Familial tumoral calcinosis (TC) is a rare disorder distinguished by the development of ectopic and vascular calcified masses that occur in settings of hyperphosphatemia (hFTC) and normophosphatemia (nFTC). Serum phosphorus concentrations are relatively tightly controlled by interconnected endocrine activity at the level of the intestine, kidney, and skeleton. Discovering the molecular causes for heritable forms of hFTC has shed new light on the regulation of serum phosphate balance. This review will focus upon the genetic basis and clinical approaches for hFTC, due to genes that are related to the phosphaturic hormone fibroblast growth factor-23 ( FGF23 ). These include FGF23 itself, an FGF23-glycosylating enzyme ( GALNT3 ), and the FGF23 co-receptor α-Klotho (α KL ). Our understanding of the molecular basis of hFTC will, in the short term, aid in understanding normal phosphate balance, and in the future, provide potential insight into the design of novel therapeutic strategies for both rare and common disorders of phosphate metabolism.
Introduction
Familial tumoral calcinosis (TC) is a rare autosomal recessive metabolic disorder characterised by the progressive deposition of calcium phosphate crystals in periarticular spaces and soft tissues. TC may manifest under situations of hyperphosphatemia (hyperphosphatemic familial TC; hFTC; MIM ID #211900) or normophosphatemia (normophophatemic familial TC; nFTC; MIM ID #610455). Maintenance of serum phosphate concentrations is required for proper skeletal development and for preservation of bone integrity. In addition, phosphate is required for cellular processes involving energy transfer in the form of adenosine triphosphate (ATP), is an integral molecule in DNA and RNA and is a critical component of multiple intracellular signalling pathways. Recent advances in our understanding of hFTC have shed light on the underlying mechanisms that control phosphate homeostasis in normal and in disordered states. The biochemical hallmark of TC is hyperphosphatemia caused by increased renal reabsorption of phosphate. This review summarises the heterogeneous genetic defects, and molecular mechanisms that lead to hFTC involving the phosphaturic hormone, fibroblast growth factor-23 (FGF23; nFTC will be reviewed elsewhere in this issue).
Phosphate homeostasis
Maintenance of serum phosphate concentrations involves hormonal regulation at the level of the intestine, skeleton and kidneys. The skeleton represents the largest stores of phosphate complexed with calcium in hydroxyapatite crystals, which constitute the main inorganic component of the mineralised bone matrix. Normal serum concentrations of phosphate in adults range from 2.5–4.5 mg dl −1 and are fairly tightly regulated. Serum phosphate concentrations are higher in infancy, decreasing as the child ages to adolescence and adulthood. Absorption of phosphate in the intestine is directly proportional to dietary intake, but is also influenced by the active form of vitamin D, 1,25(OH) 2 vitamin D (1,25D). In normal individuals, 25-hydroxy vitamin D is converted to 1,25D by the 25-hydroxy vitamin D 1-alpha hydroxylase enzyme (1α(OH)ase; Cyp27b1 ) and inactivated by the vitamin D 24-hydroxylase ( Cyp24 ) in the kidney proximal tubule (PT). 1,25D regulates serum phosphate concentrations by increasing calcium and phosphate absorption in the intestines, and at high concentrations, by increasing phosphate release by stimulating skeletal reabsorption . Rising serum calcium concentrations decrease parathyroid hormone PTH secretion, which in turn releases expressional inhibition of the primary transport protein in the PT, the type IIa sodium-dependent phosphate co-transporter, NPT2a . The abundance of apical NPT2a is the main determinant of percent tubular reabsorption of phosphate (%TRP) as this transporter accounts for 70–80% of reabsorption . Increased abundance of the NPT2a protein confers increased %TRP and consequently raises serum phosphate level and vice versa. Accordingly, in normal individuals the serum phosphate concentration also determines the expression of NPT2a: hypophosphatemia increases the expression of NPT2a, and hyperphosphatemia results in decreased expression of NPT2a, to re-establish normal serum phosphate. Although less is known about the type IIc sodium-phosphate co-transporter, NPT2c, this protein must also play a role in PT phosphate reabsorption, as inactivating NPT2c mutations lead to renal phosphate wasting and the autosomal recessive disorder hereditary hypophosphatemic rickets with hypercalciuria (HHRH) .
Phosphate homeostasis
Maintenance of serum phosphate concentrations involves hormonal regulation at the level of the intestine, skeleton and kidneys. The skeleton represents the largest stores of phosphate complexed with calcium in hydroxyapatite crystals, which constitute the main inorganic component of the mineralised bone matrix. Normal serum concentrations of phosphate in adults range from 2.5–4.5 mg dl −1 and are fairly tightly regulated. Serum phosphate concentrations are higher in infancy, decreasing as the child ages to adolescence and adulthood. Absorption of phosphate in the intestine is directly proportional to dietary intake, but is also influenced by the active form of vitamin D, 1,25(OH) 2 vitamin D (1,25D). In normal individuals, 25-hydroxy vitamin D is converted to 1,25D by the 25-hydroxy vitamin D 1-alpha hydroxylase enzyme (1α(OH)ase; Cyp27b1 ) and inactivated by the vitamin D 24-hydroxylase ( Cyp24 ) in the kidney proximal tubule (PT). 1,25D regulates serum phosphate concentrations by increasing calcium and phosphate absorption in the intestines, and at high concentrations, by increasing phosphate release by stimulating skeletal reabsorption . Rising serum calcium concentrations decrease parathyroid hormone PTH secretion, which in turn releases expressional inhibition of the primary transport protein in the PT, the type IIa sodium-dependent phosphate co-transporter, NPT2a . The abundance of apical NPT2a is the main determinant of percent tubular reabsorption of phosphate (%TRP) as this transporter accounts for 70–80% of reabsorption . Increased abundance of the NPT2a protein confers increased %TRP and consequently raises serum phosphate level and vice versa. Accordingly, in normal individuals the serum phosphate concentration also determines the expression of NPT2a: hypophosphatemia increases the expression of NPT2a, and hyperphosphatemia results in decreased expression of NPT2a, to re-establish normal serum phosphate. Although less is known about the type IIc sodium-phosphate co-transporter, NPT2c, this protein must also play a role in PT phosphate reabsorption, as inactivating NPT2c mutations lead to renal phosphate wasting and the autosomal recessive disorder hereditary hypophosphatemic rickets with hypercalciuria (HHRH) .
Disorders of elevated FGF23
The biochemical abnormalities in hFTC include hyperphosphatemia, increased %TRP and inappropriately normal or elevated 1,25D concentrations. HFTC can be considered as the clinical converse of several diseases characterised by elevation of the phosphaturic hormone FGF23. The FGF23 gene, composed of three exons encoding a 251 residue polypeptide, is located on the human chromosome 12p13 . Although FGF23 messenger RNA (mRNA) can be detected at low levels in many tissues including heart, liver, thyroid/parathyroid and small intestine, it is predominantly expressed in bone by osteoblasts, osteocytes, flattened bone lining cells, and osteoprogenitor cells . The manifestations of prolonged elevations in FGF23 include reduced serum phosphorus concentrations due to isolated renal phosphate wasting, inappropriately low or normal serum 1,25D concentrations with typically normal serum calcium and PTH concentrations and osteomalacia/rickets.
Autosomal-dominant hypophosphatemic rickets (ADHR) is an allelic disorder to hFTC (see below) and caused by gain-of-function mutations in the FGF23 gene . These mutations replace the arginine (R) residues at positions 176 or 179 with glutamine (Q) or tryptophan (W) within a 176 RXXR 179 /S 180 subtilisin-like proprotein convertase (SPC) site that separates the conserved FGF-like N -terminal domain from the variable C -terminal tail, and lead to stabilisation of the intact, bioactive FGF23 molecule . Injection of intact FGF23 or purified N – and C -terminal fragments into rodents showed that only the intact FGF23 species caused hypophosphatemia, consistent with the idea that the intact form of FGF23 is bioactive. In patients with X-linked hypophosphatemic rickets (XLH; loss of function PHEX mutations), and autosomal recessive hypophosphatemic rickets (ARHR; loss-of-function DMP1 or ENPP1 mutations), wild-type FGF23 can be markedly elevated. In addition, the corresponding mouse models of these diseases, Hyp (XLH) and Dmp1 -null (ARHR), have increased FGF23 mRNA expression and serum protein concentrations, potentially due to improper osteoblast to osteocyte differentiation . The acquired disorder tumour-induced osteomalacia (TIO) is associated with FGF23 overexpression by a rare category of neoplasm, classified as phosphaturic mesenchymal tumours of the mixed connective tissue variant (PMTMCT) .
Bioactivity of FGF23
FGF23 is central to renal phosphate and vitamin D metabolism, and has emerging roles in the regulation of PTH. FGF23-dependent control of renal phosphate reabsorption was demonstrated by the finding that mice transgenic for human FGF23 had markedly increased phosphate excretion secondary to decreased PT Npt2a and Npt2c expression . In normal individuals, hypophosphatemia is typically a strong stimulator for increased serum 1,25D . However, in patients with syndromes caused by elevated FGF23, there is a lack of appropriate elevation of 1,25D in response to hypophosphatemia. Animal studies demonstrated that in spite of severe hypophosphatemia, FGF23 transgenic mice demonstrated decreased renal levels of Cyp27b1 and increased Cyp24 . Thus, the effects of FGF23 on the renal vitamin D metabolic enzymes account for the reductions in serum 1,25D concentrations observed in TIO, ADHR and XLH patients. More recently, short-term treatment with FGF23 has been shown to suppress PTH mRNA and protein production in vitro in cultured bovine parathyroid cells, and in vivo in intact rat parathyroid glands .
TC due to loss of function in FGF23-related genes
hFTC is a genetically heterogeneous syndrome, caused by loss-of-function mutations in genes relevant to the production of the intact, bioactive form of FGF23 ( FGF23 and GalNAc transferase 3 ( GALNT3 )), and to the end-organ effects of FGF23 bioactivity ( αKlotho ). Human genetic studies, taken together with analyses of corresponding animal models, have provided key insight into the molecular etiology of hFTC.
TC due to FGF23 loss-of-function mutations
Using a candidate gene approach, several groups demonstrated that the hyperphosphatemic TC phenotype can be caused by recessive mutations in the FGF23 gene. These mutations replace conserved residues in the N -terminal FGF-like domain of the mature FGF23 ( Tables 1 and 2 ) and appear to destabilise the bioactive intact form of the hormone. In this regard, the use of multiple FGF23 serum assays with overlapping specificities has provided critical insight into the molecular mechanisms associated with TC. FGF23 can be measured in the serum using two distinct enzyme-linked immuno-sorbent assays (ELISAs). The ‘intact’ FGF23 assay (Kainos, Inc., Redwood City, CA, USA) uses conformation-specific monoclonal antibodies to N – and C -terminal portions of FGF23 that detect the intact, bioactive form of FGF23 . The ‘ C -terminal’ FGF23 ELISA (Immutopics, Int’l. Inc., San Clemente, CA, USA) uses polyclonal antibodies that bind two different peptide epitopes, both ‘ C -terminal’ (3′) to the FGF23 176 RXXR 179 /S 180 proteolytic cleavage site, and thus recognises both the intact FGF23 and the C -terminal fragments present in the circulation .
Syndrome | Gene | Mutation | Protein | Reference |
---|---|---|---|---|
hFTC | FGF23 | 123c > a | H41Q | Masi 2005 |
hFTC | FGF23 | 160c > a | Q54K | Garringer 2008 |
hFTC | FGF23 | 287t > c | M96T | Chefetz 2005 |
hFTC | FGF23 | 211a > g | S71G | Larsson 2005, Benet-Pages 2005 |
hFTC | FGF23 | 367g > t | G123W | Lammoglia 2008 |
hFTC | FGF23 | 385t > c | S129P | Bergwitz 2005 |
hFTC | FGF23 | 386c > t | S129F | Araya 2005 |
HHS | GALNT3 | 2t > a | M1K | Gok 2009 |
hFTC | GALNT3 | 41–58del | R14fsX21 | Garringer 2006 |
hFTC | GALNT3 | 484c > t | R162X | Topaz 2004, Ichikawa 2005 |
hFTC | GALNT3 | 485g > a | R162Q | Ichikawa 2010 |
hFTC | GALNT3 | 516-2a > t | (Splice Site) | Ichikawa 2005 |
hFTC | GALNT3 | 677delC | A226VfsX3 | Ichikawa 2010 |
hFTC | GALNT3 | 815c > a | T272K | Ichikawa 2006 |
HHS | GALNT3 | 803_804insC | A268fsX271 | Ichikawa 2007 |
HHS | GALNT3 | 839g > a | C280Y | Gok 2009 |
hFTC | GALNT3 | 842a > g | E281G | Joseph 2010 |
hFTC | GALNT3 | 966t > a | Y322X | Barbieri 2007 |
hFTC | GALNT3 | 1076c > a | T359K | Ichikawa 2006 |
hFTC | GALNT3 | 1097t > g | L366R | Joseph 2010 |
hFTC | GALNT3 | 1102_1103inst | E375X | Garringer 2007 |
hFTC | GLANT3 | 1245t > a | H415Q | Yancovitch 2011 |
hFTC | GALNT3 | 1312c > t | R438C | Dumitrescu 2008 Yancovitch 2011 |
HHS | GALNT3 | 1313g > a | R438H | Olauson 2008 |
hFTC | GALNT3 | 1387a > t | K463X | Campagnoli 2006 |
HHS | GALNT3 | 1392 + 1g > a | (Splice Site) | Ichikawa 2010 |
hFTC | GALNT3 | 1441ct | Q481X | Barbieri 2007 |
hFTC | GALNT3 | 1460 g > a | W487X | Garringer 2007 |
hFTC /HHS | GALNT3 | 1524 + 1g > a | K465_Y508del | Topaz 2004, Fishburg 2005 |
hFTC | GALNT3 | 1524 + 5 g > a | (Splice Site) | Topaz 2004 |
HHS | GALNT3 | 1626 + 1g > a | (Splice Site) | Ichikawa 2007 |
hFTC | GALNT3 | 1720t > g | C574G | Ichikawa 2010 |
hFTC | GALNT3 | 1774c > t | Q592X | Specktor 2006 |
hFTC | αKlotho | 578a > g | H193R | Ichikawa 2007 |
Treatment | Mechanism | Urinary phosphate | Serum phosphate | Serum calcium | Serum 1,25D | Tumoral calcinosis lesions |
---|---|---|---|---|---|---|
Dietary phosphate restriction | Decrease phosphate load | ↓ | ↓ | ↔ | ↑↔ | ↓↔ |
Phosphate binders | Decrease phosphate load | ↓ | ↓ | ↔ | ↑↔ | ↓↔ |
Acetazolamide | Increase phosphate excretion | ↑ | ↓ | ↔ | ↔ | ↓↔ |
Calcitonin | Increase phosphate excretion | ↑ | ↓in TC (but↑ in XLH) | ↔↓ | ↑ | ↓↔ |
The results of the two ELISAs generally correlate well with regard to the relative range of FGF23 concentrations in XLH and in TIO patients, and in normal individuals . By contrast, the serum from hFTC patients with S71G and S129F FGF23 mutations revealed low-normal intact FGF23 levels , whereas C -terminal FGF23 concentrations were markedly elevated . Taken together, these observations suggested a differential proteolytic cleavage of hFTC-mutant FGF23 to that of wild-type protein. Indeed, it has been demonstrated that the hFTC mutations lead to increased intra-cellular proteolytic degradation . This activity is likely mediated, at least in part, by furin-like proteases which reside in the trans-Golgi network (TGN) and recognize the FGF23 176 RXXR 179 /S 180 SPC site. The apparent loss of secretion of intact bioactive FGF23 in patients with hFTC explains the biochemical phenotype. With impaired intact FGF23 secretion, there is continued renal reabsorption of phosphate and a lack of inhibition of 1,25D production ( Fig. 1 ) despite hyperphosphatemia. These alterations likely lead to further increased intestinal calcium and phosphate absorption, creating a biochemical environment favourable for precipitation of calcium-phosphate crystals in the vasculature and soft tissues. In accord with the human findings, the human hFTC phenotype closely resembles that of FGF23 -null mice, which manifest hyperphosphatemia, elevated 1,25D and ectopic and vascular calcifications.
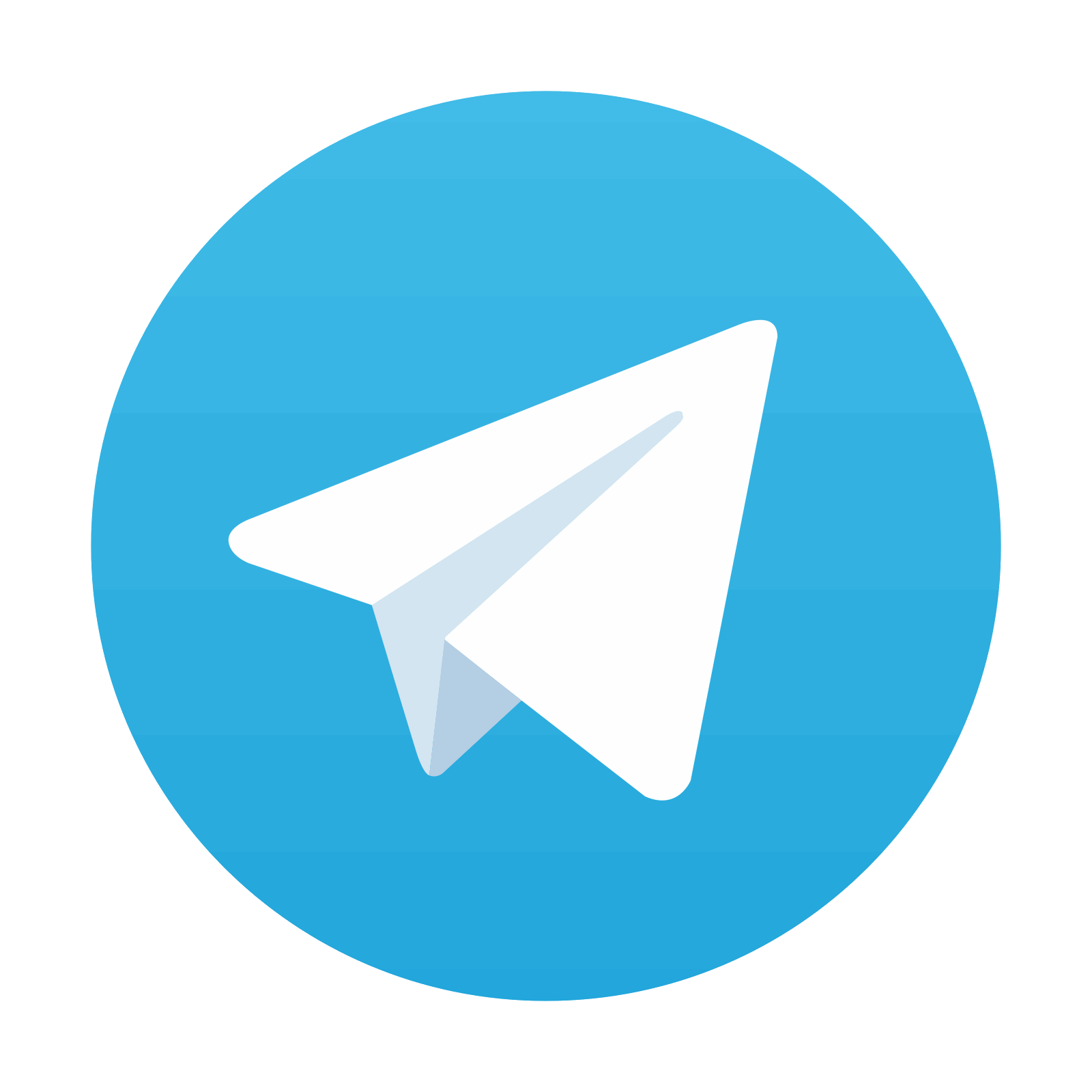
Stay updated, free articles. Join our Telegram channel

Full access? Get Clinical Tree
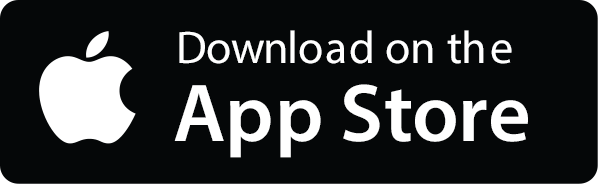
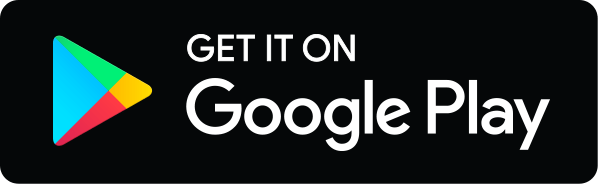
