Imaging of the Native and Prosthetic Knee
Alissa J. Burge, MD
Hollis G. Potter, MD
INTRODUCTION
Determination of an appropriate imaging algorithm for evaluation of the knee depends on a number of factors. A variety of imaging modalities are available, each of which complements the others in terms of which tissues are best evaluated. Considerations when selecting an imaging modality include clinical and surgical history, as well as the suspected pathology and types of tissue that may be involved.
CONVENTIONAL RADIOGRAPHS
Standard Views
Radiographs are commonly the first imaging modality obtained for evaluation of the knee, being relatively easily obtained, and providing global assessment of the osseous anatomy, and to an extent, the soft-tissue structures. Standard examinations typically include weight-bearing anteroposterior (AP) and lateral views, with additional views added as warranted by the clinical concern. Routine AP views may demonstrate evidence of osteoarthritis, such as joint space narrowing and marginal osteophytes, while lateral views, which are typically obtained non-weight-bearing in 30° of flexion, allow evaluation of the anterior structures such as the extensor mechanism and suprapatellar region. Tangential views of the patellofemoral compartment are often included in the routine radiographic examination of the knees, allowing demonstration of patellofemoral alignment and joint space narrowing.1
Additional Views
Posteroanterior (PA) flexion views of the knee may be obtained for more sensitive evaluation of the posterior aspect of the femorotibial compartments and are useful for demonstrating chondral loss preferentially affecting the posterior aspect of the joint (Fig. 16-1).1
The tunnel view is obtained supine with the knee in flexion and the beam directed inferiorly perpendicular to the tibia, in order to best demonstrate the intercondylar notch. This view is useful for detecting pathology within the notch, such as loose bodies, tibial spine avulsions, and osteochondral lesions along the inner aspects of the condyles.1
The cross table lateral view is obtained with the patient supine and the leg extended; this view is often obtained in the patient who have suffered acute trauma and are unable to bear weight and may demonstrate a fat-fluid level within the suprapatellar region, indicative of lipohemarthrosis in the setting of an intra-articular fracture.1
COMPUTED TOMOGRAPHY
Computed tomography (CT) has been supplanted by magnetic resonance imaging (MRI) for many indications in evaluation of the knee; however, CT remains the gold standard evaluation of mineralized bone and fine details of osseous anatomy. CT also provides an alternative cross-sectional modality for evaluation of patients with contraindications to MRI, such as those with pacemakers or severe claustrophobia.
Routine CT evaluation of the knee is generally performed with the patient in the supine position and the knee extended, with the field of view extending from the supracondylar region of the femur through the proximal tibia utilizing submillimeter axial slices and reformats constructed in three planes. Reformatted images may also be constructed at unique obliquities in order to optimally demonstrate pathology, and 3D reformats are useful for a more global depiction of the knee. CT arthrography may be performed following intra-articular injection of iodinated contrast and air, in order to assess for soft-tissue pathology in patients unable to undergo MRI.2
One of the most common indications for CT evaluation of the knee is preoperative assessment of fracture morphology. CT provides accurate characterization of the degree of comminution, areas of cortical and articular surface displacement, and small ossific fragments within the joint space. CT is also useful for preoperative templating of custom arthroplasty implants, in which the implant is specifically tailored to the patient’s individual anatomy.3,4 In patients with painful total knee arthroplasty (TKA), modification of scan parameters allows reduction of metal artifact and improved visualization of the tissues surrounding the implant.5 CT may demonstrate areas of osseous resorption, as may be seen in the setting of polymeric wear or mechanical
component loosening. CT arthrography may demonstrate meniscal tears and chondral defects in native knees, as well as areas of synovial scarring in patients following TKA (Fig. 16-2).
component loosening. CT arthrography may demonstrate meniscal tears and chondral defects in native knees, as well as areas of synovial scarring in patients following TKA (Fig. 16-2).
NUCLEAR SCINTIGRAPHY
Much like CT, nuclear scintigraphy has largely been supplanted by MRI for most indications in the knee; however, nuclear imaging may be useful in patients with
contraindications to MRI, as well as for evaluation of suspected malignancy. Bone scans utilizing technetium-labeled bisphosphonate compounds detect areas of increased osteoblastic activity and therefore are useful in evaluation of conditions involving greater than normal osseous remodeling, such as fractures and stress reactions, neoplasm, and arthroplasty loosening (Fig. 16-3).6,7 Other radiotracers are utilized for various specialized indications; for example, gallium and indium scans are useful in the setting of suspected infection. While highly sensitive, nuclear studies are generally of lower resolution than other modalities and are therefore generally less specific. Utilizing nuclear imaging in conjunction with other modalities may be useful in increasing the diagnostic specificities of these scans.
contraindications to MRI, as well as for evaluation of suspected malignancy. Bone scans utilizing technetium-labeled bisphosphonate compounds detect areas of increased osteoblastic activity and therefore are useful in evaluation of conditions involving greater than normal osseous remodeling, such as fractures and stress reactions, neoplasm, and arthroplasty loosening (Fig. 16-3).6,7 Other radiotracers are utilized for various specialized indications; for example, gallium and indium scans are useful in the setting of suspected infection. While highly sensitive, nuclear studies are generally of lower resolution than other modalities and are therefore generally less specific. Utilizing nuclear imaging in conjunction with other modalities may be useful in increasing the diagnostic specificities of these scans.
Following initial placement of TKA components, periprosthetic uptake of radiotracer may persist for approximately 1 year.8 During this time period in particular, scanning utilizing a combination of radiotracers may yield improved diagnostic accuracy over scans utilizing a single tracer. These scans may be a useful data point in identifying implant loosening. Additionally, in patients with suspected infection, a combination leukocyte and sulfur colloid marrow scan provides increased specificity over either scan alone, as infection will manifest as increased uptake on the leukocyte scan without a concurrent increase in uptake of sulfur colloid, which accumulates in areas of altered marrow distribution; this combined technique results in accuracy of approximately 90% for diagnosis of infection.9,10,11 It is important to point out that while these scans alone do not confirm periprosthetic joint infection, they may be one of multiple data points needed to confirm this diagnosis.
ULTRASOUND
Basic Principles
In addition to providing high-resolution images of the more superficial structures about the knee, ultrasound provides the additional benefits of potential dynamic evaluation and the performance of image-guided procedures. Evaluation of musculoskeletal structures is typically best performed with a linear array transducer of medium to high frequency, depending on the size and depth of the structure of interest.
Diagnostic Imaging
The more superficial tendons and ligaments about the knee are easily visualized with ultrasound.12 Normal tendons and ligaments appear overall hyperechoic, with longitudinally oriented fibers resulting in a fibrillar internal architecture which is clearly visible on ultrasound. Tendinosis manifests as areas of hypoechogenicity, enlargement, and loss of normal fibrillar architecture; however, care must be taken to maintain the probe at an orientation perpendicular to the structure being interrogated, in order to
avoid mistaking the effects of anisotropy for pathology. Frank tears appear as disruption of normal fibers with intervening fluid, often superimposed upon degeneration (Fig. 16-4).13,14
avoid mistaking the effects of anisotropy for pathology. Frank tears appear as disruption of normal fibers with intervening fluid, often superimposed upon degeneration (Fig. 16-4).13,14
Synovial expansion is also often easily appreciated on ultrasound, particularly in the suprapatellar region. Doppler imaging may detect areas of hyperemia within inflamed synovium. Popliteal cysts in arthritic knees are a common cause of posterior knee discomfort and are easily visualized on ultrasound. In patients with TKA, ultrasound is particularly useful in assessment of patellar clunk, in which a nodule of scarred synovium along the patellofemoral articulation results in mechanical symptoms during range of motion. Dynamic evaluation allows visualization of the motion of the scar nodule during flexion and extension, offering confirmation that the scar is the cause of the patients’ symptoms.15
Neurovascular structures are well-appreciated sonographically, provided they are relatively superficially positioned. Doppler evaluation of the popliteal vein is commonly performed to assess for deep venous thrombosis. Arterial abnormalities such as stenosis and pseudoaneurysm are also easily appreciated, and Doppler interrogation can provide waveform assessment for evaluation of vascular flow, potentially providing evidence of abnormalities both at and remote from the site of interrogation.16 Many of the major nerves about the knee are superficially positioned and therefore quite amenable to sonographic evaluation.17 Focal lesions such as neuromas and peripheral nerve sheath tumors are easily visualized. Areas of scar entrapment of extrinsic compression may also be identified. Nerves, much like tendons, are typically hyperechoic with internal longitudinal fascicular architecture; neuritis may manifest as fascicular swelling and hypoechogenicity, while injury may manifest as fascicular disruption.18
Ultrasound-Guided Intervention
Ultrasound is particularly well-suited for image-guided interventions.19 While knee joint aspirations and steroid injections may be performed blindly, imaging guidance is often useful in patients with difficult anatomy. More complex procedures about the knee generally benefit from imaging guidance. Popliteal cyst aspirations are performed quite commonly under ultrasound, which allows visualization of cyst size and the degree of loculation, and confirmation of cyst collapse (Fig. 16-5). Perineural injections may be performed both as a treatment for neuropathic pain, as well as for anesthesia prior to surgical intervention.17,20 Calcium hydroxyapatite deposition, while less common than in the shoulder, may occur in the knee and is amenable to ultrasound-guided lavage. Ultrasound injection of platelet rich
plasma (PRP) may be used as a treatment for tendinosis and tears, particularly those involving the extensor tendons.
plasma (PRP) may be used as a treatment for tendinosis and tears, particularly those involving the extensor tendons.
BASIC PRINCIPLES OF MAGNETIC RESONANCE IMAGING
Magnetic resonance imaging (MRI) provides excellent soft-tissue contrast as well as sensitivity for marrow changes and has therefore supplanted many traditional imaging techniques for evaluation of soft tissue and marrow within the knee.
Magnetic resonance imaging exploits the ability of spinning atomic species possessing an odd number of nucleons to induce a local magnetic field. 1H, containing a single proton, is the isotope upon which the vast majority of clinical MR imaging is based, due to its pervasiveness within biological tissues. Upon application of an external magnetic field, Bo, the normally randomly oriented hydrogen nuclei align their spins parallel to the long axis of the field, yielding a net magnetic vector, Mz, along the longitudinal axis of the field. While spinning, these nuclei simultaneously precess about the axis of the external magnetic field with a motion like that of a gyroscope, at a specific frequency which is determined by the field strength of Bo. When protons are aligned with Bo, this precessional motion is not synchronized among individual nuclei, therefore while a net magnetization vector is created in the longitudinal direction, no net magnetization vector is generated in the transverse plane. Through application of a brief radiofrequency pulse oriented at 90° to the longitudinal axis of the main magnetic field, the protons are raised to a higher energy state in which they are rotating in the transverse plane about the axis of the main magnetic field in a synchronized fashion, referred to as the state of being “in phase” with one another. This synchronicity results in the generation of a net transverse magnetization vector, Mxy, which allows transmission of the MR signal via the induction of magnetic flux within the receiver coil; the rotation of the nuclei in the transverse plane induces an alternating current within the receiver coil, which is subsequently subjected to series of digital manipulations in order to eventually form an image. Upon termination of the 90° RF pulse, the transverse magnetization vector decays via two simultaneous but independent processes: recovery of magnetization in the longitudinal axis (spin lattice, or T1, relaxation) and dephasing of rotation in the transverse plane (spin-spin, or T2, decay). These processes form the basis of MR tissue contrast, as the time required for each process depends upon the biochemical environment of each individual proton, thereby determining its signal characteristics on images generated by a given pulse sequence.21
Deliberate modification of pulse sequence acquisition parameters produces different types of soft-tissue contrast within the generated images, a concept referred to as image weighting. The main acquisition parameters contributing to image weighting are the interval between RF pulses (time to repetition, TR) and the interval from RF pulse to the sampled signal, or echo (time to echo, TE). Pulse sequences commonly employed for musculoskeletal MR imaging typically yield T1-weighted, T2-weighted, and proton density (PD)-weighted images and are obtained utilizing specific combinations of long and short TRs and TEs. Fat-suppressed, fluid-sensitive images allow sensitive detection of mobile water and are generally a part of routine clinical imaging algorithms. Knowledge of the expected signal characteristics and appearance of normal anatomic structures upon differently weighted images is key in image interpretation, facilitating recognition of pathology.21
The choice of optimal MR imaging sequences for a given algorithm depends on the body part and tissues being imaged. For orthopedic imaging, proton density (PD)-weighted fast spin echo (FSE) sequences provide excellent contrast between musculoskeletal tissues, allowing delineation of the major tissue types within the knee. The addition of fat-suppressed fluid-sensitive images
allows detection of fluid and edema. These sequences constitute the foundation of the majority of clinical imaging studies of the knee performed at the authors’ institution, though additional sequences may be added on a per case basis (Fig. 16-6). For example, gradient echo images allow more sensitive detection of hemorrhagic products and are therefore commonly added for evaluation of patients with known or suspected pigmented villonodular synovitis (Fig. 16-7).22 Imaging of the knee is typically performed with the patient in a supine position, utilizing a dedicated extremity coil.
allows detection of fluid and edema. These sequences constitute the foundation of the majority of clinical imaging studies of the knee performed at the authors’ institution, though additional sequences may be added on a per case basis (Fig. 16-6). For example, gradient echo images allow more sensitive detection of hemorrhagic products and are therefore commonly added for evaluation of patients with known or suspected pigmented villonodular synovitis (Fig. 16-7).22 Imaging of the knee is typically performed with the patient in a supine position, utilizing a dedicated extremity coil.
MENISCI
Normal Meniscal Anatomy and Imaging
Normal meniscal tissue appears uniformly hypointense on all conventional MR pulse sequences, due to its being composed of highly ordered fibrocartilage, resulting in short relaxation times. Menisci are typically best visualized on coronal and sagittal images, with the anterior and posterior horns well seen on sagittal images, and the body segments best seen on coronal images (Fig. 16-8). The medial meniscus is larger than the lateral and has a more open C shape, with the posterior horn being generally larger than the anterior horn, while the lateral meniscus has a more circular configuration, and the horns are more uniform in size. The transverse meniscal ligament, which joins the anterior horns of both menisci, is also well seen on sagittal images, as are the meniscofemoral ligaments of Wrisberg and Humphrey, which extend from the medial femoral condyle to the posterior horn of the lateral meniscus.23,24
Meniscal Pathology
Meniscal degeneration results in decreased organization of collagen fibers and increases in mobile water content,
resulting in increased signal relative to normal meniscal tissue. The presence of a frank meniscal tear is indicated by linear signal hyperintensity traversing the substance of the meniscus and contacting an articular surface, and/or changes in meniscal morphology. Meniscal tears are classified by their orientation with regard to the substance of the meniscus.
resulting in increased signal relative to normal meniscal tissue. The presence of a frank meniscal tear is indicated by linear signal hyperintensity traversing the substance of the meniscus and contacting an articular surface, and/or changes in meniscal morphology. Meniscal tears are classified by their orientation with regard to the substance of the meniscus.
Horizontal tears, which are commonly degenerative, extend in an oblique but predominantly horizontal course across the substance of the meniscus (Fig. 16-9). Vertical tears may be longitudinally or radially oriented. Longitudinal vertical tears commonly occur in the setting of acute trauma, such as pivot shirt injury, and are often amenable to repair, provided that they lie within the vascular periphery of the meniscus. Radial tears, if complete, disrupt the longitudinal fibers which provide hoop strength to the menisci, allowing for meniscal extrusion. A common location for radial tears is at the posterior horn root attachment junction; while these may be easily appreciated on coronal images, on sagittal images,
they may be subtle, appearing as “ghosting” on a single sagittal image, and sometimes not appearing on sagittal images at all, due to their orientation (Fig. 16-9). They may be evident on axial images, but again, this will largely depend on whether an axial image traverses the substance of the menisci.23,24
they may be subtle, appearing as “ghosting” on a single sagittal image, and sometimes not appearing on sagittal images at all, due to their orientation (Fig. 16-9). They may be evident on axial images, but again, this will largely depend on whether an axial image traverses the substance of the menisci.23,24
Bucket handle tears are longitudinally oriented vertical tears which allow the torn portion of the meniscus to displace, typically centrally, resulting in a “bucket handle” fragment of meniscal tissue which commonly results in mechanical symptoms. A “double PCL” sign is common on sagittal images, with the displaced bucket handle fragment paralleling the posterior cruciate ligament (PCL) (Fig. 16-10). The location of the vertical cleavage plane relative to the vascular periphery of the meniscus is important in terms of the potential for repair, as is the health of the nondisplaced meniscal remnant and bucket handle fragment, in terms of degeneration and additional areas of tearing.23,24
Flap tears are often obliquely oriented and may be subtle, with the flap largely visible only in a single plane (Fig. 16-11). Areas of abnormal meniscal truncation may provide a clue that there is a tear, allowing the radiologist to carefully scrutinize the images for a displaced flap.23,24
Meniscocapsular separation may occur in the setting of acute trauma, such as pivot shift injury. The posterior medial meniscocapsular junction and lateral fascicular attachments are well visualized on sagittal images. Laterally, injury manifests as fascicular hyperintensity and thickening and/or disruption. Medially, injury manifests as signal hyperintensity, with a complete separation appearing as a fluid signal cleft along the meniscocapsular junction.23
Discoid menisci are anatomic variants, more common laterally, resulting in meniscal tissue extending across the central and inner aspects of the compartment rather than being confined to the periphery (Fig. 16-12). This extra tissue is typically clearly visible on sagittal and coronal images, but is often markedly degenerated, to the point that it may not be easily recognizable as meniscal tissue.23
LIGAMENTS
Anterior Cruciate Ligament
The anterior cruciate ligament (ACL) is composed of parallel collagen fibers which are normally uniformly hypointense on clinical imaging sequences; these are typically interspersed with bands of fat, resulting in a striated appearance on non-fat-suppressed images (Fig. 16-13). The ACL has two distinct fiber bundles; the anteromedial bundle is slightly larger and is more taut in flexion, while the posterolateral bundle is more taut in extension.25
While complete ACL disruption is often evident clinically, MRI can provide valuable information in the setting of equivocal injury as well as in the assessment for concomitant injuries which may also warrant surgical intervention. In the acute setting, ACL tear appears as disruption of the normal ligament fibers, which typically appear hyperintense and thickened due to the interstitial load experienced prior to rupture (Fig. 16-14). Rupture is commonly the result of pivot shift injury, which typically also results in transchondral impaction injuries over the anterior lateral femoral condyle and posterior lateral plateau. These will appear as focal areas of subchondral marrow edema on fluid-sensitive, fat-suppressed images, commonly with focal subchondral depression and overlying chondral abnormality.26
Partial ACL tears are often more difficult to diagnose on MRI than complete tears, as the injury tends to have a more subtle appearance. Partial tears generally manifest as hyperintensity and thickening of fibers, which may be accompanied by changes in ligament morphology such as attenuation and a somewhat wavy or concave appearance. The characteristic pivot shift contusions commonly seen in the setting of complete ACL rupture tend to be absent.27
In the chronic setting, persistent complete ligament disruption often results in horizontal lie of the torn ligament, which generally appears hypointense following scar remodeling (Fig. 16-14).25 Torn ligament fibers may also be resorbed, resulting in absence of the ligament. Chronic ACL deficiency may allow anterior translation of the tibia, particularly within the lateral femorotibial compartment, with associated displacement/degeneration of the lateral meniscal posterior horn and preferential
wear of the subjacent articular cartilage.28 Deficiency of the ACL may also allow recurrent pivot shift; therefore, the presence of pivot shift contusions does not necessarily indicate an acute ACL disruption.
wear of the subjacent articular cartilage.28 Deficiency of the ACL may also allow recurrent pivot shift; therefore, the presence of pivot shift contusions does not necessarily indicate an acute ACL disruption.
A variety of associated injuries have been observed in conjunction with ACL tears sustained in the setting of pivot shift injury. In addition to the aforementioned lateral compartment transchondral impaction injuries, an additional area of impaction is often observed over the posterior aspect of the medial tibial plateau, thought to be related to contrecoup injury. Meniscal injury is not uncommon and often involved the meniscal periphery and/or meniscocapsular junction. Injury of the medial collateral ligament (MCL) and posterolateral corner (PCL) may also be observed and may warrant surgical intervention if severe.29
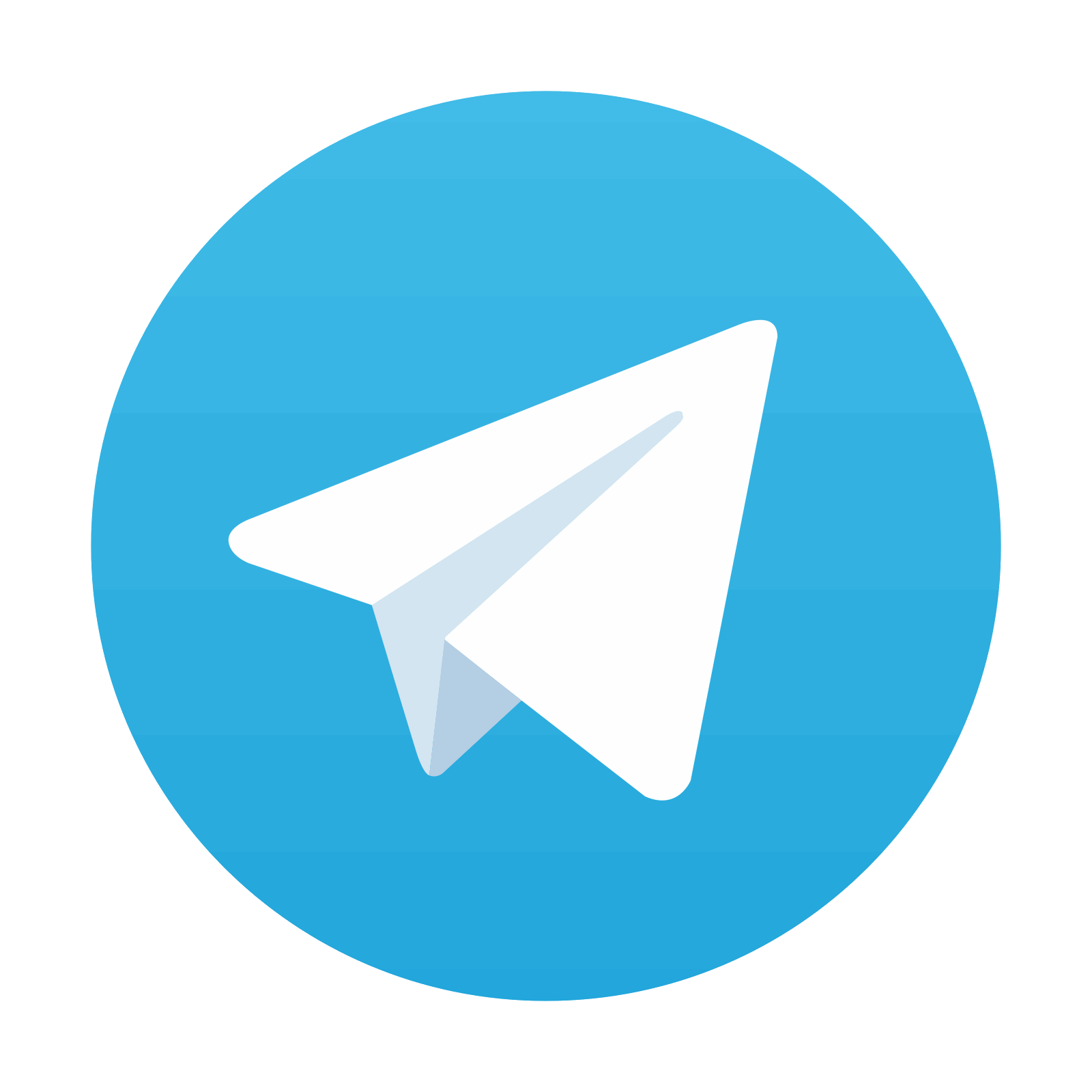
Stay updated, free articles. Join our Telegram channel

Full access? Get Clinical Tree
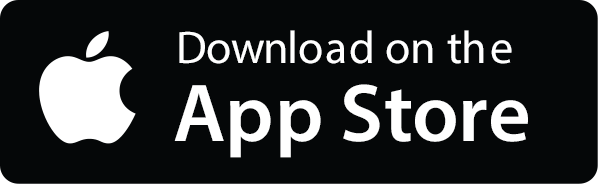
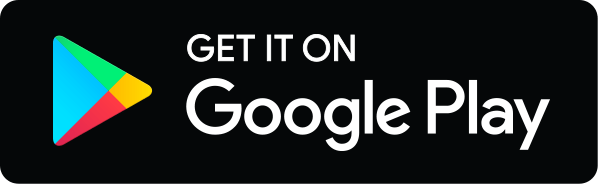