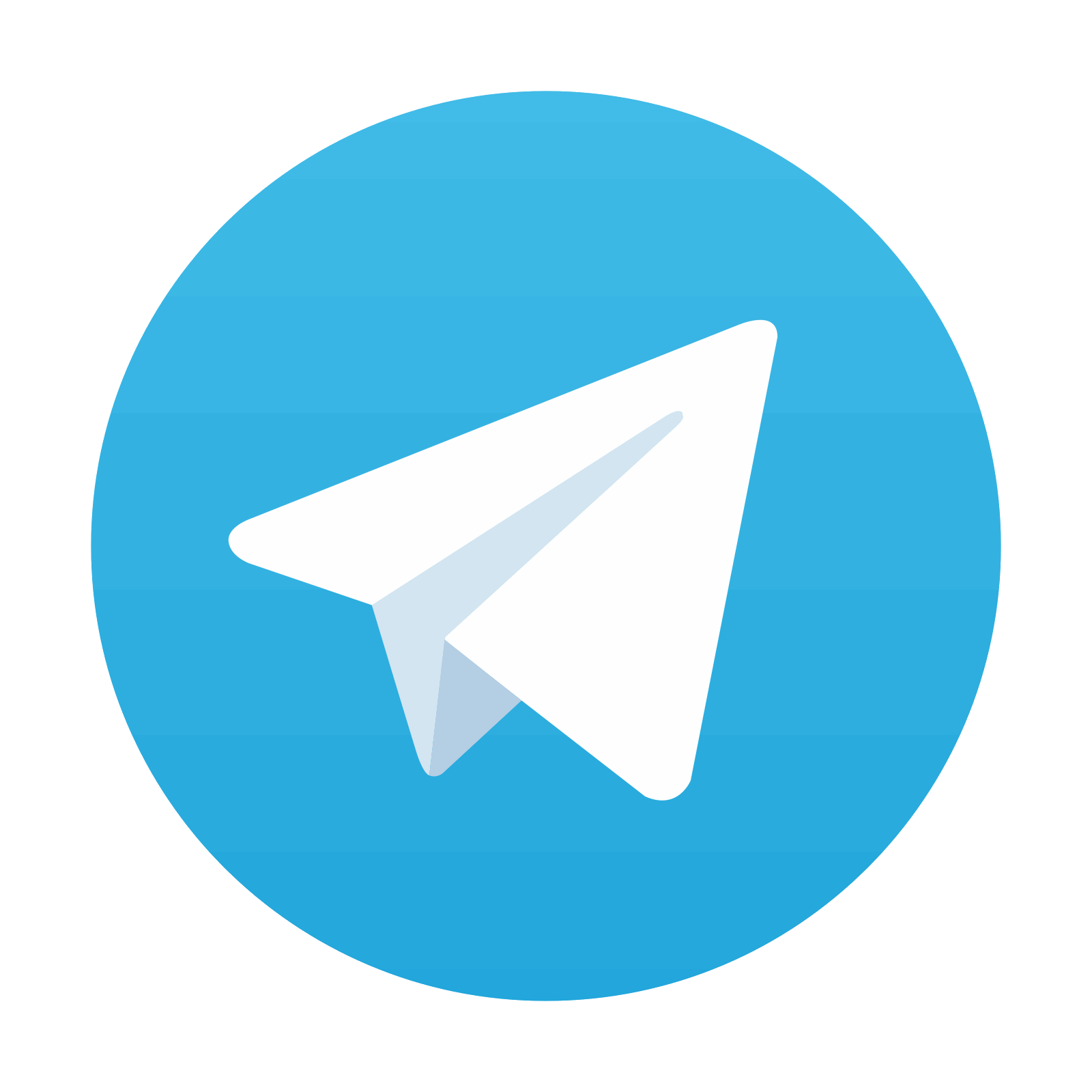
Stay updated, free articles. Join our Telegram channel

Full access? Get Clinical Tree
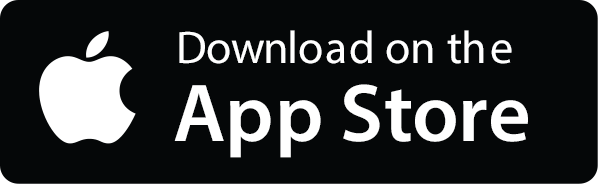
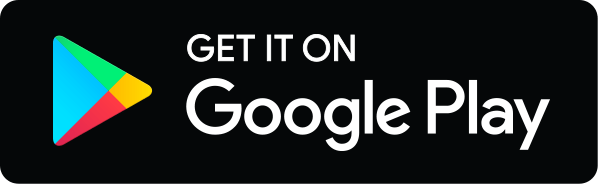
