Imaging in Sports Medicine
Jonathan A. Staser
Kenneth A. Buckwalter
After history and physical examination, imaging may be the most important diagnostic tool in the assessment of patients with sports injuries. Imaging techniques for these patients include radiography, fluoroscopy, nuclear medicine scintigraphy, ultrasound (US), computed tomography (CT), and magnetic resonance imaging (MRI). In some patients, it may be necessary to use more than one technique. For example, the imaging workup of a shoulder injury might begin with plain radiographs, but requires an advanced imaging technique such as a MR arthrogram to confirm the diagnosis.
This chapter will review some of the indications and general approaches to imaging of sports-related injuries. A discussion of muscle tears will be followed by a more detailed review covering imaging of the knee, shoulder, elbow, and ankle, the most commonly injured joints encountered in practice.
Radiography
Radiography employs x-rays to create an image of the patient. Tissues, like bone, that absorb more of the x-ray beam are whiter on the image; tissues like fat absorb much less of the x-ray beam and are blacker on the image. Because radiography is projectional, a radiograph is a two-dimensional representation of a three-dimensional object. Overlap of structures can lead to confusion and, for this reason, a minimum of two orthogonal (perpendicular) projections are recommended for assessment of bony structures. For small joints in the extremities such as the hand and wrist, three views are recommended to reveal small fractures that may only be seen on a single view (1). The minor incremental cost and radiation exposure are justifiable in this context.
Radiography is unparalleled in its ability to demonstrate bony detail because it has the highest spatial resolution of any imaging technique. However, radiography has limited ability to visualize differences in soft tissue structures. Consequently, the strength of radiography lies in the detection of bony pathology such as fractures, not soft tissue injuries. Radiographs are also extremely useful in detecting soft tissue calcifications. For example, hydroxyapatite crystals seen in calcific bursitis are easy to identify on radiographs, but difficult to detect on MRI.
Although radiography traditionally has been performed using special x-ray film and film cassettes, advances in technology have facilitated the production of radiographs in digital format (2). The two main methods used are computed radiography (CR) and digital radiography (DR). CR uses special plates that are scanned by a laser after exposure to the x-ray beam, producing the radiographic image. DR captures the digital image directly. The ability to acquire, store, and transmit radiographic images allows for simultaneous multiple location display. The diagnostic quality of digital images is equivalent to conventional film-screen radiography and there are additional benefits such as reduced radiation exposure and efficiency that warrant installation of digital equipment in busy facilities.
It is important to recognize that radiography has limitations. In the case of suspected fracture, if initial radiographs are found to be normal, there are several options. The least expensive option is to obtain follow-up radiographs. Because bony remodeling is dependent on the patient’s metabolic activity, radiographic changes will be visible sooner in children and adolescents (5–7 days)
than adults (7–14 days); radiographic changes may be extremely difficult to detect in seniors, particularly those with osteoporosis.
than adults (7–14 days); radiographic changes may be extremely difficult to detect in seniors, particularly those with osteoporosis.
For the high performance athlete, delayed radiographic imaging may be unacceptable because of the time constraints imposed by training or game play. In this situation, CT or MRI is performed next. If the working diagnosis is fracture, CT is recommended; if the working diagnosis is soft tissue injury, MRI is recommended. Because MRI is more sensitive in the detection of bone marrow edema and soft tissue damage, it is often obtained. MRI may not be able to distinguish a nondisplaced fracture from a large bone contusion. Additionally, small avulsion fractures often are occult on MRI. For these reasons, it is essential to remember that radiographs should always be obtained concurrently.
Nuclear Medicine
Bone scintigraphy is performed by injecting a radiopharmaceutical (typically Tc-methylene diphosphonate) intravenously followed by imaging in 1 to 2 hours. The radiopharmaceutical concentrates in metabolically active regions of the bone. A viable blood supply is necessary to deliver the agent to the target tissues. Because the radiopharmaceutical is radioactive, only a small quantity must localize in the bone for a positive result. Therefore, nuclear imaging is extremely sensitive in detecting bony remodeling. However, the radiopharmaceuticals used in skeletal imaging are nonspecific; a fracture could present a similar appearance as an infection or tumor.
Nuclear bone scans have other limitations. Although scintigraphy is extremely useful when a large region of the body needs to be surveyed for radiographically occult disease, detailed pictures of the anatomy are difficult to obtain. Because growing children have metabolically active growth plates, injuries to the growth plates may not be detectable on scintigraphy. Furthermore, imaging of the soft tissues such as ligaments, tendons, and cartilage is not possible with a bone scan, further limiting the usefulness of scintigraphy. In large part, scintigraphy has been supplanted by MRI, which is usually as sensitive as scintigraphy in assessing bone marrow disease, provides superior spatial resolution and facilitates visualization of soft tissue injuries.
Positron emission tomography (PET) is another nuclear imaging technique for skeletal and soft tissue imaging. Most of the current applications of PET are cardiac or oncologic, but the clinical experience continues to evolve. Newer PET devices are coupled with CT scanners: PET/CT. The addition of CT imaging to PET addresses the concerns that some physicians have regarding the limited spatial resolution of nuclear imaging. The short-lived radioisotopes for PET reduce the time between injection and imaging, increasing the usefulness of the technique. Although there is great potential for this imaging method, the role in sports imaging is unclear and the examinations are costly.
Ultrasound
US relies on the transmission and reception of high-frequency sound waves (4–15 mHz) to produce images of soft tissues. In most instances, the US transducer is placed on the skin overlying the symptomatic region. The depth of penetration of the beam is determined by the frequency of the transducer; lower frequencies penetrate deeper into the tissues. Spatial resolution is also determined by the frequency of the transducer; higher frequencies are associated with higher resolution. As a result, it is difficult to obtain detailed pictures of deep tissues, but superficial structures can be evaluated in great detail.
US images are limited to a small display field. Consequently, US images are difficult to orient and interpret. Some surgeons are reluctant to operate on the basis of US findings alone, which is another limitation of US. Furthermore, it is difficult to show a large anatomical region such as a thigh on a single image. US examinations typically are performed in real time by a physician or a technologist supervised by a physician. There is a steep learning curve for musculoskeletal imaging, further limiting the usefulness of US in sports injuries.
On the other hand, US imaging is ideal for interventional procedures, such as steroid injections of bursae, tendon sheaths, and joints. The real time capability of US makes it possible to do these procedures with ease. Additionally, US equipment is relatively inexpensive and the technology has continued to shrink so that portable US devices are now laptop computer–sized. Image quality for these smaller devices can be inferior to that produced by larger stand alone units, but the gap in quality between small and larger units continues to narrow. The role of US in diagnosis and intervention of musculoskeletal injuries is continuing to expand.
Computed Tomography
CT is a widely available imaging technique. The strength of CT lies in its ability to image bony structures in high resolution. Additionally, CT imaging is tomographic, revealing the internal structure of patients by displaying two-dimensional cross-sections of the scanned anatomy. The cross-sectional display is helpful in eliminating the overlap of structures that limits radiography. Although CT is the workhorse of modern body imaging, discrimination of the soft tissues in the musculoskeletal system limits its application in extremity imaging. In general, intravenous contrast is not warranted unless there is a suspicion for mass or abscess.
Throughout most of its history, CT imaging was limited to the transverse plane. Because radiographs of joints are usually obtained in frontal and lateral projections, physicians are most comfortable assessing joints using sagittal or coronal planes; unfortunately, conventional CT is best suited for axial plane imaging. The development of multichannel computed tomography (MCCT) scanners
over the last decade has enabled submillimeter-thick imaging, which makes it possible to create sagittal and coronal multiplanar reformations of joints in detail similar to direct axial imaging (3).
over the last decade has enabled submillimeter-thick imaging, which makes it possible to create sagittal and coronal multiplanar reformations of joints in detail similar to direct axial imaging (3).
Both CT and MCCT use x-rays to produce images of patients. Radiation dose is relatively low for most CT examinations, but it is not insignificant, especially when multiple follow-up examinations are performed. Radiation-sensitive tissues such as the gonads and thyroid are exposed when imaging the pelvis (hip joints) and the upper chest (shoulder and sternoclavicular joints), but most extremity imaging (elbow, wrist, knee, ankle, foot) can be performed without exposing radiation-sensitive tissues. In general, radiation exposure should be minimized in children and young adults, but should not be considered a contraindication. Pregnancy is a relative contraindication for a CT examination, particularly when direct exposure of the fetus would be incurred.
The availability of modern MCCT scanners has expanded the indications for CT imaging. In particular, imaging of patients with metal hardware is facilitated greatly by MCCT (4). Although metal artifacts may not be eliminated completely, most patients with orthopedic hardware can be imaged using MCCT. Types of hardware that do not interfere with bony visualization include intramedullary nails (rods), fixation plates, and small screws. Many patients with joint prostheses also can be imaged successfully. Additionally, patients with metal fragments such as shrapnel and gunshot debris are suitable candidates for CT examinations.
Another good application for MCCT is high resolution computed tomographic arthrography (CTA) (5). CTA can be performed in any joint using either single or double contrast technique. The indications for CTA include patients with the following conditions: metal in close proximity to a joint, MR incompatible implants, severe claustrophobia, failed MR examination (with or without an arthrogram), and morbid obesity. CTA provides excellent visualization of the articular surfaces and intra-articular structures such as the knee menisci (see Figure 34.1) and the glenoid labrum in the shoulder.
Magnetic Resonance Imaging
MRI has revolutionized imaging of the musculoskeletal system. Similar to CT, MRI produces cross-sectional images of the relevant anatomy. Unlike CT, MRI uses no ionizing radiation. Instead, MRI uses radiowaves to produce images of the body. For most applications, the soft tissue contrast of MRI is much greater than that of CT, explaining the usefulness of this technique for musculoskeletal imaging. Additionally, depending on the radiofrequency pulse sequence, images can be made to emphasize different types of tissue contrast.
For conventional MRI, the hydrogen atom or proton is the most widely imaged nucleus. Tissue contrast is related to the T1 and T2 relaxation times of the target tissues as well as the concentration of protons in the tissue. Depending on the pulse sequence used, tissue signal intensities can
reflect T1, T2, or proton density weighting. Fluid is dark and fat is bright on T1- weighted images; fluid is brighter than fat on T2-weighted images; proton density weighted images are somewhere in between (see Table 34.1). In general, injuries are detected on MR images by alterations in anatomy, for example, a meniscal tear, or by the presence of edema, for example, a bone contusion. As a result, it is common practice to obtain sequences that show anatomical detail in conjunction with fluid-sensitive sequences in every imaging plane.
reflect T1, T2, or proton density weighting. Fluid is dark and fat is bright on T1- weighted images; fluid is brighter than fat on T2-weighted images; proton density weighted images are somewhere in between (see Table 34.1). In general, injuries are detected on MR images by alterations in anatomy, for example, a meniscal tear, or by the presence of edema, for example, a bone contusion. As a result, it is common practice to obtain sequences that show anatomical detail in conjunction with fluid-sensitive sequences in every imaging plane.
TABLE 34.1 Relative Tissue Signal and Mr Image Weighting | ||||||||
---|---|---|---|---|---|---|---|---|
|
Chemical saturation (fat saturation) is a commonly used technique to reduce signal from fat. T2-weighted images produced with fat suppression increase the contrast between edema and bone marrow, making it easier to identify bone contusions in the intramedullary space. A similar effect can be achieved using the short inversion time inversion recovery (STIR) sequence (see Figure 34.2). Fat suppression applied to T1-weighted images increases image contrast on postgadolinium enhanced images.
Although MRI is very safe, the intense magnetic field used to produce images may not be safe for all patients. This magnetic field is tens of thousands of times stronger than the earth’s magnetic field and the main MR magnet is always turned on. Therefore, exposure to the magnet may be harmful even if the patient is not being imaged at the time of exposure. Most orthopedic hardware is considered to be safe; however, metal implants in close proximity to the region of interest can obscure detail. Patients with pacemakers, specific kinds of heart valves, and certain venous filters may not be safe in close proximity to the magnet. Because some medical devices are MR safe, it is important to know as much as possible about the device before the examination including such details as device manufacturer and model number.
Intravenous contrast agents such as gadolinium can be helpful to document sites of active inflammation, for example, plantar fasciitis, and should be administered if a malignant soft tissue mass is suspected. For many examinations, contrast is not necessary. However, there are growing number of applications when intra-articular contrast is extremely useful. For a direct MRI arthrogram, the gadolinium agent is diluted and injected into the joint under fluoroscopy. If radiographic contrast media (iodine) is injected along with the gadolinium, CTA can be performed without another injection if the patient is unable to tolerate the MRI examination. It is also possible to perform an indirect MRI arthrogram by injecting the gadolinium contrast intravenously and performing delayed imaging within 20 to 60 minutes. This procedure eliminates the need for an arthrogram and is well tolerated by most patients. Indirect arthrography works well in joints like the knee that have a small to moderate sized effusion; however, indirect arthrography is not as useful in joints like the shoulder where distension of the joint is necessary for optimal visualization of labral tissue.
Presently, most imaging is performed at 1.5 Tesla (T) and there is a great deal of experience in this field strength. Clinical imaging is now possible at higher and lower field strengths. Imaging at 3.0 T is driven by neuroimaging and body applications; however, scanners currently are being delivered with musculoskeletal coils to facilitate musculoskeletal applications. Although there is potential for improved image quality at 3.0 T, care must be taken to address image artifacts such as chemical shift, which will offset the gains achieved by higher field strength imaging. Additionally, tissue heating limits the use of certain pulse sequences and image contrast can be different than that at 1.5 T. The clinical utility of 3.0 T imaging may take time to establish for routine bone and joint studies; except for specialized examinations, there is minimal incremental benefit at present.
Patient preferences for less confining magnets have led to the development of the open magnet scanner. A wide variety of field strengths are available from 0.2T to 1.0T. Advances in open imaging technology have improved image quality greatly; however, many systems may be
operating without the benefit of a recent system upgrade. In general, higher field strengths are associated with higher image quality, and this is also true for open magnets (see Figure 34.3). When referring patients to any system, particularly an open system, it is worthwhile inquiring if the system has passed the American College of Radiology Accreditation standard; this is a standard required by some insurers and is a minimal quality guarantee (6).
operating without the benefit of a recent system upgrade. In general, higher field strengths are associated with higher image quality, and this is also true for open magnets (see Figure 34.3). When referring patients to any system, particularly an open system, it is worthwhile inquiring if the system has passed the American College of Radiology Accreditation standard; this is a standard required by some insurers and is a minimal quality guarantee (6).
Muscle and Tendon Tears
Muscle and tendon tears are extremely common sports injuries. Optimal treatment depends on accurate staging. The bone–tendon–muscle unit tears at its weakest point and this functional unit is analogous to a chain, which is only as strong as its weakest link. The weakest link varies with age; adolescents tend to avulse apophyses, adults tear the muscle, and mature adults often tear tendons. Knowledge of these injury patterns is helpful to guide the imaging assessment.
After the initial clinical evaluation, radiographs should be obtained. This is especially true for older children and adolescents presenting with an apophyseal injury, which may be diagnosed from the initial radiographs. Common sites of apophyseal injuries are the ischial tuberosity (hamstrings), the anterior inferior iliac spine (rectus femoris) and the anterior superior iliac spine (sartorius) (7,8). Further investigation of muscle and tendon injuries proceeds with US or MRI. US has a limited field of view and is best suited for focal abnormalities such as a distal Achilles tendon tear. Because many muscle tears may extend over tens of centimeters, MRI is suggested as the most effective imaging tool (9). MRI is a more comprehensive assessment than US and allows for the assessment of occult bone injuries such as stress fractures.
Muscle tears occur more often in muscles spanning two joints, muscles with a higher proportion of type II fibers, and with activities favoring eccentric muscle contraction (10). As a result, more muscle tears are seen in lower extremity muscles, such as the hamstring and quadriceps muscle groups in the thigh and the gastrocnemius muscle in the calf. Upper extremity tears are seen less often; most of them occur in the biceps muscle or tendon of the upper arm (see Figure 34.4). Other factors increasing the likelihood of tear include aging, prior injury, and steroid abuse.
At MR imaging, muscle tears are found at the myotendinous junction within the muscle body; this is the junction between muscle fascicles and the central tendon, not the anatomical junction between the muscle and tendon seen grossly at surgery. On cross-sectional views of muscle tears, the edema is seen around the central tendon of the muscle.
Muscle tears can be graded depending on the severity of the injury. A grade I or mild strain is characterized by low level diffuse muscle edema demonstrated on fat suppressed T2- weighted or STIR images. Perifascial edema is sometimes seen with a grade I strain. Conservative treatment is usually sufficient. A grade II or moderate strain is
characterized by a partial thickness tear associated with partial loss of strength. At MRI, a grade II strain presents as high signal at the myotendinous junction with perifascial edema. Most of these strains resolve in 10 to 12 days, but they are associated with an increased risk of future tear. Lastly, grade III strains are complete muscle tears with discontinuous muscle fibers; a hematoma may be visible in the gap (see Figure 34.5). Surgery may be indicated in the case of a grade III strain to prevent permanent retraction and muscle atrophy.
characterized by a partial thickness tear associated with partial loss of strength. At MRI, a grade II strain presents as high signal at the myotendinous junction with perifascial edema. Most of these strains resolve in 10 to 12 days, but they are associated with an increased risk of future tear. Lastly, grade III strains are complete muscle tears with discontinuous muscle fibers; a hematoma may be visible in the gap (see Figure 34.5). Surgery may be indicated in the case of a grade III strain to prevent permanent retraction and muscle atrophy.
Older patients may present with tendon tears instead of a muscle injury. Other factors predisposing patients to tendon tears include metabolic diseases such as hyperparathyroidism and diabetes (see Figure 34.6). Lastly, patients on fluoroquinolone antibiotics can experience tendon disruption, particularly the Achilles tendon; comorbidities include obesity, renal disease and prior steroid injection (11).
A variety of conditions can mimic muscle tears. Delayed onset muscle soreness (DOMS) resembles a grade I muscle strain at MRI, but symptoms begin 1 to 2 days after exercise as opposed to the immediate symptoms of a grade I strain. Patients with poorly controlled type 1 diabetes can develop muscle infarcts that present clinically with calf or thigh pain, resembling a muscle strain. The edema pattern of infarcts is more diffuse than the peritendinous edema of a muscle strain, allowing differentiation. Patients with muscle abscess will present with muscle pain, but the clinical history and MR appearance should lead to the correct diagnosis. Rupture of a Baker’s cyst can cause acute calf pain, resembling a muscle strain or deep venous thrombosis, but the edema of a ruptured cyst often extends to the intramuscular fascial planes, facilitating the correct diagnosis.
Knee Imaging
The knee is often injured in sport activities. Physical examination of the knee may be incomplete in the setting of an acute injury because of pain or guarding, increasing the importance of imaging. Radiographs are helpful to assess for fracture, effusion, and calcified loose bodies. In acute trauma, a cross-table lateral radiograph of the knee is preferred to a conventional lateral knee radiograph to look for the presence of a fat-fluid level which indicates an intra-articular fracture. Radiographs also are helpful to assess for small cortical fractures that may be difficult to detect on MRI. One example, the Segond fracture (see Figure 34.7), is a harmless looking chip fracture of the proximal lateral tibial plateau margin. This fracture represents an avulsion of the middle third lateral capsular ligament and has a high likelihood of an associated anterior cruciate ligament tear (ACL). Similar, but less common, the reverse Segond, is a medial tibial plateau fracture associated with a posterior cruciate ligament (PCL) tear and a medial meniscus tear.
If radiographs are normal or inconclusive and a serious injury remains a strong consideration, MRI or CT can be pursued as the next step. CT is helpful when assessing bony structures and CT is used most often to confirm or stage a fracture if surgical repair is planned. For most sport related trauma, MRI is the most commonly used imaging tool.
Although MRI excels at soft tissue imaging, the bone marrow also can be assessed well with MRI. Bone marrow edema can be detected at MRI using fat suppressed T2-weighted or STIR images. Edema may indicate the presence of a contusion, stress fracture, infarct, or other pathological process. Following trauma, edema in the marrow space usually indicates a bone contusion which represents microscopic trabecular disruption and intraosseous hemorrhage. In general, avulsion injuries such as the Segond fracture are not associated with significant marrow edema; however, impaction injuries are associated with significant edema. Additionally, marrow signal changes, particularly those in the subchondral region, are nonspecific; some of these abnormalities represent stress-related changes or early degenerative cysts. A typical stress fracture seen in the distal femur is important to recognize as it was previously referred to as spontaneous osteonecrosis of the knee (SONK). This condition actually represents a subcortical insufficiency fracture of the femoral condyle (12). The typical patient is not a marathon runner, but a middle-aged adult, perhaps inspired by a new exercise program. Intense marrow edema is identified throughout most of the affected femoral condyle, associated with significant pain (see Figure 34.8). Many patients have associated chronic meniscus tears.
Meniscus tears are quite common and easily detected with MRI; sensitivity and specificities vary between 85% and 95% for the medial meniscus with similar, but slightly lower numbers for the lateral meniscus (13,14,15,16). The normal meniscus consists of fibrocartilage, which is dark on most MRI pulse sequences (see Figure 34.3). Each meniscus is C-shaped and is divided roughly into an anterior horn, a body, and a posterior horn. On sagittal and coronal images, the meniscus has a triangular shape. The point of the triangle is referred to as the free edge or apex and the base of the triangle is the peripheral margin. The remaining two surfaces articulate with the femoral condyle and the tibial plateau. The anterior and posterior horns are best seen on sagittal images; the meniscal body is best seen on coronal images. Tears are diagnosed by the presence of a line of increased signal extending to one or both of the articular surfaces (see Figure 34.9) (17). To decrease the number of false positives, the finding must be seen on a minimum of two images (18). An additional sign of tear is distortion of the meniscal shape with blunting or irregularity of the normal black triangle (see Figure 34.8B). Some meniscus signal changes are associated with parameniscal cysts and the presence of a cyst increases the likelihood of a tear.
There are varieties of types of meniscus tears including horizontal, vertical, and complex. Radial tears involve the free edge of the meniscus and may extend into the substance of the meniscus resulting in flap or parrot beak tears. Most significant is the bucket handle tear which is a vertical tear involving the entire meniscus (19). The free edge fragment, the “bucket handle”, often displaces into the central notch of the knee, resulting in locking or other mechanical symptoms (see Figure 34.10). Less comprehensive tears may produce a free fragment which rotates or flips into another portion of the knee.
Occasionally, the meniscus is too large. This developmental abnormality usually occurs laterally and is termed a discoid meniscus; it can occur bilaterally. A discoid meniscus is more prone to tear than a normal meniscus, possibly related to the altered stresses induced by the abnormal meniscus shape. There are several subtypes, but on coronal images the mid-body of most discoid menisci extends beyond the medial or lateral compartment midline (see Figure 34.3). On sagittal images, the normal “bowtie” shape of the meniscus persists on three or more consecutive images.
Serious injuries of the knee may be associated with ligament tears. MRI is extremely reliable in the evaluation of ACL tears (20). There are numerous indirect signs of ACL injury (21). However, the most reliable sign of a complete ACL tear is nonvisualization of the ligament; a normal appearing ACL on MRI has near 100% accuracy in confirming that the ligament is intact (see Figure 34.11). Because valgus stress is associated with ACL injuries, it is common to see lateral compartment bone bruises in
the setting of an ACL tear secondary to impaction of the lateral femoral condyle on the lateral tibial plateau. More severe valgus stress results in additional tears of the medial collateral ligament (MCL) and the medial meniscus, although there is some evidence to suggest that lateral meniscus tears are more common than medial meniscus tears in this setting (22). PCL tears are much less common than ACL tears and usually are associated with significant trauma; isolated PCL tears are uncommon (23,24).
the setting of an ACL tear secondary to impaction of the lateral femoral condyle on the lateral tibial plateau. More severe valgus stress results in additional tears of the medial collateral ligament (MCL) and the medial meniscus, although there is some evidence to suggest that lateral meniscus tears are more common than medial meniscus tears in this setting (22). PCL tears are much less common than ACL tears and usually are associated with significant trauma; isolated PCL tears are uncommon (23,24).
Tears of the MCL and lateral collateral ligament (LCL) usually occur in association with other injuries (25,26
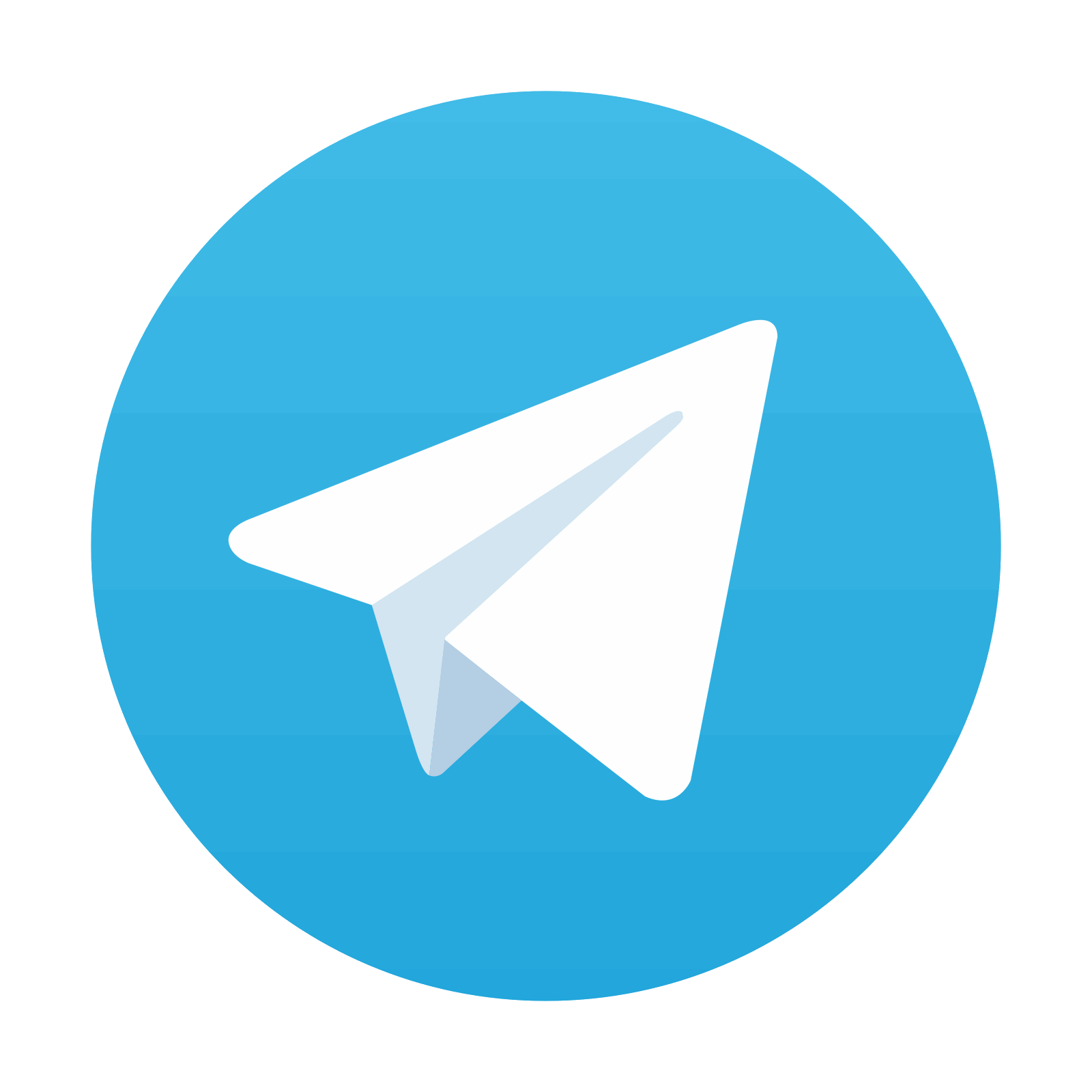
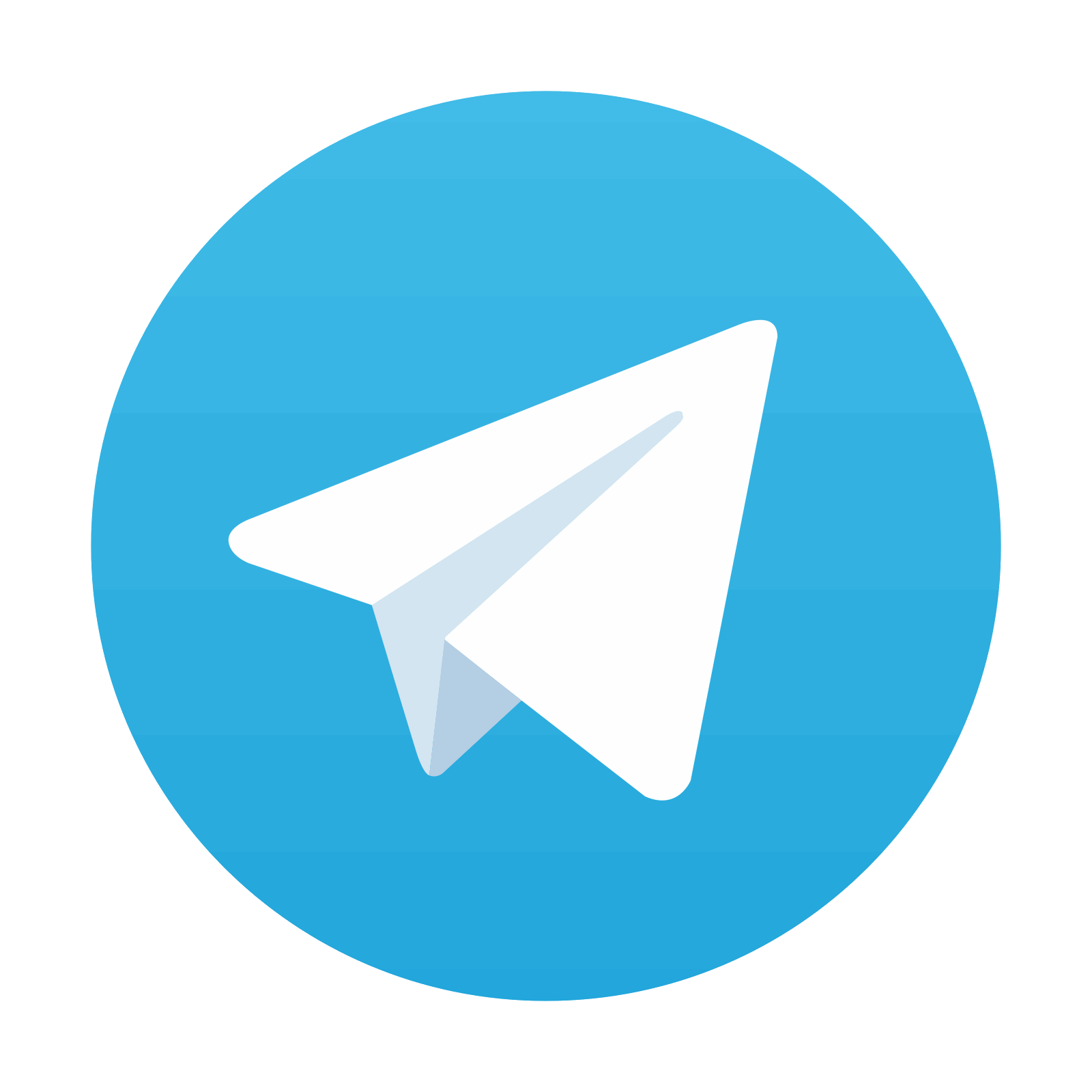
Stay updated, free articles. Join our Telegram channel

Full access? Get Clinical Tree
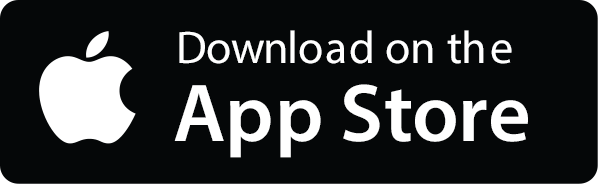
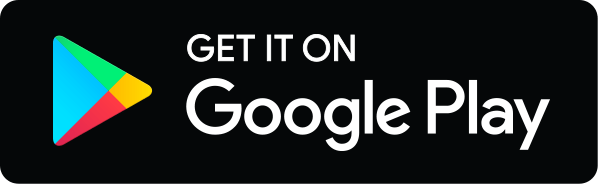
