Implant Fixation
Mick P. Kelly, MD
Brett R. Levine, MD, MS
Joshua J. Jacobs, MD
Dale Rick Sumner, PhD
INTRODUCTION
Total knee arthroplasty (TKA) relying on cement fixation remains the gold standard approach with proven long-term results.1,2,3,4 However, due to an increasing number of procedures performed in a younger, more active patient population combined with advancements in biomaterials, there is renewed interest in cementless TKA.5,6 Benefits of cementless fixation include potential for increased longevity, elimination of cement third-body wear, and perhaps decreased operative time. The purpose of this chapter is to describe the basic science of cemented and cementless fixation, with an emphasis on osseointegration in TKA. A thorough understanding of this topic is critical because it allows the surgeon to predict, recognize, and avoid common mechanisms of failure.
CEMENTED FIXATION
Basic Science of Polymethylmethacrylate
Bone cement comes in several different varieties that are typically supplied in a two-component system, a polymethylmethacrylate (PMMA) powder copolymer and a liquid methylmethacrylate monomer. Within the powder, there is typically an initiator (benzoyl peroxide), a radio-opacifier (barium sulfate,7 zirconium dioxide), possibly a coloring agent and/or an antibiotic. The liquid portion typically contains a stabilizer for the monomer (hydroquinone) and an accelerator (N,N-dimethyl para-toluidine, DMPT). Once the two components are mixed, the cement follows four stages as it sets up for use and will ultimately serve as a grout in aiding fixation of TKA components. The four phases are mixing, waiting, working, and hardening, and these may vary in length based upon the bone cement, local environment, and mixing process.8
There are numerous properties of bone cement that must be understood to evaluate all of the options currently available as well as to assure that it is utilized appropriately. One of the most commonly discussed properties of PMMA is viscosity, or the resistance to flow of a liquid, which can vary greatly based on the mixing method, molecular weight, liquid to powder ratio, and the addition of other materials to the cement (copolymers, antibiotics, etc.). High-viscosity cement typically has a shorter waiting phase and a longer working phase, which would seemingly be ideal for implanting TKA components.8 However, there are some reports and concerns that these “thicker” cement options may not penetrate into the cancellous bone as well, leading to the potential for higher failure rates.8,9,10 With modern cement techniques, it does not appear that these potential concerns have come to fruition in clinical practice with high-viscosity cement. Recently, Kelly et al confirmed this enthusiasm in regards to high-viscosity cement as its utilization has increased from 46% to 61.3% from 2012 to 2017,11 even though survivorship studies are still needed prior to encouraging greater adoption of high-viscosity cements. Despite the less-than-ideal mixing conditions with low-viscosity cement, there have been reports with greater success than with high-viscosity cement.11 An additional difference associated with cement viscosity includes the thermal energy produced during mixing and curing of the cement. High-viscosity cements tend to cure faster producing greater peak temperatures, while low-viscosity cements have lower heat release that lasts a longer time.8 In the end, there is no consensus and both appear to work well if the appropriate steps are taken during the cementation process during a TKA.
During the cementation process, it is critical for cement to penetrate into the cancellous bone. The deeper and more complete the interdigitation is at the cement-bone interface, the greater the overall strength of the construct. To aid in this process, cement can be injected into the bone using a cement gun or finger packed using manual pressure. Both are successful techniques if the bony surface is cleaned and dried in preparation for receiving a cemented implant. Pulsatile lavage, drying the bone, and drilling into sclerotic areas of bone can aid in cement penetration and are encouraged prior to applying cement to the surface of the cancellous bone.12,13 Typically, cement is quite strong in compression but is not as durable under shear forces, yet these properties can be greatly influenced by voids and deficiencies in the cement mantle. Therefore, meticulous cement preparation and mixing are critical and can intimately affect cement properties.
Mixing of cement can be performed by hand or under a vacuum preparation. The latter has been associated with decreased porosity and increased tensile strength.8 Other concerns with hand mixing have been reports of higher mortality and pulmonary embolism rates.8,14 Much of this data are based on case series in total hip arthroplasty but still remains a consideration in preparation for TKA. As mentioned previously, compounds can be added to PMMA as opacifying agents. Barium
sulfate is often utilized in this regard, yet increasing concentrations can lead to a reduction in shear strength and polymerization temperatures, with possible further decreases in fatigue, compressive, and transverse bending strengths.15,16 Alternatively, smaller size particles may aid in osteoblast adhesion as well as have a positive effect on fatigue strength.8 Modern cement preparations in the United States often involve Simplex (Stryker, Mahwah, NJ) or Palacos (Heraeus Medical LLC, Yardley, PA) formulations with other options from alternative vendors being available. Classically, Simplex is an example of a low-viscosity cement and Palacos is a high-viscosity preparation. Some of the specific properties of these two commonly used cements include opacifier—zirconium dioxide for Palacos and barium sulfate for Simplex; higher-molecular-weight polymer for Palacos versus lower for Simplex; sterilizing method—ethylene oxide for Palacos and Gamma radiation for Simplex; initial bending strength 87 versus 72 MPa and residual strength after cycling, 17.8 versus 14.2 MPa, both favoring Palacos over Simplex.17
sulfate is often utilized in this regard, yet increasing concentrations can lead to a reduction in shear strength and polymerization temperatures, with possible further decreases in fatigue, compressive, and transverse bending strengths.15,16 Alternatively, smaller size particles may aid in osteoblast adhesion as well as have a positive effect on fatigue strength.8 Modern cement preparations in the United States often involve Simplex (Stryker, Mahwah, NJ) or Palacos (Heraeus Medical LLC, Yardley, PA) formulations with other options from alternative vendors being available. Classically, Simplex is an example of a low-viscosity cement and Palacos is a high-viscosity preparation. Some of the specific properties of these two commonly used cements include opacifier—zirconium dioxide for Palacos and barium sulfate for Simplex; higher-molecular-weight polymer for Palacos versus lower for Simplex; sterilizing method—ethylene oxide for Palacos and Gamma radiation for Simplex; initial bending strength 87 versus 72 MPa and residual strength after cycling, 17.8 versus 14.2 MPa, both favoring Palacos over Simplex.17
The final potential advantage of utilizing bone cement in TKA is the ability to deliver local antibiotics within the cement. This remains a relatively controversial topic as there is no definitive data to support the routine use of antibiotic-laden bone cement in primary TKA. In selecting an ideal antibiotic, it has to be heat stable, water soluble, bactericidal (against the bacteria of choice), and have minimal effect on the mechanical strength of the cement. Balancing cost (antibiotic bone cement can be up to three times that of plain cement) versus efficacy is an important concern regarding adding antibiotics routinely to the bone cement in primary TKA. The Norwegian and Swedish registries have shown a reduced risk of periprosthetic infection when antibiotics are preloaded into the cement during total hip procedures.18,19 These early results have been favorable but must be weighed against the risks of compromised mechanical strength, drug-resistant bacteria, and hypersensitivity reactions.20,21 Alternatively, Hinarejos et al reported on 2948 cemented TKAs with 1465 receiving PMMA without antibiotics and 1483 with erythromycin- and colistin-loaded cement. The study was a prospective randomized trial in which they found no difference in deep (1.4% vs. 1.35%) or superficial (1.2% vs. 1.8%) infection rates with the control group compared to the antibiotic-PMMA cohort.22 Bohm et al. reported from the Canadian Joint Registry that 2-year revision rates were similar for those receiving antibiotic-loaded bone cement compared to those without antibiotics.23 This included over 36,000 cases, and the revision rate for infection was similar between the groups at this short-term follow-up. Despite the potential advantage of adding antibiotics, there has been a trend of decreasing utilization in the American Joint Registry ranging from 44.2% to 34.5% from 2012 to 2017.11 Some of the commonly used antibiotics for mixing with PMMA include vancomycin, gentamicin, tobramycin, ciprofloxacin, and voriconazole for fungal infections.
In regards to outcomes, cemented TKA remains the current gold standard. There are some recent studies that suggest equivalence between modern cementless versus cemented TKA. However, in general, the literature in the past has shown superior results with cemented implants as evidenced by the Swedish Registry reporting a 1.6× higher risk of revision if the tibial component is cementless.24,25,26 In general, cemented components show smaller migration distances and rates compared to uncemented tibial implants. Cemented implants are typically cheaper, more forgiving during insertion, and can be used for all patients requiring TKA.
CEMENTLESS FIXATION
Biology of Cementless Fixation
Cementless fixation involves a connection between newly formed bone tissue and the implant’s surface in contrast to cemented fixation, in which the polymer interdigitates with the surrounding trabecular bone to provide the bond between the host skeleton and the implant. An underlying assumption of the present chapter is that establishment and maintenance of a secure bony connection between the host skeleton and implant are prerequisites for long-term success. The theoretical advantage of cementless fixation is that the body relies on a self-repairing system of bone remodeling to maintain a mechanically competent interface, whereas with a cemented interface the mechanical properties of the polymer, if not the bone as well, would be expected to deteriorate with time.
Most of the initial studies of bone ingrowth in porous-coated implants indicated that bone ingrowth was a rare occurrence.27,28 However, other studies which include a significant number of implants retrieved at autopsy from patients who had well-functioning implants indicate that bone ingrowth occurs more reliably than originally thought.29,30 Even so, the topographic “coverage” by bone ingrowth typically involves less than one-third of the bone-contacting implant surface.29,30,31 Despite this limited amount of osseous fixation, studies have shown comparable mechanical stability of TKA implants fixed by bone ingrowth and cement32,33 and no differences in medium- to long-term survivorship based on revision for all causes in cemented versus cementless TKA in randomized controlled trials.34 A meta-analysis of 15 studies which included 5 randomized controlled trials and 10 other studies found better survivorship and less aseptic loosening with cemented implants; although if the analyses were restricted to the randomized controlled trials, there were no significant differences between cemented and cementless TKA with respect to survivorship or aseptic loosening.35
From a mechanical standpoint, the load at the fixation interface could exceed the strength of the connecting bone tissue, in which case, one would expect fixation failure. This can occur if the area of fixation is insufficient, in which case interface stresses can exceed the capacity of newly formed bone to support the implant. Thus, implant designs need to accommodate this factor by providing broad enough areas of fixation. On the biologic side, it would seem advantageous to have surgical techniques and, perhaps, biologically active agents to ensure broad areas of bone ingrowth/apposition. Data suggest that most, if not all, cases of late aseptic loosening actually represent failure to obtain fixation initially.36,37 Thus, the early mechanical and biologic events in cementless fixation are of critical importance.
The surgically induced trauma created at the time of implantation provides the context in which cementless fixation occurs.38 The skeleton mounts a response leading to woven bone formation after hematoma formation and mesenchymal tissue development (Fig. 14-1). In this process of intramembranous bone regeneration, lamellar bone eventually forms on the spicules of woven bone and the hematopoietic marrow is reestablished. Under the correct conditions, this biologic response can result in the development of a mechanically competent connection between the implant’s surface and host bone (Fig. 14-2).
After surgically induced intramembranous bone regeneration in sites where bone is not normally found (i.e., the diaphysis of a long bone), the newly formed bone will be completely resorbed and replaced by marrow unless an implant is present to provide a mechanical reason for the new bone to persist. In the context of knee arthroplasty, where the implants are placed in a trabecular bone bed, one would expect long-term persistence and functional adaptation of the newly formed bone tissue because fixation to the host skeleton imparts a mechanical function to the new tissue. Therefore, the distribution or amount of bone ingrowth would be expected to change over time and reflect areas of stress transfer from the implant to the host skeleton. Adaptation of the bone in periprosthetic regions has been identified in the context of knee replacement and is suspected to reflect changes in the local mechanical environment.39,40,41
The osteogenic potential of the implantation site would seem to be an important factor in cementless fixation. For instance, osteogenesis after skeletal trauma would be inhibited in the absence of appropriate stem cells. These cells are thought to reside in the marrow, especially in the region near the endosteum.42,43,44 Although skeletal stem cells can be isolated from multiple anatomic locations, including bone marrow, periosteum, skeletal muscle, fat, and umbilical cord blood,45 the general consensus is that only cells recruited locally serve as bone cell progenitors during bone repair.46 If the local source-only concept for bone repair is correct, then decreased availability of stem cells is likely after aseptic loosening of cemented implants, where the marrow spaces are filled by granuloma rather than normal marrow tissue, and may account for decreased bone formation in models of revision arthroplasty.47,48 The issue of compromised osteogenic potential of stem cells as a function of aging is controversial.49,50,51,52,53,54,55 Currently, there is limited clear-cut evidence of decreased osteogenesis in response to skeletal trauma in the aged skeleton.56 However, diminished mechanical fixation related to osteopenia may pose problems.57
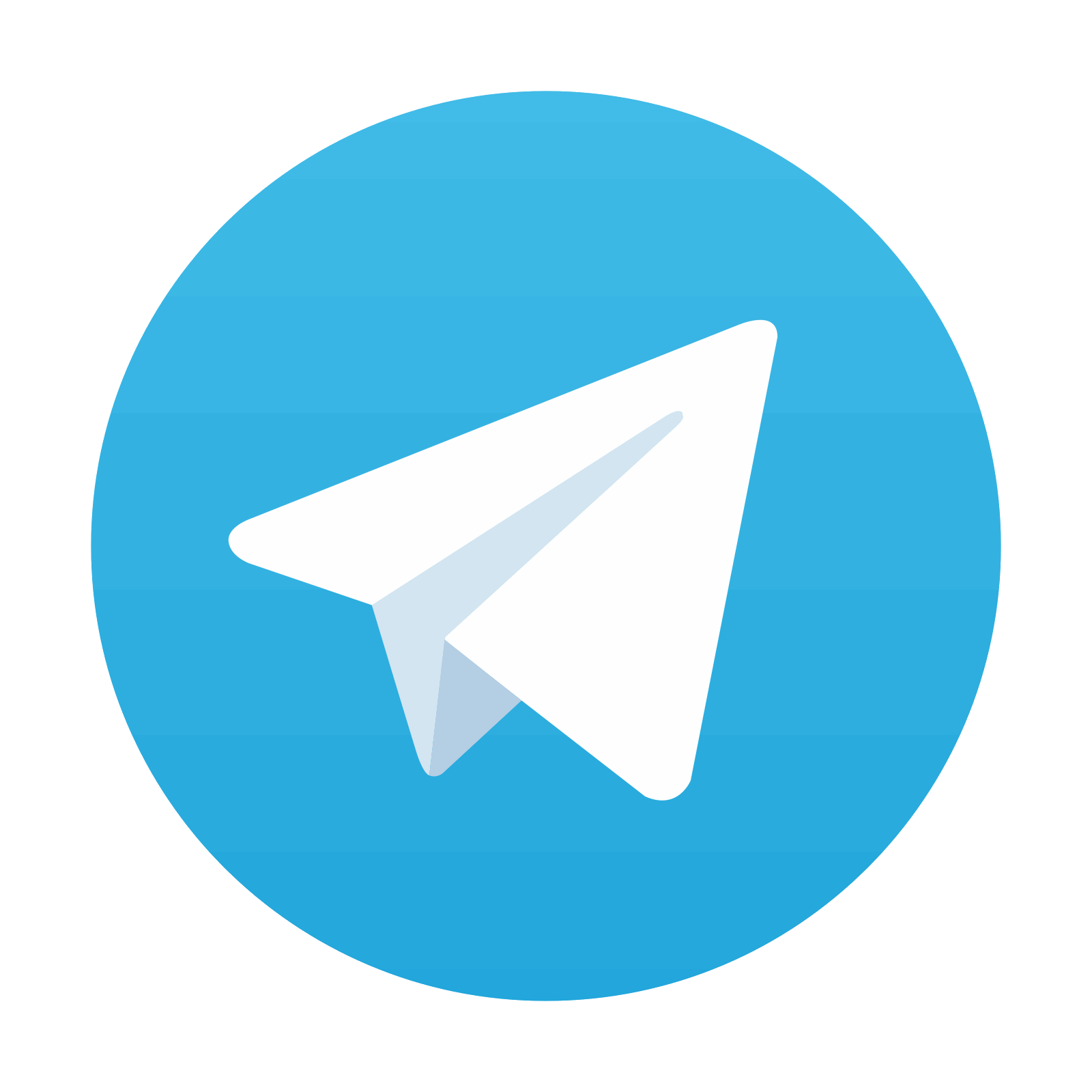
Stay updated, free articles. Join our Telegram channel

Full access? Get Clinical Tree
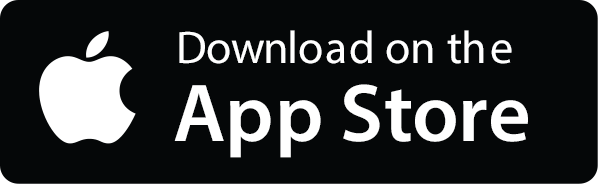
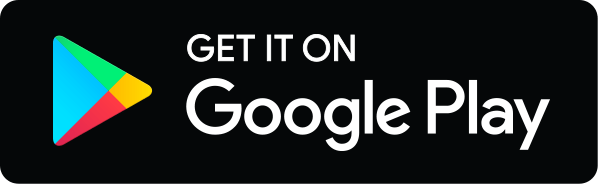