Key points
- •
Various imaging modalities exist to assess the intervertebral disc.
- •
Classification grading schemes have been proposed to assess the morphological and structural changes of the disc.
- •
Novel or nontraditional imaging platforms have been developed to assess early disc changes, biochemical profiles of the disc and assist in identifying painful discs.
- •
Clinical studies are needed to assess the efficacy of all imaging technologies in relation to identifying problematic discs, monitoring their profiles, and providing insight in clinical decision-making.
Acknowledgments
Research reported in this publication was supported by the National Institute of Biomedical Imaging and Bioengineering of the National Institutes of Health under award Number P41EB015893.
Introduction
Low back pain (LBP) is one of the most common reasons for all physician visits in the United States [ ] with an estimated annual direct/indirect healthcare costs of over $100 billion USD [ ]. The most common cause of LBP among adults is degeneration of the intervertebral disc (IVD) in the spine [ ] (see Chapter 1 ). Diagnostic imaging technologies for spinal discs can readily detect disc bulges, vertebral endplate changes, canal stenosis, and mild spondylolisthesis associated with normal aging [ , ] (see Chapter 6, Chapter 7, Chapter 8, Chapter 9, Chapter 10, Chapter 11 ). These methods are routinely utilized for surgical planning and to categorize patients into those with nerve root pain (radiculopathy) or spinal pathology such as stenosis, or another specific spinal cause such as a tumor or infection. However, the majority of chronic low back pain patients fall into the third category of nonspecific low back pain, where no specific pathology can be identified and advanced diagnostic imaging techniques do not improve outcomes [ ]. Unnecessary diagnostic imaging can lead to an unintended cascade of events initiated by a positive test that may produce more unexpected results. Either due to patient or physician anxiety, such a situation typically results in further ill-advised tests or surgical procedures that may cause avoidable adverse effects and morbidity [ ]. Hence, the current medical consensus recommends against the routine use of advanced imaging except in cases of suspected serious pathology, and when conservative care is unsuccessful [ , ].
Patient outcomes could be significantly improved by reducing unnecessary tests and unsuccessful treatments [ ]. To this end, there needs to be a paradigm shift from testing and treating late-stage spinal disc pathologies to techniques and methods that can detect the early changes, perhaps even biochemical changes, that are expected to occur before gross morphological changes are readily visible in routine diagnostic approaches. Information about the early-stage disease can also be used to measure treatment efficacy in clinical trials.
The following sections describe imaging approaches for the detection of disc pathology by reviewing morphological imaging techniques such as plain radiographs (X-ray), computed tomography (CT) imaging, and conventional magnetic resonance imaging (MRI) approaches. Then, the novel MRI-based methods that are sensitive to biochemical abnormalities of the disc are described. As these latter approaches could be used to monitor disease progression of the disc as well as gauge the effect of biological therapies, their potential as clinical imaging biomarkers is also discussed.
Radiographic imaging
Radiographic imaging of the IVD is routinely used for the classification of surgical indications, erosion of the margins of the vertebral plates, degree of osteophyte formation, and narrowing of the disc space in clinical diagnoses [ ]. Imaging planes for initial screening in the clinic are typically in lateral or anteroposterior (A-P) directions to visualize the entire spine and to detect physical deformities in patients. This also facilitates a more quantitative approach by grading disc degeneration as described by Kellgren et al. [ ]. While MRI has replaced plain film radiography to visualize the IVD directly, grading schemes based on plain radiographs have certain advantages. X-ray technology is more widely available and more economical. Furthermore, radiography of the spine is routine for diagnostic or follow-up purposes and hence digital plain films are easily accessible for analysis [ ]. Additional information regarding the stage of disc degeneration (presence of clefts in the nucleus or fissures of the annulus, etc.) can be detected using a discogram, where a radio-opaque dye is injected into the discs [ ] and the resulting image is graded according to a scale ( Fig. 5.1 ). However, the lack of soft-tissue contrast and the requirement the injection of contrast, is a fundamental limitation of radiographic imaging and discogram approaches. Hence CT and MRI have become widely used for diagnosing IVD abnormalities, and the latter has become the most commonly used imaging modality in the diagnosis of degenerative disc disease (DDD) [ ].

Computed tomography
CT discography has been used to improve surgical outcomes [ ]. However, it is an invasive technique due to its use of radiation and some cases require the placement of needles through the annular fibers and injection of contrast, and there are high incidences of false positives [ , ]. While CT can accurately visualize disc morphology, it fails to distinguish findings that are symptomatic from those that are incidental. Hence, CT fails to demonstrate the cause of pain in patients without obvious nerve root compression. “Dynamic” CT can be employed to accurately measure ranges of motion of vertebrae when a load or torque is applied, in vivo. This technique is made possible due to the advent of high-speed capability and image processing tools in CT scanners [ ].
MRI methods to investigate morphology
MRI is a diagnostic imaging method that utilizes radiofrequency waves to excite and detect a magnetic signal from the human body. Unlike other imaging modalities like X-rays and CT, MRI uses no ionizing radiation. For this reason, MRI can be used repeatedly on healthy individuals and is uniquely suited for clinical research.
With its excellent soft-tissue contrast, MRI is routinely utilized for characterizing the disc morphology and function. The standard MRI protocol includes a series of pulse sequences, which are timings of application of various radiofrequency pulses and magnetic gradients pulses for preparation and spatial localization of the MRI signal, respectively [ ]. Most clinical MRI protocols for DDD will include some combination of T 1 -weighted and T 2 -weighted fast (or turbo) spin-echo in both sagittal and axial planes to visualize the entire spine or individual discs, respectively. This basic protocol can be modified to include a short tau inversion recovery sequence for enhancing the dynamic range of non-CSF tissue, or images acquired in the coronal plane [ ].
The nucleus pulposus (NP) appears bright in the T 2 -weighted images, whereas the annulus fibrosus (AF) is invisible due to its short T 2 in normal discs. IVD degeneration can be qualitatively graded by quantifying the reduction in signal intensity of the NP [ ], while in advanced stages of degeneration, there is no clear distinction between NP and AF. The widely used classification system of Pfirrmann [ ] is based on T 2 -weighted MR images. An integer grade (between I and V) is assigned to the disc, based on structural morphology (e.g., homogeneity within the NP, distinction between the NP and AF, signal intensity, and disc height). Although this classification system is among the most widely accepted and used [ ] and provides excellent detection of advanced stage degeneration, integer-based classification systems cannot discriminate among early degenerative changes, suffer from observer bias, and are not specific for disc substructures [ , ]. There is little correlation with presence or severity of clinical symptoms such as pain, hence the usefulness of Pfirrmann grading in clinical decision-making is dependent upon correlations with other clinical and radiographic findings.
Measurement of MRI relaxation times can help reduce some of the issues with qualitative MRI reads and user-biased quantification approaches. In these approaches, the actual T 1 , T 2 , or other MR property of water in the tissues is quantified by the use of an appropriate pulse sequence [ ]. The discriminating power of these quantitative MRI approaches for IVD characterizing IVDs in individuals and in cohorts is dependent on knowing the reproducibility of repeat measurements and its relation to the expected tissue differences due to disease. Several studies have measured T 1 and T 2 relaxation times and the diffusion coefficient of water to improve the capability of MRI-based methods to objectively quantify disc degeneration. In ex vivo disc specimens [ ], where correlations were observed between T 2 and water content and noted significant differences in relaxation rates between human NP and AF [ ]. And although an in vivo study [ ] found no correlation between proton density and age, there were significant differences in T 1 and T 2 between normal and degenerated IVDs. However, a later study [ ] showed that the differences were only 196 ms for T 1 and 15 ms for T 2 and reproducibility for the measurements was low, at 16.4% and 13.4%, respectively. Diurnal variations in T 2 due to changing water content from morning to evening in the same individual would further confound T 2 measurements. However, quantitative T 2 measurements may have a clinical relevance if diurnal differences were to be found between cohorts of patients with back pain and asymptomatic controls, for example [ ]. Significant decreases in these parameters were observed in both NP and AF compartments with an increase in Thompson grade and loading in IVD specimens [ ]. A decrease in the diffusion of water in the nucleus was detected by diffusion-weighted MRI with reduction of proteoglycan ex vivo [ ], and with degeneration in vivo compared to healthy controls [ , ]. However, diffusion-weighted MR images suffer from low SNR and resolution and are susceptible to motion artifacts and difficult to reproduce measurements in vivo.
Novel MRI techniques for disc degeneration
A major drawback of conventional MRI methods is that while they effectively detect morphological changes in the disc, they lack sensitivity to the early stages of disc degeneration. While in its late stages, DDD is characterized by a loss of disc height, annular tears and rim lesions, and osteophyte formation [ ]. The early stages are characterized by loss of glycosaminoglycan (GAG) in the NP resulting in reduced capacity to bind water and a loss of hydration and pressure in the disc [ , ]. Disc degeneration has been implicated as a potential source of low back pain [ ]. Marked compositional changes in total collagen content increase, and the distribution of collagen types also occurs with the disease [ , ].
Hence, newer MRI-based methods have approached the diagnostic process by attempting to detect and quantify the biochemical composition of the extracellular matrix of the IVD since changes in NP and AF composition and structure are the earliest changes in disc degeneration. Subsequently, the following methods have evolved over the last few years from several research groups that are specifically working in developing these methods for eventual application in the clinics.
T 1Ρ MRI
T-1-rho” MRI is an alternative to conventional T 1 and T 2 MRI [ ] in which a long-duration, low-power radiofrequency (RF) referred to as “spin-lock” (SL) pulse is applied to the magnetization in the transverse plane. The magnetization is spin-locked and undergoes relaxation in the presence of an RF field (B 1 ) in the rotating frame, a situation similar to that of the longitudinal magnetization in the main magnetic (B 0 ) field. The spin-locked magnetization will relax with a time constant T 1ρ , called the spin-lattice relaxation in the rotating frame, during the B 1 field that attenuates the effect of signal loss mechanisms (i.e., dipolar relaxation, static dipolar coupling, chemical exchange, and background gradients) on the MRI signal [ ]. For this reason, T 1ρ is always greater than T 2 . In a typical T 1ρ mapping experiment, the duration of the SL pulse is incremented while the amplitude of the SL pulse (γB 1 ~0.1-few kHz) is fixed. T 1ρ MRI has recently been used as a biomarker for symptomatic disc degeneration with low values correlating with higher degeneration, low PG content, and low swelling pressure in the NP [ , , , ].
Degeneration of the AF may be the source of back pain in patients with DDD as annular fissures can be innervated [ ]. Unfortunately, the AF appears dark in conventional MRI due to the nonaveraged dipolar interaction of water bound to highly oriented collagen fibers, which shorten T 2 . The effect of spin-locking on the laminar appearance in MRI of cartilage using orientation-dependent studies was previously investigated where it was found that T 1ρ values were greater than T 2 at all orientations throughout the cartilage layers [ ]. Validation of T 1ρ as a biomarker for GAG proteoglycan was recently demonstrated by Mulligan et al. [ ]. T 1ρ was measured in IVDs obtained from harvested human lumbar spines and analyzed by comparing to Pfirrmann grades obtained from corresponding T 2 -weighted MRIs ( Fig. 5.2 ) as well as GAG content measure by biochemical assay. T 1ρ values provided a better assessment of disc degeneration as it correlated with GAG concentration.

T 1ρ MRI has been used to study the variation in the IVD in normal adults (34 men and 29 women) in their twenties [ ]. T 1ρ values (in milliseconds) were significantly higher in the NP than in the AF at all disc levels. T 1ρ values were significantly lower in females at L3–L4 and L4–L5 discs compared to males suggesting that disc degeneration may occur earlier in females. In another study, LBP patients received multilevel provocative discography before their MRI and disc opening pressure (OP), when the injected fluid first enters the nucleus of the IVD, was recorded before T 1ρ MRI. The results demonstrated a strong correlation between T 1ρ values and in vivo OP measurements in LBP patients, and T 1ρ was significantly lower in painful discs [ ]. A recent study revealed the potential role of T 1ρ MRI in the assessment of early degenerative changes occurring in IVDs of young asymptomatic weightlifters compared with a healthy control group matched for age. Lower T 1ρ values were observed in discs of weightlifters compared to nonweightlifters ( Fig. 5.2 ): Representative examples of midaxial and corresponding sagittal T 1ρ images across age and severity of degeneration as classified by Pfirrmann grading intervertebral disc. The dashed circles indicate where the biopsy punches were performed for assessing proteoglycan concentrations in the NP [ ]. Fig. 5.3 illustrates images that corresponded to previous studies that had demonstrated that weightlifters have a higher lifetime risk of specific spinal pathologies correlated with loading.

Magnetization transfer MRI
The magnetization transfer (MT) pulse sequence employs a saturation pulse to selectively saturate magnetization, and hence the MRI signal, from water bound to macromolecules such as collagen [ ]. MT ratio (MTR) maps can be generated from two images that are collected, with MT preparation (M s ) and without (M 0 ). By setting the off- resonance saturation pulse at 6.4 kHz down field of the free water proton resonance frequency provides MTR maps ( Fig. 5.4 ). A proton-density MRI depicts differences between a 30-y.o. healthy individuals lumbar discs compared to a 69 y.o. asymptomatic subject who exhibits decreased disc height, loss of NP hydration, and herniation in the lower lumbar IVDs. The corresponding MTR maps (in color) showed increased values in the NP of the 69-year-old subject while there is a clear delineation of AF-NP boundary in the 30-year-old subject. High MTR values (in white) indicate intact collagen in the AF of the younger individual. A closer look at the MTR maps of the L2–L3 disc, for example, demonstrates a distinct boundary between MTR values within the NP and AF of the 30-year-old subject, while it diffuses in the 69-year-old subject, indicating loss of collagen architecture in the AF and infiltration of collagen into the NP indicating a disruption of the boundary between these two tissue types [ ]. While MT MRI can provide information on the collagen architecture, the early degenerative changes that could provide a better predictor of disc disease need more sensitivity to the GAG content in the nucleus of the IVD. Furthermore, the broad MR spectral lines of intrafibrillar water magnetization are prone to a “direct saturation effect” from the saturation pulse even if it is applied at several kilohertz off-resonance, reducing the sensitivity of MT MRI to the GAG content in the NP. Hence, researchers have instead pursued a similar method called chemical exchange saturation transfer (CEST) MRI, which is also based on the MT effect but with higher sensitivity to [GAG] in the NP.

Chemical exchange saturation transfer MRI
CEST is a method to indirectly detect exchangeable solute protons in tissues by constant irradiation and saturation of their chemically shifted magnetization [ , ]. The CEST signal is detected as a reduction in the water signal after the saturated spins undergo chemical exchange with water [ ], provided the exchange is more rapid than the T 1 of either site. A simple difference image obtained after saturating symmetrically on either side of the water yields an MTR asym map reflecting the distribution of labile protons around the central water peak:
MTRasym(Δω)=S(−Δω)−S(Δω)S0
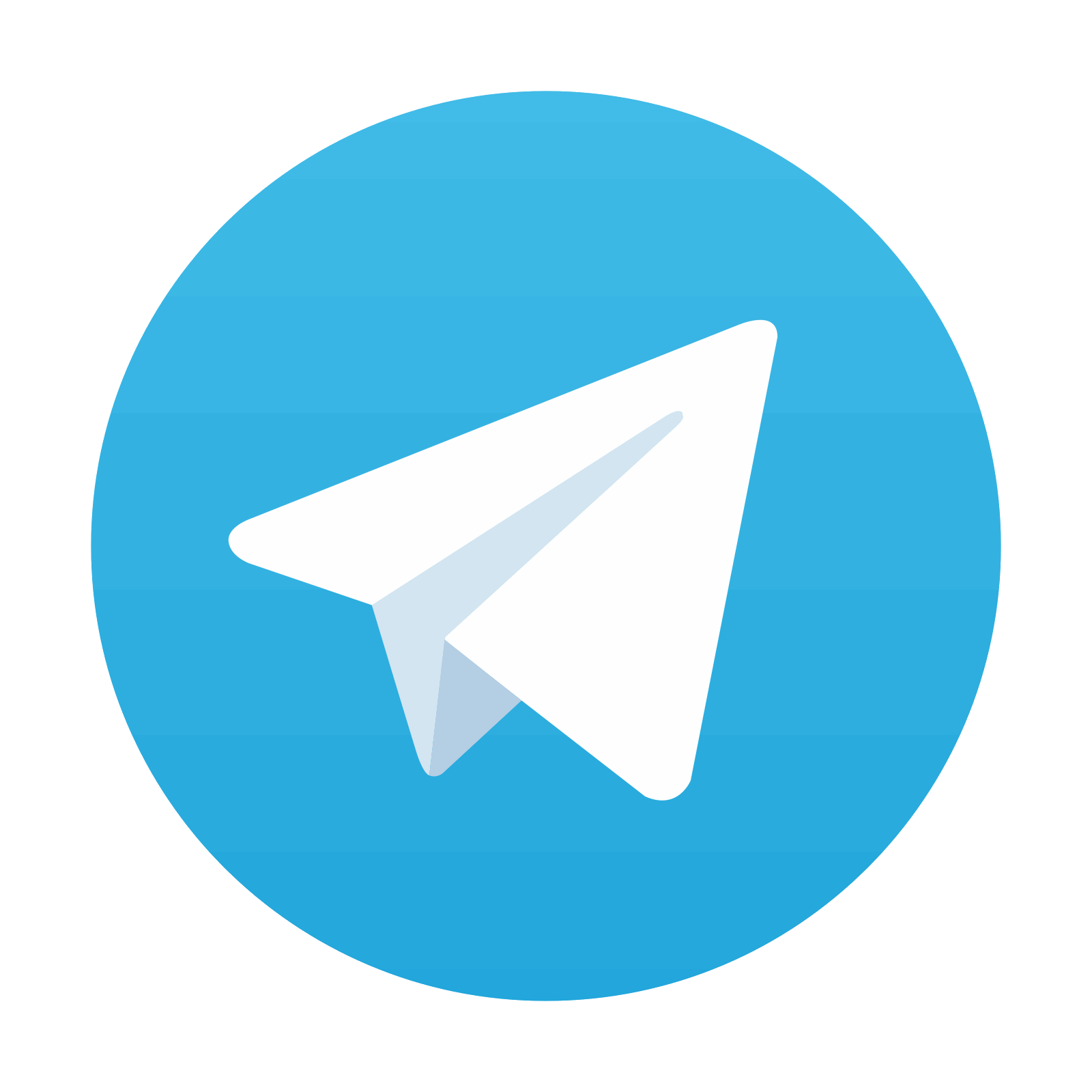
Stay updated, free articles. Join our Telegram channel

Full access? Get Clinical Tree
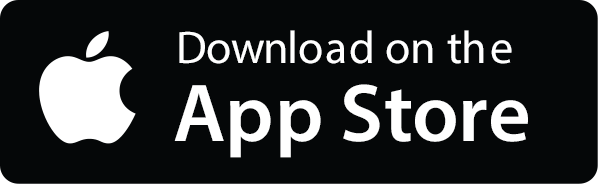
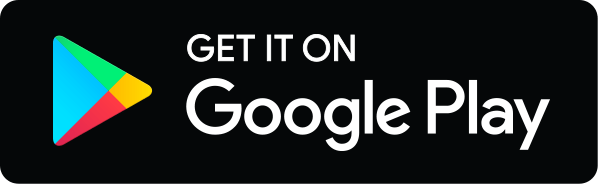
