Abstract
Objectives
To evaluate the effect of ankle-foot orthosis on lower limbs kinematic segmental covariation (KSC) among stroke patients.
Methods
Ten chronic hemiparetic spastic stroke patients presenting with a lack of ankle dorsiflexion were assessed with instrumented gait analysis under three conditions: wearing a shoe, with a prefabricated ankle-foot orthosis (AFO), and with a dynamic AFO. Kinematic parameters were recorded and computed KSC was calculated according to Borghese’s methodology.
Results
Contrary to the prefabricated AFO, the dynamic AFO improved KSC of the paretic side. We observed a high correlation between the external mechanical work and the affected side’s KSC. In the unaffected side, KSC was globally unchanged.
Conclusion
In stroke patients, wearing a dynamic AFO improves KSC of the paretic lower limb only.
Résumé
Objectif
Notre objectif était d’évaluer l’effet de l’appareillage de la cheville (traitement mécanique) sur la covariation cinématique segmentaire des membres inférieurs chez des patients hémiparétiques.
Méthode
Dix patients hémiparétiques spastiques présentant un déficit de flexion dorsale de la cheville ont été évalués par analyse quantifiée de la marche sous trois conditions : sans orthèse, avec orthèse suropédieuse préfabriquée et avec orthèse suropédieuse dynamique. À partir des données cinématiques enregistrées par analyse instrumentée, nous avons calculé la covariation cinématique segmentaire en suivant la méthode de Borghese.
Résultats
Contrairement à l’orthèse suropédieuse préfabriquée, l’orthèse suropédieuse dynamique améliore la covariation cinématique segmentaire du côté parétique. Il existe une haute corrélation entre le travail mécanique externe et la covariation cinématique segmentaire du côté parétique. Du côté sain, la covariation cinématique segmentaire n’est globalement pas modifiée par l’appareillage.
Conclusion
Chez les patients hémiparétiques, l’orthèse suropédieuse dynamique améliore la covariation cinématique segmentaire du côté parétique uniquement.
1
English version
1.1
Introduction
Human walking is a complex activity, using a repetitive sequence to move the body forward while simultaneously maintaining stance stability. Biomechanics involve the study of kinematics, kinetics and mechanical work . With those objective data, gait analysis allows a precise description of gait abnormalities and a better targeting and assessment of treatment strategies.
The coordination of a large number of muscles is necessary to enable forward progression of the body while maintaining balance and limiting energetic consumption. However, the degrees of freedom of muscle activity do not match those of the body’s center of mass, as the electromyographic activity patterns in the lower limbs are much more complex that a simple alternation between flexors and extensors. They contribute to generate the center of body mass motion, reduced to two degrees of freedom of linear motion (horizontal and vertical translation) . In order to match the muscle patterns to the pattern of motion of the center of body mass, the study of intersegmental lower limbs coordination was explored by different researchers. Some of them used angular velocity to define the temporal sequencing of joint movements during transitional phases of the gait cycle. Another approach, based on the orientation of lower limb segments, was proposed by Borghese et al. : the temporal waveform of the angles of lower limb segments (thigh, shank and foot) relative to the vertical define the elevation angles, which are known to be more stereotypical across trials and subjects than the corresponding waveforms of the joint angles . When plotted one versus the others in a 3D-position space graph, the elevation angles covary along an attractor plane. This approach is called kinematic segmental covariation (KSC) . In normal subjects, KSC follows a simple and consistent law under different walking modes: KSC of lower limb segments in backward locomotion is, for example, the mirror image of that of the forward locomotion, while mechanical characteristics and patterns of muscle activity differ drastically from one to another . It can also be applied to cats (quadruped walking), with a remarkable similarity to humans (biped locomotion), despite important differences in the postural attitude in these two species . This law leads to an original approach of human locomotion, supporting the hypothesis that the KSC law is controlled by spinal Central Pattern Generators (CPGs) and reflects lower limb coordination . The covariation law’s application has been studied in a few central nervous system diseases. Grasso et al. demonstrated an improvement in KSC among idiopathic Parkinson’s disease patients under continuous infusion of apomorphine or under electrostimulation of both internal globi pallidi. Dan et al. reported an improvement in KSC after an intrathecal baclofen bolus in a patient presenting with a hereditary spastic paraparesis. More recently, we showed an improvement in KSC of both lower limbs in spastic stroke patients, 2 months after botulinum toxin injections in the paretic limb .
The objective of the present study was to assess the impact of mechanical constraint (bracing) on intersegmental covariation in stroke patients. In another previous study, we indeed assessed the value of a dynamic ankle-foot orthosis (AFO) — the Chignon ® AFO, represented in Fig. 1 — and a prefabricated thermoplastic AFO (PAFO) in the gait of hemiplegic patients, in comparison with the absence of an orthosis . We observed a significant and similar reduction of the muscular mechanical work with both orthoses. The Chignon ® orthosis significantly improved spontaneous walking speed and the ankle’s segmental kinematics, in contrast to the PAFO.
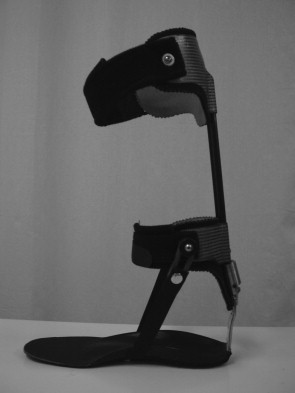
1.2
Methods
1.2.1
Patients, ankle-foot orthosis and instrumented gait analysis
We used kinematic data acquired in a previous study : in summary, ten chronic hemiparetic spastic stroke patients presenting with a lack of ankle dorsiflexion at toe-off were asked to perform an instrumented gait analysis under three conditions: wearing a shoe, with a PAFO, and with a Chignon ® AFO. There were nine males and one female. Their mean age was 49 ± 20 years (range: 20–80), and the mean time since stroke was 28 ± 18 months (range: 7–72). All the patients had received a study information sheet and signed an informed consent form prior to study initiation. The study protocol was approved by the local independent ethics committee.
To specifically analyze segmental kinematics, each subject was fitted with 19 reflective anatomical markers positioned according to the model of Davis et al. and was asked to walk on a treadmill at spontaneous speed. This speed was firstly evaluated following an overground 10 m speed test performed under the “shoe” condition. Each patient walked then on the treadmill at the same spontaneous speed under the three conditions. Six infrared cameras (ELITE system) placed around the treadmill filmed the coordinates of these reflective markers every 10 ms in 3D. Ten successive cycles were recorded for each patient in each condition. Riley et al. have shown that treadmill gait is qualitatively and quantitatively similar to overground gait. However, to our knowledge, no previous study assessed the impact of treadmill gait on elevation angles, and particularly in impaired subjects. Riley et al. indicated indeed in their study that “further studies will be required to determine the appropriateness of treadmill-based motion analysis for frail or impaired subjects”.
Internal mechanical work (W int ), external mechanical work (W ext ) and energetic cost (C) data were previously computed for each patient and taken back from our previous publication .
1.2.2
Statistical analysis
We studied the angles of lower limb segments kinematics (thigh, shank and foot) relative to the vertical, i.e., the elevation angles. Borghese et al.’s methodology was used to analyze the planar covariation of those elevation angles during the gait cycle. The elevation angles of thigh, shank and foot were plotted one versus the others in a 3D-position-space gait path, as shown in Fig. 2 for one normal subject and one patient wearing a shoe, PAFO and AFO. The plane orientation and the shape of the loop reflected the phase relationship between the different segments and therefore the timing of the intersegmental covariation. The path progressed over time in the counterclockwise direction, with heel contact and toe-off phases corresponding, respectively, to the top and bottom of the loop.
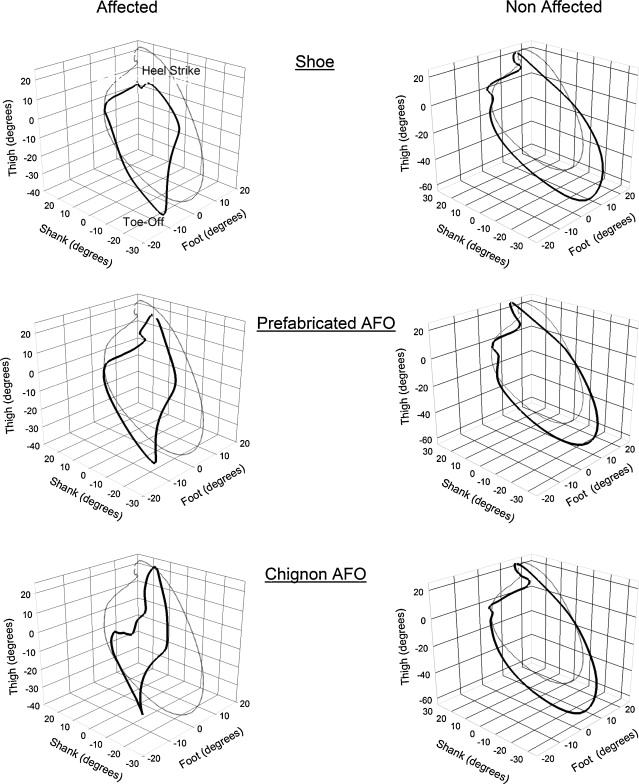
Statistical analysis of the angle covariation was based on principal component (PC) analysis. PCs were computed by pooling the sample of time-varying angles after subtracting the mean. A 3 × 3 covariance matrix was computed over all trials performed by a given subject in all conditions. The changes of the elevation angles of the thigh, shank, and foot covaried linearly throughout the gait cycle in normal gait. The thigh-shank-foot 3D loops described a path that could be fitted by a plane computed by means of orthogonal regression . The percentage of variance of the first two components (PV 1 , PV 2 ) identified the best-fitting plane of angular covariation. A greater PV 1 value induced a more eccentric loop (closer to a line segment). The third component defined the plane orientation (0 = perfect plane).
Reference values for normal subjects were previously computed .
In order to compare the effect of bracing (factor), we tested each variable (PV) using a one-way repeated measure ANOVA for each lower limb. If P value was significant (< 0.05), we used a post hoc analysis (Holm-Sidak method); these data are presented as means plus or minus standard deviations. Not normally distributed data underwent nonparametric statistical analysis (Friedmann’s repeated measure ANOVA) combined with a post hoc analysis (Tukey test) and are presented as the median [interquartile range].
Finally, a Pearson’s correlation was used between the PV values and the mechanical variables and the energetic cost. According to Shrout and Fleiss , a correlation coefficient ( r ) > 0.75 indicated excellent reliability, r of 0.40 to 0.75 indicated fair to good reliability, and r < 0.40 indicated poor reliability.
All statistical analyses were performed using SigmaStat software, version 3.1.
1.3
Results
The results are shown in Table 1 .
Affected side : results of one-way ANOVA on the rank | |||||
---|---|---|---|---|---|
Shoe [median and interquartile range] | Prefabricated ankle-foot orthosis [median and interquartile range] | Chignon ® orthosis [median and interquartile range] | P | Power | |
PV 1 (%) | 67.4 [64.3–78.1] | 73.1 [70.7–80.2] | 79.6 [73.6–82.9] a | 0.003 | 0.83 |
PV 2 (%) | 31.9 [27.4–33.9] | 25.8 [18.9–28.6] | 19.5 [15.1–25.7] a | 0.007 | 0.95 |
PV 3 (%) | 0.8 [0.4–1.4] | 0.7 [0.4–1.1] | 0.8 [0.4–1.6] | 0.39 | 0.65 |
Unaffected side: results of one-way ANOVA | |||||
---|---|---|---|---|---|
Shoe (mean ± SD) | Prefabricated ankle-foot orthosis (mean ± SD) | Chignon ® orthosis (mean ± SD) | P | Power | |
PV 1 (%) | 70.4 ± 4.9 | 69.8 ± 5.8 | 70.1 ± 5.2 | 0.83 | NA |
PV 2 (%) | 28.7 ± 5.0 | 29.4 ± 5.7 | 29.1 ± 5.1 | 0.78 | NA |
PV 3 (%) | 0.9 ± 0.4 | 0.8 ± 0.4 | 0.7 ± 0.2 b | 0.04 | 0.47 |
a Significant difference in the Tukey test, relative to the « shoe only » value.
b Significant difference in the Holm-Sidak method relative to the « shoe only » value.
In the affected side, the percentage of variance of the first two factors is improved by wearing an AFO. PV 1 increases from 67.4% [64.3–78.1] under the “shoe only” condition to 73.1% [70.7–80.2] with the PAFO and to 79.6% [73.6–82.9] with the Chignon ® AFO. Only the difference between the shoe condition and the Chignon ® AFO condition is statistically significant ( P = 0.003). PV 2 follows the same pattern, decreasing from 31.9% [27.4–33.9] under the “shoe only” condition to 25.8% [18.9–28.6] with the PAFO and to 19.5% [15.1–25.7] with the Chignon ® AFO. As for PV 1 , only the difference between the shoe condition and the Chignon ® AFO condition is statistically significant ( P = 0.007). PV 3 value (index of the planarity of the loop) is not modified by bracing; its value ranges from 0.8% [0.4–1.4] under the “shoe only” condition to 0.7% [0.4–1.1] with the PAFO and to 0.8% [0.4–1.6] with the Chignon ® AFO ( P = 0.39).
On the unaffected side, the percentage of variance of the first two factors is not modified by the affected side’s bracing. PV 1 remains stable at 70.4 ± 4.9% with the shoe, 69.8 ± 5.8% with the PAFO and 70.1 ± 5.2% with the Chignon ® AFO ( P = 0.83). PV 2 does not change either, with values at 28.7 ± 5.0% with the shoe, 29.4 ± 5.7% with the PAFO and 29.1 ± 5.1% with the Chignon ® AFO ( P = 0.78). PV 3 , is slightly improved by bracing; its value passes from 0.9 ± 0.4% under the shoe condition to 0.8 ± 0.4% with the PAFO and to 0.7 ± 0.2% with the Chignon ® AFO. Only the difference between the shoe condition and the Chignon ® AFO condition is statistically significant ( P = 0.04).
Those results are illustrated by a typical trace presented in Fig. 2 .
Fig. 3 shows the correlation between PV values of the affected side and external mechanical work (W ext –left column), internal mechanical work (W int –middle column) and energetic cost (Cost–right column). We observe a high correlation between W ext and PV 1 ( r 2 = −0.61) and PV 2 ( r 2 = 0.57). The correlation between the PV 1 and PV 2 values and W int ( r 2 = −0.39; 0.37), and between the PV 1 and PV 2 values and C ( r 2 = −0.41; 0.39) is good. The correlation between PV 3 and mechanical work, and between PV 3 and cost, is poor.
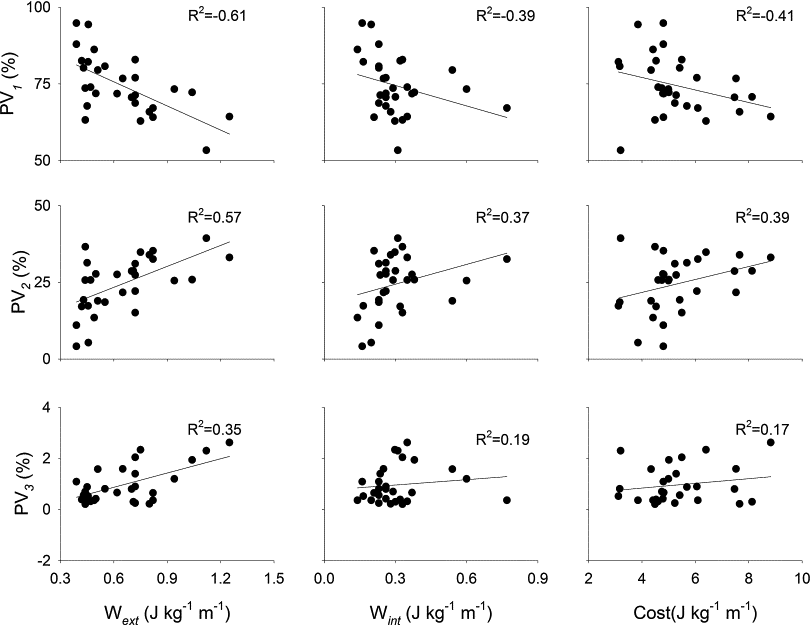
1.4
Discussion
Our results showed a significant improvement in elevation angles in the affected lower limb in hemiparetic spastic stroke patients presenting with a lack of ankle dorsiflexion at toe-off braced with a dynamic AFO; we indeed observed an increase in PV 1 value and a decrease in PV 2 value, while PV 3 (index of the planarity of the loop) remained stable.
Many studies have shown that the elevation angles, in contrast with real joint angle of the lower limbs, are stereotyped and describe a regular loop lying on a plane in normal gait . For example, during backward walking, the elevation angles are nearly identical to that of forward locomotion despite the different electromyographic patterns. This suggests that the planar covariation reflects a form of neural control rather than biomechanical constraints . The origins of this planar covariation law are debatable; the existence of such a robust kinematic pattern suggests that this law may represent an independent control by the central system nervous of limb geometry . In this idea, a biomechanical constraint (bracing) would not affect the unaffected side’s elevation angles. In a previous study , we described an improvement in elevation angles of both lower limbs in spastic stroke patients, two months after botulinum toxin injections in the paretic limb. As we discussed in our previous work, such a result on the unaffected side’s elevation angles could be an argument in favor of a central impact of treatment via the spinal CPGs. A retrograde transport of botulinum toxin along motor fibers to the motoneurons has indeed been described in the literature . The present study’s results could be thus in favor of that hypothesis, but the question remains open.
We nevertheless observed in the present study a correlation between elevation angles and mechanical work, which tends to confirms the link between neurophysiology and mechanics of gait. Bianchi et al. showed that the covariation plane parameters were found to predict mechanical energy expenditure. These authors demonstrated the presence of a linear correlation between another variable, the thigh eigenvector of 3rd component of the PC (U3t), and the net external mechanical power (Pu); smaller values of U3t tended to be associated with smaller values of Pu, and vice versa. Our results tend to confirm Bianchi et al.’s , as we observed a relationship between the shape of the loop (PV 1 and PV 2 ) and the mechanical work and energy cost.
Only one previous study, conducted by Barela et al., assessed the effect of bracing on intralimb coordination in hemiplegic subjects . The unaffected side’s results are in contradiction to those of the present study. The authors compared thigh-shank relative phase angle (angular velocity) between hemiplegic and non-disabled adult subjects. The hemiplegic subjects were asked to walk braced with an AFO, at spontaneous speed. The non-disabled subjects were divided into three groups: the first group walked at spontaneous speed, the second one as slowly as the hemiplegic subjects, and the third group walked slowly and braced with a right AFO. The authors observed that intralimb coordination was remarkably similar in normal subjects when walking at preferred speed, slow speed or mechanically constrained by an AFO. In hemiplegic subjects, walking slowly with or without AFO accounted for 22% of the variance in intralimb coordination of the affected limb and 16% in the unaffected limb. In the present study, the unaffected side’s results differ from Barela et al.’s , who observed a modification of intralimb coordination of the unaffected side after the affected side’s bracing. This difference could be due to the type of AFO used in the study. Indeed, Barela et al. used a fixed AFO. In the present study, a quite flexible PAFO and a dynamic AFO were chosen, and our initial objective was to compare the positive impact of two different type of AFO on elevation angles. Furthermore, the analysis of intralimb coordination used in Barela et al.’s study was not based on the same principles of KSC used in the present work. A future research work could include the comparison of KSC in hemiplegic patients, braced with a fixed AFO, a PAFO and a dynamic AFO.
1.5
Conclusion
In stroke patients, wearing a dynamic AFO improves KSC of the paretic lower limb only. Like in our previous work , we found a difference between the PAFO and the dynamic AFO. The PAFO did not modify enough the PV values to reach the level of statistical significance. The advantages of the Chignon ® dynamic AFO were already discussed in our previous study .
Disclosure of interest
The authors declare that they have no conflicts of interest concerning this article.
2
Version française
2.1
Introduction
La marche est une activité complexe nécessitant une séquence répétitive pour propulser le corps en avant à chaque pas tout en maintenant une stabilité durant les phases d’appui. La biomécanique de la marche comprend l’étude de la cinématique, de la cinétique et du travail mécanique . À l’aide de ces données objectives, l’analyse de la marche permet une description précise de ses défauts conduisant à une analyse plus fine et un meilleur ciblage des stratégies thérapeutiques.
La coordination d’un grand nombre de muscles est nécessaire pour que le corps progresse en avant tout en se maintenant en équilibre et en limitant la dépense énergétique. Cependant, les degrés de liberté de l’activation musculaire ne corroborent pas ceux du centre de masse, puisque les schémas d’activation électromyographique (EMG) des membres inférieurs sont bien plus complexes qu’une simple alternance entre muscles fléchisseurs et extenseurs. Ils contribuent à générer le déplacement du centre de masse, celui-ci étant réduit à seulement deux degrés de liberté de mouvement linéaire (vertical et horizontal) . Pour que les schémas musculaires corroborent ceux du déplacement du centre de masse, différents chercheurs ont étudié la coordination inter-segmentaire des membres inférieurs. Certains ont utilisé la vitesse angulaire pour affiner la séquence temporelle des déplacements angulaires des segments pendant les phases du cycle de marche. Borghese et al., quant à eux, ont proposé une autre approche en montrant que l’évolution temporelle des angles d’élévation segmentaires des membres inférieurs (cuisse, mollet et pied), par rapport à la verticale et à un plan d’attraction, présentaient un schéma similaire chez différents sujets pour diverses situations, contrairement aux changements temporels des déplacements angulaires correspondants . Quand on affiche les angles d’élévation des trois segments sur un graphe 3D, on observe que les angles covarient dans un même plan en décrivant une boucle plus ou moins elliptique. Cette approche se nomme la covariation cinématique segmentaire (CCS) . Chez le sujet sain, la CCS suit une loi simple et invariable en fonction des différentes modalités de la marche ; par exemple, la covariation cinématique segmentaire des membres inférieurs en marche arrière est le reflet exact de la marche avant, alors que les caractéristiques mécaniques et les schémas d’activation musculaire sont totalement différents les uns des autres . La CCS peut également s’appliquer aux chats (marche quadrupède) et présente une similarité avec celle de l’homme (marche bipède), malgré d’importantes différences dans l’attitude posturale des deux espèces . De cette loi, découle une approche originale du mouvement humain, soutenant l’hypothèse que la covariation cinématique segmentaire est contrôlée par les générateurs centraux de rythme (Central Pattern Generators [CPG]) et reflète la coordination des membres inférieurs . L’application de cette loi de covariation a été étudiée pour quelques pathologies du système nerveux central. Grasso et al. ont rapporté une amélioration de la CCS chez les sujets souffrant de maladie de Parkinson idiopathique sous perfusion constante d’apomorphine ou sous électrostimulation des deux parties du globus pallidus. Dan et al. ont montré également une amélioration de la CCS après un bolus de baclofène (infusion intrathécale) chez un patient présentant une paraparésie spastique héréditaire. Plus récemment, une autre étude rapportait une amélioration de la CCS des deux membres inférieurs chez des patients hémiplégique spastiques, cela deux mois après des injections de toxine botulinique dans les muscles du membre parétique .
L’objectif de notre étude était d’évaluer l’impact d’une contrainte mécanique (orthèse) sur la covariation inter-segmentaire chez le patient hémiparétique. Lors d’une étude précédente, nous avions déjà étudié la pertinence d’une orthèse dynamique suropédieuse — l’orthèse Chignon ® , présentée en Fig. 1 — et d’une orthèse suropédieuse thermoformée dans la marche du patient hémiplégique en comparaison avec la marche sans orthèse . Nous avons observé une réduction significative du travail mécanique avec les deux orthèses et cela de façon similaire. L’orthèse Chignon ® améliorait de manière significative la vitesse de marche spontanée et la cinématique segmentaire de la cheville par rapport à l’orthèse thermoformée.
