Innate lymphoid cells (ILCs) and natural killer (NK) cells are components of the innate immune system that protect against invading pathogens.
ILCs and NK cells contribute to immune responses by amplifying inflammation or facilitating wound healing via the production of cytokines.
By functioning in an antigen-independent manner, these cells augment the early immune response, limit pathogen replication, and “buy time” for the adaptive immune response of B and T cells to develop and control offending agents.
Emerging data implicate ILCs and NK cells in rheumatologic conditions.
Introduction
As constituents of innate immunity, innate lymphoid cells (ILCs) and natural killer (NK) cells can provide early host responses against a variety of pathogens, including viruses, bacteria, fungi, and parasites. , The diversity of ILC and NK cell responses parallels the biology of T cells ( Fig. 16.1 ). , Therefore, much of the current understanding of ILC biology is built upon the scaffold of T cell differentiation.

ILCs are divided into three types based on development and function, such as cytokine production. Type 1 ILCs (ILC1s) produce interferon-γ (IFN-γ) and require the T-box transcription factor T-bet for their development, paralleling Th1 cells. ILC2s produce the type 2 cytokines IL-5 and IL-13, and are programmed by the transcription factors GATA3 and RORα, resembling Th2 cells. ILC3s includes two members, distinguished by expression of a natural cytotoxicity receptor (NCR) (i.e., NCR + ILC3s and NCR − ILC3s), require the transcription factor RORγt, and produce IL-17 and/or IL-22, akin to Th17 cells.
Two additional subsets, NK cells, studied for more than 40 years, and lymphoid tissue-inducer (LTi) cells are also considered innate lymphocytes and have been previously classified as belonging to ILC1s and ILC3s, respectively, but are now recognized as distinct cell lineages. While NK cells share a primary feature of ILC1s, such as the production of IFN-γ, several properties of NK cells clearly distinguish them from other ILCs. For example, under homeostatic conditions, NK cells actively circulate between the blood and multiple organs, while ILCs predominantly remain in extra-lymphoid tissues and rarely circulate. In addition, NK cells are primed to rapidly kill virally infected or transformed malignant cells, whereas ILC1s have reduced cytotoxic capacity. Furthermore, ILC1s and NK cells arise from distinct developmental pathways (discussed in the next section). As a result, NK cells likely play a distinct role in human pathophysiology compared with ILC populations.
LTi cells share many features with ILC3s, such as the transcription factors RORγt, Tox, AHR, TCF1, and Notch as well as the production of cytokines IL-17, IL-22, and lymphotoxin. , However, unlike ILC3s, LTi cells are essential during embryonic development to form secondary lymphoid tissue, such as lymph nodes and Peyer’s patches. This is a distinctive function of LTi cells. Similarly, LTi cells can also contribute to the development of tertiary lymphoid structures within organs subjected to chronic inflammation. In contrast to secondary lymphoid tissue, however, this is not a unique capacity of LTi cells as other cell types, such as T or NK cells, are able to initiate tertiary lymphoid tissue development. LTi cells can also be distinguished from ILC3s by the chemokine receptor CCR6 and a distinct developmental pathway (discussed in the next section). As a result, LTi cells are now considered a subset on their own.
Distinguishing Human ILCs and NK Cells
ILCs and NK cells are defined as lymphoid cells that do not require antigen receptor rearrangement for their development, unlike T and B cells, and can now be clearly distinguished from other leukocytes. For example, ILCs and NK cells lack proteins expressed on specific cell types, such as CD3 and CD5 (T cells), CD19 and CD20 (B cells), and CD13 and CD14 (myelomonocytes).
Discriminating between ILCs and NK cells has become important as cells formerly thought to be immature NK cells were subsequently identified as ILCs. For example, most ILCs are dependent on the cytokine IL-7 and therefore can be identified by the expression of the IL-7 receptor (CD127), whereas NK cells utilize IL-15 for survival and have high expression of the IL-2/IL-15 receptor β chain (CD122). In addition, NK cells can be identified based on the expression of the transcription factor Eomesodermin (EOMES), the cytolytic molecules granzyme and perforin, their MHC-binding receptors, and CD16, an Fc receptor responsible for antibody-dependent cellular cytotoxicity (ADCC). Despite these acknowledged differences, there is significant overlap between ILCs and NK cells, which still contributes to challenges in cell identification.
Target Recognition by NK Cells and Killer Immunoglobulin-like Receptors (KIRs)
One of the main functions of NK cells is direct killing of infected or transformed target cells with the help of germline-encoded NK cell receptors. The major family of these receptors is the killer immunoglobulin-like receptors (KIRs), consisting of both activation and inhibitory receptors. There are several KIR isoforms with two or three Ig-like domains (2D or 3D). Inhibitory receptors are designated as having long cytoplasmic tails, such as KIR2DL1, with intra-cellular immunoreceptor tyrosine-based inhibitory motifs (ITIMs). Those with short cytoplasmic domains, such as KIR2DS1, are activation receptors because they couple to signaling chains with immunoreceptor tyrosine-based activation motifs (ITAMs), similar to those in the T or B cell antigen receptor complexes. NK cells integrate the positive and negative signals from these respective receptors to determine whether to kill a target cell and release pro-inflammatory cytokines or to remain quiescent ( Fig. 16.2 ).

In addition to providing the first line of defense against virally infected or transformed cells, NK cells can cooperate with adaptive immunity to accelerate pathogen elimination. For example, NK cells are central mediators of ADCC, where (1) antibodies bind antigens on the surface of an infected or malignant cell, (2) NK cells use their low affinity Fc receptor for IgG (CD16) to recognize the cell-bound antibodies, and (3) subsequent CD16 crosslinking results in degranulation and lysis of the target cell. This antibody-mediated destruction of target cells by NK cells appears to be operative in a variety of clinical settings, including B cell depletion with the monoclonal anti-CD20 antibody rituximab for rheumatoid arthritis and vasculitis.
Due to this ability to elicit effector functions, CD16 is considered among the activation receptors on NK cells, which also include the natural cytotoxicity receptors (NKp46, NKp44, NKp30), NKG2C, NKG2D, and several KIRs (KIR2DS1, KIR2DS2, KIR2DS4). The ligands for these activation receptors have not all been identified, but they include alleles of the human leukocyte antigen (HLA) locus, including HLA-C (for KIR2DS1), HLA-E (NKG2C), and HLA-G (KIR2DL4). Ligands for NKG2D include MICA, MICB, and ULBP1-4, which are generally not expressed well under normal conditions but can be upregulated during periods of cell stress, such as during infection or malignancy. The corresponding activation receptor (NKG2D) can then trigger NK cell killing, thereby limiting the spread of infection or cancer.
Activation receptors trigger effector functions through their association with type I transmembrane-anchored proteins that contain a cytoplasmic ITAM: FcR γ, CD3 ζ, and DAP12. , Upon ligation of the activating receptor, tyrosine residues in the ITAMs are phosphorylated through Src family kinases (e.g., Lck, Lyn, Fyn, Src, Yes, and Fgr). These phosphorylation events lead to activation of the Syk family tyrosine kinases that trigger additional downstream signaling events involving phospholipase C-γ (PLC-γ) and Vav, which subsequently lead to actin reorganization, degranulation, and activation of the transcription factors NFAT, NF-κB, ERK, and AKT with associated transcriptional changes. While some activation receptors are able to elicit effector functions in isolation, other receptors only mediate activity in combination with other co-receptors.
In contrast to activation receptors, NK cell inhibitory receptors contain a cytoplasmic ITIM. Members of the inhibitory receptor group include 2B4, KLRG1, NKG2A, KLRB1, LAIR1, LILRB1, and several KIRs (KIR2DL1, KIR2DL2, KIR2DL3, KIR3DL1, KIR3DL2). When the inhibitory receptor is crosslinked, the ITIM is tyrosine-phosphorylated, which can recruit the tyrosine phosphatases SHP-1 or SHP-2, or the SH2 domain-containing inositol 5′phosphatase-1 (SHIP-1). Positioning SHP-1 and SHP-2 at the immunologic synapse by ITIMs leads to dephosphorylation of adapter signaling molecules, such as VAV, which abrogates ITAM-mediated signaling. Similarly, recruitment of SHIP leads to degradation of the signaling molecules PI(3,4,5)P 3 and IP 3 , which inhibits parallel signaling pathways downstream of ITAM signaling. Inhibitory receptor signaling is able to counteract activation receptors, protecting potential target cells from NK cell killing.
The ligands for the inhibitory receptors are frequently class I MHC alleles, such as HLA-A3/A11 (for KIR3DL2), HLA-C (KIR2DL1, KIR2DL2, and KIR2DL3), HLA-E (NKG2A), or any HLA class I (LILRB1). Notably, HLA-C alleles can be grouped into two broad categories based on amino acid residue 80, which dictates recognition by certain KIR isotypes (i.e., KIR2DL1 is specific for HLA-C [Lys80] while KIR2DL2 and KIR2DL3 are specific for HLA-C [Asn80]), indicating stringent specificity of KIRs for their HLA ligands, similar to the exquisite specificity of TCRs.
Biology of ILCs and NK Cells
ILCs and NK cells both originate from hematopoietic stem cells (HSCs) in the bone marrow. HSCs give rise to the common lymphoid precursor (CLP), which is the progenitor for B cells, T cells, NK cells, and ILCs. CLPs subsequently differentiate into the common innate lymphoid progenitor (CILP), the branch-point for ILC and NK cell commitment ( Fig. 16.3 ).

ILC Development
CILPs generate common helper innate lymphoid progenitors (CHILPs) under the influence of the transcription factors GATA3, TCF-1, and ID2. , In addition to the ILC1, ILC2, and ILC3 lineages, CHILPs are also precursors to LTi cells. The transcription factor PLZF, however, directs CHILPs to differentiate into the innate lymphoid cell precursor (ILCP), preventing formation of LTi cells. ILCPs are the direct precursor to ILC1s, ILC2s, and ILC3s.
The differentiation of ILCPs into ILC1s, ILC2s, and ILC3s is heavily influenced by environmental cytokines. For example, the cytokines IL-12, IL-15, and IL-18 lead ILCPs to differentiate into ILC1s and produce IFN-γ. , In contrast, IL-7, IL-25, and IL-33 promote the formation of ILC2s, while IL-7, IL-23, and retinoic acid drive ILC3 differentiation. Once formed, however, these ILC lineages are malleable and demonstrate plasticity. For example, ILC1s can convert to ILC2s, while ILC2s can be induced to produce the ILC1-defining cytokine IFN-γ. Similarly, there may also be conversion between ILC1s and ILC3s. , , Thus, ILCs can facilitate multiple pro-inflammatory processes, a feature currently under intense investigation.
NK Cell Development
Under the direction of several transcription factors (TOX, NFIL3, ID2, PU.1, Runx3, and ETS1), CILPs develop into NK cell precursors (NKPs), which are characterized by expression of CD122 without NK cell lineage markers. The expression of CD122 and other components of the IL15R are critical for NK cell development, as NKPs and their mature progeny are dependent on IL-15 for survival. NKPs further develop into immature then finally mature NK cells under the coordination of Gata-3, IRF-2, CEBP-γ, MEF, MITF, and two T-box transcription factors (T-BET and EOMES). , , Unlike mice where NK development largely takes place in the bone marrow, human NK cell development may also occur in secondary lymphoid tissues, such as the lymph node or spleen. This process of maturation is characterized by the acquisition of several surface receptors (CD16, KIRs, NKp30, NKp46, 2B4, NKG2A, NKG2C, NKG2D), effector molecules (perforin), and glycoproteins (CD56, CD57).
The glycoprotein CD56 distinguishes two populations of mature human NK cells (CD56 bright and CD56 dim ). , These two populations differ in terms of phenotype and functional capacity, with CD56 bright NK cells having robust proliferation and production of IFN-γ in response to IL-2, IL-12, IL-15, and IL-18, whereas CD56 dim NK cells express CD16, are highly cytotoxic, and produce IFN-γ in the context of target cell recognition. These two subsets of NK cells also differ in terms of anatomic prevalence; while CD56 dim NK cells comprise 90% of circulating NK found in the blood, the majority of NK cells found in secondary lymphoid organs are CD56 bright .
CD56 bright and CD56 dim NK cells have been hypothesized to be developmentally related, with the CD56 bright NK cells giving rise to CD56 dim NK cells. This is supported by observations that CD56 bright NK cells contain longer telomeres, have more robust proliferation after stimulation, and can generate CD56 dim NK cells after an in vitro culture. This model of linear differentiation has been suggested to occur during development and homeostasis. Data from nonhuman primates suggest, however, that these two populations undergo little transition during homeostasis. Instead, CD56 bright NK cells may only give rise to CD56 dim NK cells in substantial numbers during generation or replenishment of the NK cell pool, such as during development, after bone marrow transplantation, or after targeted NK cell ablation via IL-15 deprivation.
NK Cell Function
The two well-defined features of NK cells are their ability to lyse target cells and produce inflammatory cytokines. In contrast with T and B cells from the adaptive immune response, NK cell function does not require prior exposure to the offending tumor or viral pathogen, but instead is present on the initial encounter. NK cell activity is, however, finely tuned based on the process of licensing and the state of NK cell activation (discussed in the next section).
The importance of NK cells in combating viral infection has been established from humans with NK cell deficiencies. A patient with complete and relatively selective NK cell deficiency suffered two life-threatening herpesvirus infections: disseminated varicella zoster virus and cytomegalovirus pneumonitis at ages 13 and 17, respectively. In addition to NK cell deficiency, impaired NK cell function also leads to increased vulnerability to herpesviruses. Small case series of siblings have demonstrated that NK functional defects may lead to severe infections from EBV and HSV. Thus, NK cells play a critical role in human immunity to viral pathogens.
NK cells have other functions in addition to elimination of target cells. For example, NK cells accumulate at the maternal-fetal interface in the placenta and have been suggested to play a supportive role in pregnancy by preventing trophoblast invasion and the development of pre-eclampsia. , These uterine NK cells have a distinct transcriptional program compared to traditional NK cells, possibly due to the non-inflammatory requirements of fetal-placental development. As a result, NK cell function can be heavily influenced by its surrounding environment.
Regulation of NK Cell Activation, Licensing, and Missing Self
While mature NK cells are functional at a basal resting state, activation of NK cells through inflammatory cytokines and cell-cell contact greatly enhances NK cell activity. Several cytokines activate NK cells, such as IFN-α, IFN-β, IL-12, IL-15, and IL-18. Upon activation, NK cells produce pro-inflammatory cytokines such as IFN-γ, TNF, and granulocyte-macrophage colony-stimulating factor (GM-CSF). These inflammatory cytokines are important to augment anti-viral and anti-tumor responses. In addition, NK cells can release chemokines to recruit additional immune cells to initial sites of inflammation.
Most ligands for the KIRs on NK cells belong to the HLA region, a locus with high genomic variability. Moreover, the KIRs themselves are highly polymorphic. As a result, inherited KIR ligands and KIRs show profound variability from one individual to the next, risking the possibility of autoreactivity in individuals who do not inherit the right combination of cognate ligands for their inhibitory receptors. Consequently, the NK cell population must finely tailor its potential for activation, a process termed licensing .
Licensing is an education process for NK cells that depends on exposure to host class I MHC. Experiments in mice have demonstrated that genetic lesions affecting normal expression of all class I MHC proteins do not affect NK cell number but lead to severe NK cell defects, such as dysfunctional NK killing and cytokine production, contrary to what would be otherwise expected by the missing-self hypothesis, as discussed in the next section. Reintroduction of a selected class I MHC gene allows NK cells with receptors that specifically recognize that class I MHC protein to become fully functional. This process has also been observed in humans for specific pairings of inhibitory receptors and their corresponding HLA allele. , As a result, NK cells are only “licensed” (or educated) when they recognize self-class I MHC proteins.
As mentioned previously, several ligands for NK inhibitory receptors are class I MHC proteins, HLA-A, -B, and -C. The function of these class I MHC proteins is to present intra-cellular antigens to cytotoxic CD8 T cells. This allows CD8 T cells to identify virally infected cells due to the presentation of viral antigens. Consequently, to evade CD8 T cell responses, multiple viruses decrease or remove class I MHC genes from the surface of the infected cell. Class I MHC downregulation, however, enables NK cell killing, providing a critical role for NK cells in eliminating viruses that utilize this immune-evasion strategy.
This function of NK cells is described by the “missing self” hypothesis since class I MHC is nearly ubiquitously expressed and normally prevents NK cells from killing target cells due to inhibitory receptors that bind class I MHC. When the target cell reduces the expression of class I MHC (such as during viral infection) (i.e., missing self), the NK cell receives less inhibitory signals, tipping the balance towards NK cell activation and target cell killing. Thus, the “missing self” hypothesis is a major guiding principle of NK cell function, but current hypotheses suggest that missing self reactivity requires licensed NK cells that were educated by self-class I MHC molecules.
The Evolution of Missing Self
“Missing self” appears to have developed in parallel in separate mammalian species, a prime example of convergent evolution. For example, while human NK cells utilize an assortment of KIRs, mice do not have KIRs and instead independently evolved a collection of structurally distinct receptors, termed the Ly49 family, that are not present in humans but serve the same purpose. While the mammalian strategy to detect “missing self” is young in evolutionary terms, cytotoxic cells themselves are phylogenetically old. Indeed, cell-mediated cytotoxicity may have even evolved prior to the evolution of adaptive immunity and the development of recombination-activating gene (RAG) in jawed fish. In support of this, cell-mediated cytotoxicity occurs not only in multiple non-mammalian vertebrates, but also in invertebrates, protecting the host from invasive cells from another individual of the same species, presumably by discriminating “self” from “non-self.” Thus, in contrast to the self- versus nonself-discrimination present in both vertebrate and invertebrate species, class I MHC-dependent “missing self” likely evolved more recently under species-specific, selective pressures.
ILC Functions
As mentioned previously, ILCs broadly function in parallel to their CD4 Th cell counterparts. However, there are several notable differences between ILCs and Th cells. In contrast to T cells, ILCs do not express unique rearranged antigen receptors (i.e., the T cell receptor). Instead, similar to NK cells, ILCs can respond quickly to inflammatory cytokines to potentiate an immune response. While T cells and B cells must undergo several days of proliferation and differentiation to acquire effector functions, due to clonal selection, cytokine-induced activation allows ILCs (and NK cells) to respond rapidly in the early phases of an immune response. An additional feature that accelerates the ILC response over T cells is that ILCs are resident in the tissues, already present at the site of initial inflammation, while T cells generally circulate and must home to inflammatory foci.
Role of NK Cells in Health and Disease
NK Cell Correlates With Rheumatologic Diseases
NK cells have been examined in multiple rheumatologic conditions. Multiple autoimmune diseases are associated with fewer circulating NK cells and/or reduced NK cell functionality. Whether this is an initial risk factor or secondary to the development of a systemic inflammatory disorder remains unclear. Moreover, reduced NK cell cytotoxicity appears to be a common feature of rheumatologic disorders, but this finding should be interpreted with caution. Disorders with excess production of NK cells, such as chronic NK cell lymphocytosis, are associated with increased production of immature NK cells that have not yet acquired full effector functions. , As a result, autoimmune diseases that accelerate NK cell development could be associated with reduced NK cell killing, regardless of whether diminished cytotoxicity was a factor in developing the rheumatic disorder. Nevertheless, these correlations raise the possibility that NK cells participate in rheumatologic diseases.
Rheumatoid Arthritis and NK Cells
Similar to other autoimmune conditions, features of NK cells are altered in the setting of rheumatoid arthritis (RA). For example, the frequency and cytotoxicity of circulating NK cells in the blood are reduced while the ability for NK cells to produce IFN is not impaired. In contrast, examination of the inflamed joints of RA patients has revealed an expansion of CD56 bright NK cells, a lineage with enhanced cytokine production but reduced cytotoxicity. , Thus, decreased circulating NK cells may be the result of trafficking to the inflamed tissue, but it is possible the diminished cytotoxicity may be a risk factor for the development of RA.
Systemic Lupus Erythematosus and NK Cells
Studies in systemic lupus erythematosus (SLE) have reported low numbers of circulating NK cells and reduced NK cell killing. While improvement in SLE disease activity led to normalization of NK cell numbers, the defect in NK cell killing remained. Thus, NK cell cytotoxicity appears permanently altered in the setting of SLE and may be a contributing risk factor for the development of disease, though considerations mentioned previously for RA may apply.
Juvenile Idiopathic Arthritis and NK Cells
Patients with juvenile idiopathic arthritis (JIA) have also been reported to have diminished levels of peripheral blood NK cells. This observation was most extreme in patients with the systemic form of JIA. NK cells in JIA patients were also less effective killers, expressing lower levels of the cytotoxic molecule perforin.
Macrophage Activation Syndrome/Hemophagocytic Lymphohistiocytosis and NK Cells
Macrophage activation syndrome (MAS) is a rare robust inflammatory disorder associated with rheumatologic diseases. MAS shares a strong clinical overlap with hemophagocytic lymphohistiocytosis (HLH). Unlike some rheumatologic conditions, MAS/HLH has a robust mouse model. NK cells and cytotoxic CD8 T cells from mice deficient in the cytotoxic molecule perforin are unable to kill virally infected cells. Infection of these mice with specific viruses leads to a robust inflammatory response that resembles HLH and is eventually fatal. , This is thought to be due to a persistent viral stimulus of the immune system because the cytotoxic cells are unable to clear the virus. In support of this, persistent stimulation of innate immunity through Toll-like receptor 9 (TLR9) leads to macrophage activation syndrome-like disease in mice.
Consistent with the mouse models, NK cell defects likely underlie a large subset of human cases of MAS and HLH. For example, mutations in the cytotoxic molecule perforin that lead to loss of expression and absent NK cell killing have been identified in inherited forms of HLH. , Furthermore, NK cell defects have been found in sufficient frequency in these diseases that they were included as part of a proposed diagnostic criteria for HLH and MAS. ,
KIR/HLA and Their Association With Autoimmune Disease
Through genetic studies, several rheumatologic conditions have been associated with specific HLA and KIR alleles. For example, a meta-analysis has suggested KIR2DL3, KIR2DL5, KIR3DL3, and KIR2DS5 decrease the risk of developing RA. In contrast, a genome-wide association study associated the LILRB3 locus, which encodes an NK inhibitory receptor, as a risk allele for developing Takayasu’s arteritis. By comparison, inheritance of the activating receptor KIR2DS2 in the absence of the inhibitory KIR2DL2 is associated with scleroderma. Thus, the relative risk or benefit of specific KIR alleles varies by rheumatologic condition.
While genetic HLA associations with disease risk are generally thought to be due to the role of HLA in antigen presentation to T cells, it remains possible that the risk association may also be related to the binding of HLA alleles to NK cell activation or inhibitory receptors. For instance, a specific HLA-C allele (HLA-C∗06:02) is associated with the development of psoriasis and psoriatic arthritis. One notable feature of HLA-C∗06:02 is that it does not bind the inhibitory KIR, KIR2DL1. As a result, NK cells may be predisposed towards activation without such inhibitory signaling. Consistent with this hypothesis, patients who inherit the activating receptors KIR2DS1 and KIR2DS2 with HLA-C∗06:02 homozygosity have increased risk of developing psoriatic arthritis.
The genetic association between KIR and autoimmune diseases implicates NK cells in playing a pathogenic role in these disorders. However, this interpretation warrants caution. While KIRs were first identified on NK cells, KIRs are now known to be expressed by other immune cells. For example, RA patients with vasculitis were more likely to have inherited the activating receptor KIR2DS2 compared with RA patients without vasculitis or healthy controls. However, in that study, CD4 T cells were demonstrated to have higher expression of KIR2DS2 in RA vasculitis cases compared with healthy controls, raising the possibility that the risk for RA vasculitis from KIR2DS2 may be mediated by CD4 T cells instead of, or in addition to, NK cells. Similarly, patients with HLA-B27-associated spondyloarthropathy have higher expression of the inhibitory receptor KIR3DL2 on NK cells and CD4 T cells, , and KIR3DL2-expressing CD4 T cells are enriched in the synovial fluid of patients with spondyloarthritis. In addition, the interaction between HLA-B27 and KIR3DL2 appears to increase the survival of this particular CD4 T cell population. Thus, some of the pathogenesis associated with HLA-KIR interactions may be mediated by non-NK cells, particularly T cells.
ILCs and Potential Contributions to Rheumatologic Disease
ILC populations have been reported to be altered in the setting of autoimmune disease. In psoriatic arthritis, synovial fluid contains elevated numbers of both ILC1s and NKp44 + ILC3s. The synovial ILC3s produce the inflammatory cytokine IL-17, a pathogenic cytokine in psoriatic arthritis that is currently targeted by biologic therapy. Similarly in ankylosing spondylitis, specific subpopulations of ILC3s are increased in the gut, blood, synovial fluid, and bone marrow. These ILC3s produce IL-17, which is also a target in ankylosing spondylitis. Thus, targeted therapy for different forms of spondyloarthritis may work through successfully mitigating ILC function.
It is difficult to discern the relative contribution of ILCs to the development of rheumatologic conditions after the disease has been established. However, certain single-nucleotide polymorphisms (SNPs) associated with inflammatory bowel disease or RA are located in regulatory regions of the genome called super-enhancers that are specifically used by ILCs compared with T cells. This work suggests that genomic variations that influence ILC behaviors may increase the risk of developing autoimmune disease.
In addition, recent work has highlighted ILC alterations in individuals at risk for rheumatologic disorders before the development of full-fledged disease. Specifically, a study examined lymph node biopsies from subjects with early RA, subjects with pre-clinical RA due to the presence of autoantibodies, and healthy controls. Compared to healthy controls, subjects with early RA or pre-clinical RA demonstrated increased ILC1s. These disturbances in ILC populations that occur during the earliest detectable phases of disease support the possibility that ILCs participate in the development of rheumatologic disorders.
Summary
ILCs and NK cells provide important innate immune functions in antipathogen and anti-tumor immunity, both before and in concert with the adaptive immune response. In addition, there is emerging evidence that ILCs and NK cells may participate in inappropriate autoimmune responses and contribute to rheumatologic disease. However, whether specifically detecting or manipulating ILCs and NK cells will have diagnostic or therapeutic benefit in patients with autoimmune disorders remains to be determined.
References
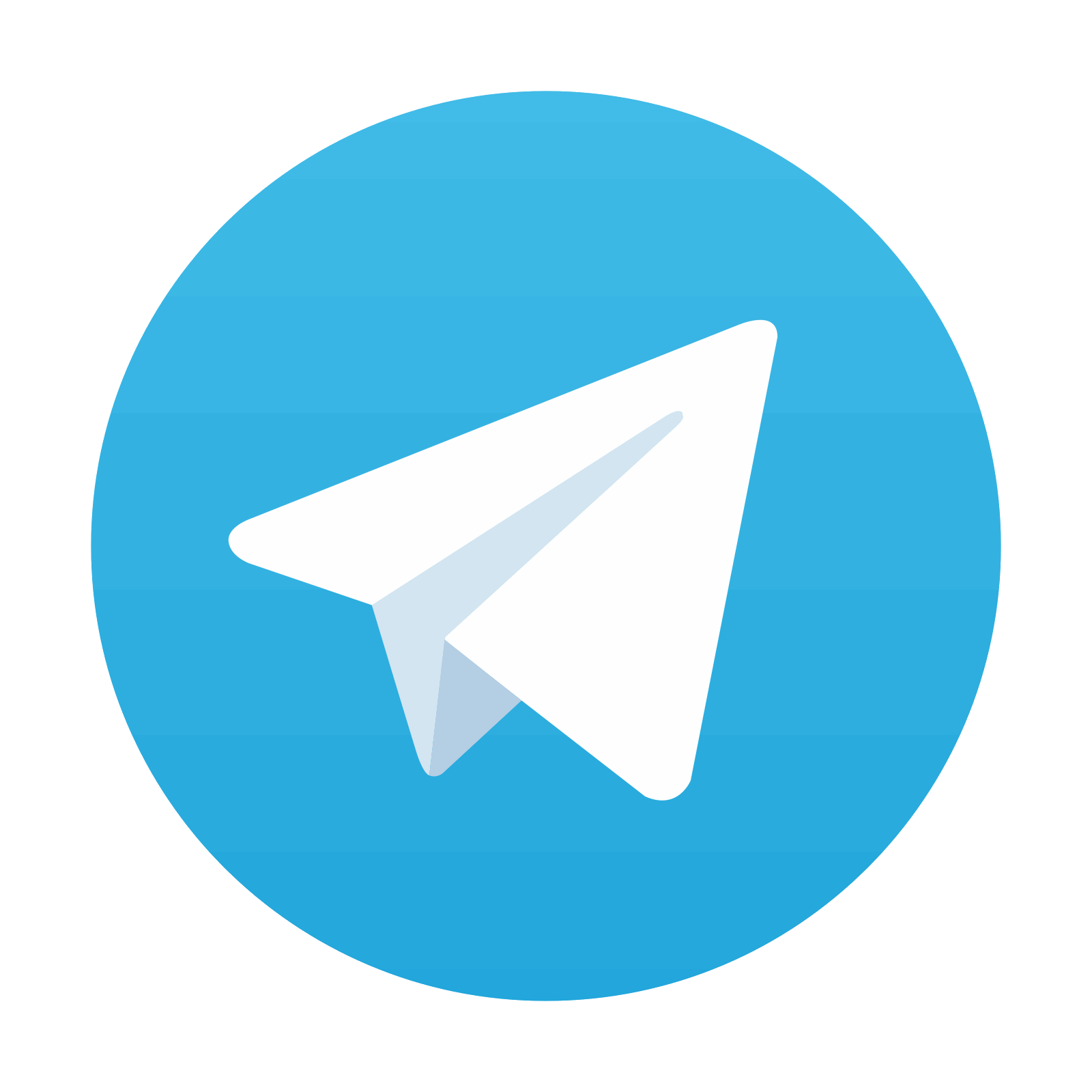
Stay updated, free articles. Join our Telegram channel

Full access? Get Clinical Tree
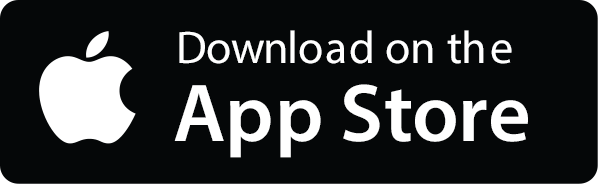
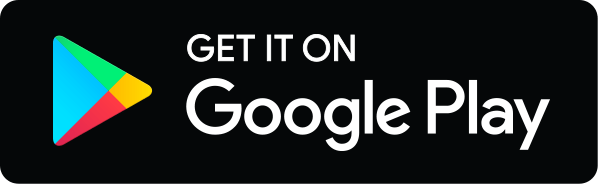
