Introduction
In the patient with a failed total knee replacement (TKA), imaging modalities represent an integral part of diagnostic evaluation. As joint replacement arthroplasty becomes more common with the aging population and implant longevity improves, the number of patients requiring imaging evaluation for their TKA will continue to increase. This chapter discusses diagnostic imaging of the failed TKA, including the roles of radiographs, radionuclide scans, computed tomography (CT), and magnetic resonance imaging (MRI).
Radiographic Assessment
Standard radiographs used in the initial evaluation include weight-bearing anteroposterior (AP), lateral, tangential axial (Merchant), and long leg alignment views of the knee ( Fig. 4.1 ). The radiographs are evaluated for component alignment, size, and any overhang, stress fracture, component loosening, periprosthetic lucencies, osteolysis, wear of the polyethylene insert with loss of joint space or asymmetric joint space, and heterotopic ossification. The femoral component should be in 4 to 7 degrees of anatomic valgus, and the anterior flange should be in contact with the anterior cortex without notching. Any overhang should be noted. Femoral component overhang of greater than 3 mm doubles the risk of clinically important knee pain 2 years after TKA. The tibial component should be perpendicular to the long axis of the tibia on the AP radiograph and perpendicular or posteriorly sloped, appropriate for the implant design, on the lateral radiograph. The likelihood of tibial tray loosening is increased when it is placed in greater than 5 degrees of varus. The tibial tray should cover at least 85% of the tibial surface without overhang. Medial overhang of the tibial component can be a source of pain due to impingement on the pes anserinus or medial collateral ligament.

Preoperative and postoperative films are compared for size of the femoral component relative to the original anatomy, posterior condylar offset, patella height, and position of the joint line in relation to the patella. It is important to distinguish true patella baja, caused by a short patellar tendon, from iatrogenic patella baja, which results from a raised joint line, because the treatments of these conditions differ.
The value of oblique radiographs in accurately delineating the peripheral margins of osteolytic lesions is uncertain. Previous studies have suggested that plain radiographs, despite multiangle and multiprojection approaches, often underestimate the size of the osteolytic lesion. However, a study by Miura and colleagues demonstrated that radiographic analysis using the oblique posterior condylar view is reproducible and is significantly more accurate than standard radiographs for the detection of radiolucencies or osteolysis of the posterior aspects of the femoral condyles.
Patellofemoral Evaluation
On the Merchant view, the patella should be centered in the trochlear groove. Radiographic evidence of maltracking, including lateral tilt and subluxation, on an axial weight-bearing radiograph has been shown to more closely correlate with clinical symptoms than a standard unloaded Merchant view does. Risk factors for overstuffing of the patellofemoral compartment are noted, including anterior translation of the femoral component, oversizing of the femur, and creation of an aggregate patellar thickness thicker than on the contralateral side.
Loosening
Serial radiographs are an essential component in the evaluation of the painful TKA ( Fig. 4.2 ). Radiographic signs of loosening include progressive increase in radiolucent lines, changes in component position, subsidence, fracture of the cement mantle, and reaction about the tip of stemmed components. The cement–bone or component–bone interface is closely evaluated; loosening is suspected with all radiolucencies greater than 2 mm. Ritter and co-workers found that proper preparation of the cancellous bone and pressurization of the cement can reduce the initial occurrence of a radiolucent line. A loose tibial component frequently shifts into varus alignment, whereas a loose femoral component typically shifts into flexion. Visualization of osteolysis located in the posterior condyles may be obscured by the femoral component.

Fluoroscopically positioned radiographs can assist in ensuring an optimal view of the interface, particularly in uncemented prostheses ( Fig. 4.3 ). Although arthrography is rarely necessary, loosening is suggested by any flow of iodinated contrast material into the area of lucency. However, the absence of contrast flow does not exclude the diagnosis. For unicompartmental knee arthroplasty, lucency at the tip of the femoral peg is most associated with loosening of the femoral component.

Ligamentous Instability
Stress radiographs can be helpful in diagnosing subtle varus and valgus instability ( Fig. 4.4 ). Posterior cruciate ligament (PCL) instability can be detected by obtaining weight-bearing lateral radiographs in both flexion and extension. PCL instability is present when the femorotibial contact position is substantially more anterior in deep flexion than in full extension.

Wear
Polyethylene wear can result in joint asymmetry on an AP radiograph and is frequently associated with osteolysis. It is suggested by interval decrease in the space between the femoral condyles and the tibial baseplate on serial AP and lateral radiographs. One study that used ultrasound for the assessment of polyethylene wear reported the ability to determine wear with an accuracy of 0.5 mm.
Computed Tomography
Historically, CT was considered to be of limited utility because of the beam-hardening artifact that is produced when the metal severely attenuates the x-ray beam. Recent advances in the form of multidetector CT using high photon techniques helps overcome this problem. In addition, images can be reformatted, producing sagittal and coronal views.
Using modern techniques, CT scans are valuable in determining the size and extent of osteolytic lesions, proximity of the lytic areas to the prosthesis, and involvement of the cortical bone ( Fig. 4.5 ). Reish and co-workers showed that plain radiographs are inadequate for evaluating periprosthetic osteolysis in TKA. Only 8 (17%) of 48 lesions detected by multidetector CT were visible on the standard radiographs. In addition, CT slices using a specific protocol allow an exact analysis of the axial alignment in patients with suspected malrotation of the femoral or tibial component or both ( Fig. 4.6 ). A line drawn along the posterior aspects of the femoral condyle should be parallel to the epicondylar axis. Even 4 degrees of internal rotation of the femoral component can lead to patellar maltracking. Greater degrees of internal rotation can lead to patellar subluxation, patellar dislocation, and patellar prosthetic failure. In addition, it has been shown that increased internal rotation of the femoral component is associated with increased lateral flexion laxity and less favorable clinical outcomes. Tibial component rotation is assessed in relation to the medial third of the tibial tubercle. In a study of patients with stiffness complicating TKA, Bédard and colleagues found that 33 of 34 patients had internal rotation of the tibial component, with a mean rotation of 13.7 degrees (range, 1 degree external rotation to 35 degrees internal rotation). Twenty-four of the patients also had modest internal rotation of the femoral component, with a mean of 3.1 degrees. The authors recommended routine CT scanning of patients with stiffness complicating TKA to identify internally rotated components.


Magnetic Resonance Imaging
As with CT, the role of MRI has traditionally been limited due to metal artifact but has increased with the availability of new MRI software and imaging techniques ( Fig. 4.7 ). Examples of strategies to minimize artifacts include orienting the frequency-encoding gradient along the long axis of the prosthesis, using fast spin-echo sequences, using three-dimensional acquisitions and thin sections, using high matrix size, increasing receiver bandwidth, reducing interecho spacing, and using short tau inversion recovery (STIR) fat-suppression sequences.

These improvements have allowed for the evaluation of both intracapsular and extracapsular components of the TKA. Sofka and associates showed that MRI scans with metal subtraction software had diagnostic value in 20 (43.5%) of 46 cases of problematic TKA with negative plain radiographs, ultimately leading to further surgical or other therapeutic interventional procedures in these cases. The diagnoses included osteolysis, synovitis, bursitis, ligamentous or tendinous injury, fat-pad scarring, pigmented villonodular synovitis, and intramuscular hematoma. In addition, MRI is commonly used as a second- or third-line modality when radiographs are negative and the cause for a painful knee is uncertain.
Nuclear Medicine Scans
Bone Scan
A positive technetium-labeled bone scan is extremely sensitive for detecting bone remodeling changes around prosthetic joints and can indicate loosening, infection, or stress fracture. Diffuse uptake may be present in complex regional pain syndrome (CRPS). Several studies have reported that bone scans were neither sensitive nor specific for differentiating infection from aseptic loosening. Three-phase bone scans do little to improve the accuracy of diagnosing infection. Levitsky and co-workers reported a sensitivity of 30%, a specificity of 86%, and an accuracy of 68%, whereas Love and colleagues reported a sensitivity of 76%, a specificity of 51%, and an accuracy of 62% for diagnosing infection ( Table 4.1 ). Increased uptake on the three-phase technetium bone scan can persist indefinitely after TKA, which limits the usefulness of this test. In one study, increased periprosthetic uptake persisted for more than 1 year after surgery in 89% of tibial components and 63% of femoral components.
Type of Arthroplasty | Sensitivity (%) | Specificity (%) | Accuracy (%) | |
---|---|---|---|---|
Bone Scan | ||||
Weiss et al, 1979 | Hips | 100 | 77 | |
Love et al, 2008 | Hips/Knees | 50 | ||
Three-Phase Bone Scan | ||||
Levitsky et al, 1991 | Hips/Knees | 30 | 86 | 68 |
Palestro et al, 1991 | Knees | 67 | 70 | |
Love et al, 2008 | Hips/Knees | 76 | 51 | 62 |
Magnuson et al, 1988 | Hips/Knees | 100 | 18 | 53 |
McKillop et al, 1984 | Hips/Knees | 80 | ||
Mountford et al, 1986 | Hips | 80 | ||
Aliabadi et al, 1989 | Hips | 37 | 100 | |
Bone/Gallium Scan | ||||
Merkel et al, 1986 | Hips/Knees | 66 | 81 | 77 |
Gomez-Luzuriaga et al, 1988 | Hips | 70 | 90 | 80 |
Kraemer et al, 1993 | ||||
Love et al, 2008 | Hips/Knees | 79 | 59 | 66 |
Labeled Leukocyte Imaging | ||||
Pring et al, 1986 | Hips/Knees | 100 | 89 | |
Magnuson et al, 1988 | Hips/Knees | 88 | 73 | |
McKillop et al, 1984 | Hips/Knees | 50 | 100 | |
Wukich et al, 1987 | Hips/Knees | 100 | 45 | |
Palestro et al, 1991 | Knees | 67 | 78 | |
Johnson et al, 1988 | Hips | 100 | 50 | |
Leukocyte/Bone Imaging | ||||
Wukich et al, 1987 | Hips/Knees | 85 | 85 | |
Palestro et al, 1991 | Knees | 89 | 75 | |
Johnson et al, 1988 | Hips | 88 | 95 | |
Love et al, 2008 | Hips/Knees | 70 | ||
Leukocyte/Marrow Imaging | ||||
Mulamba et al, 1983 | Hips | 92 | 100 | |
Palestro et al, 1991 | Knees | 100 | 97 | |
Love et al, 2008 | Hips/Knees | 96 | 87 | 91 |
Joseph et al, 2001 | Hips/Knees | 100 | 46 | |
Love et al, 2004 | Hips/Knees | 100 | 91 | 95 |
FDG-PET | ||||
Zhuang et al, 2001 | Hips/Knees | 90 | 89 | 89 |
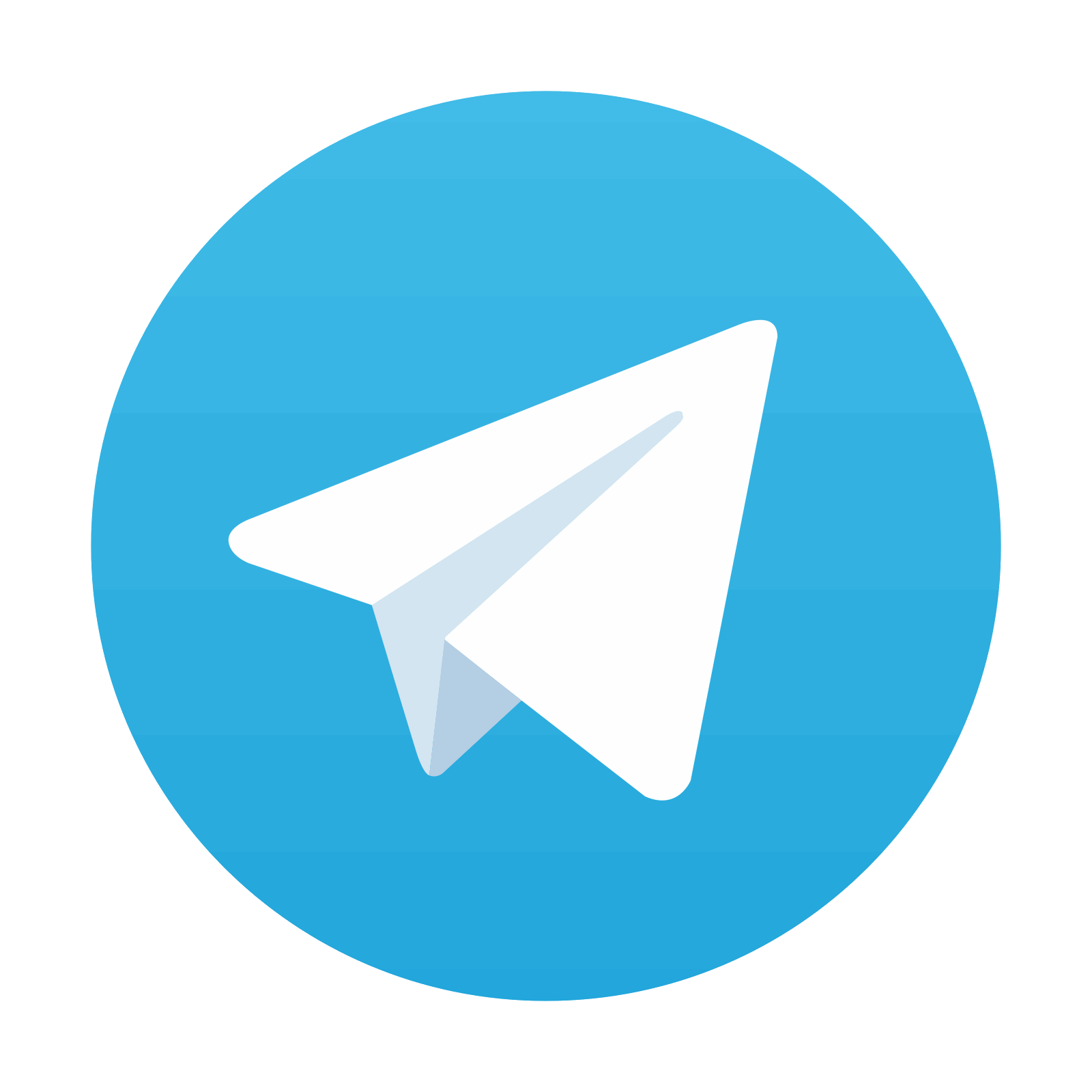
Stay updated, free articles. Join our Telegram channel

Full access? Get Clinical Tree
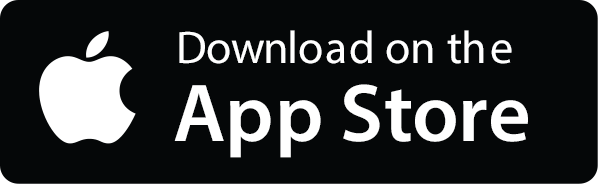
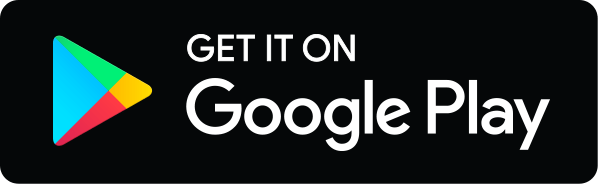
