Genetic haemochromatosis is a hereditary disease characterised by tissue iron overload. In Caucasians it is most often due to homozygous C282Y HFE gene mutation, but other genes may be involved. Without treatment by venesections, patients can develop life-threatening visceral damage such as liver cirrhosis and carcinoma, diabetes or heart failure. This treatment has been remarkably successful in preventing these complications, but patients survive with other symptoms of the disease susceptible to impair, sometimes seriously, their quality of life. This is the case of arthropathy and osteoporosis complicating haemochromatosis. In this chapter, focus has been placed on the rheumatological complications of genetic haemochromatosis.
Genetic haemochromatosis (GH) is a hereditary disease expressed by tissue iron overload. It is due to an intestinal hyperabsorption of iron. The most common genotype is homozygosity for the p.Cys282Tyr mutation of the HFE gene (MIM 235200). However, other genetic conditions have been described. The central role of hepcidin is now recognised. Before phlebotomy therapy, GH was a serious disease which could lead to vital complications such as cirrhosis, liver carcinoma, heart failure or diabetes mellitus because of iron overload. Currently, these complications can be prevented by regular blood removal. However, despite treatment by phlebotomies, patients’ quality of life is often altered by the rheumatological conditions of the disease, particularly joint symptoms and sometimes osteoporosis. That is why rheumatologists are in first line to diagnose the disease and to treat bone and joint damage.
Genetics and physiopathology
GH was originally reported by Trousseau in 1865 . The major finding was the presence of a triad associating cirrhosis and diabetes in a tanned man. The picture of the disease has been progressively completed until now. Von Recklinghausen reported, in 1889 , the presence of strong iron deposits within the liver of those patients, thus leading him to evoke the role of iron excess in the disease occurrence. He named the disease haemochromatosis. Thereafter, a large contradictory debate was initiated to identify the etiology of this ‘idiopathic’ iron overload occurring out of haematological disease and iron supplementation. Some people supported the role of associated factors, such as alcohol, in its development, leading to the hypothesis of secondary iron overload linked to excessive alcohol intake. However, others supported the hypothesis of a primary iron overload disease linked to genetic factors . Simon et al. definitively demonstrated in 1976 that the original clinical picture was related to genetic factors, linking the disease to chromosome 6, more specifically in area p21, close to HLA genes which were subsequently used for family studies for 20 years . In addition, he demonstrated that the transmission of this GH was recessive. At the same time, the clinical description of GH was completed especially with the reports of patients exhibiting cardiac complications, osteoporosis and arthritis, hypogonadism and dermoskeleton alteration .
Understanding of the pathophysiology of the development of iron overload and its complications is a major challenge to improve the follow-up of haemochromatosis patients as well as to increase, from this ‘model disease’, our knowledge of iron metabolism.
Normal iron metabolism
Iron, a transition metal, is required for cell life. It is involved in a large number of biological functions, especially in oxygen transport when associated with haemoglobin, and also in the activity of a large number of enzymes, requiring iron as a cofactor and playing a role in the different metabolisms. For this purpose, iron must be present in sufficient concentrations and adequately distributed to the different cell types requiring the presence of iron .
The total amount of iron in the body is close to 4 g for an adult. Such a quantity of iron must be strictly controlled to ensure that the amount of iron is sufficient, to avoid manifestations linked to iron deficiency, and not excessive to limit its potential toxicity. The control of iron stores and distribution involves a large number of genes encoding proteins, thus ensuring iron metabolism homeostasis. Mutations in some of these genes may promote the development of genetic iron overload . We will briefly describe iron metabolism before the presentation of GH pathophysiology.
Plasma plays a major role in iron metabolism. Indeed, despite the fact that plasma iron concentration is low (12–25 μM), this iron form is the only one available for cells. Therefore, the control of plasma iron is a major goal. The main sources of iron for plasma are macrophages and enterocytes. The control of iron import in plasma from these cells involves hepcidin.
Macrophages are involved in recycling iron from erythrocytes . Indeed, erythrocytes concentrate 70% of the body iron for incorporation into haemoglobin. When erythrocytes reach their lifespan, they undergo the erythrophagocytic process within macrophages. Haem is then extracted from erythrocytes and iron is released under the haem oxygenase action. Iron can therefore be stored in macrophages within ferritin – the iron-storage protein which may contain up to 4500 iron atoms under a chemically inactive form – or released in plasma through the ferroportin protein. Ferroportin is a protein encoded by the SLC40A1 gene and located in the cell membrane . It transfers ferrous atoms of iron (Fe 2+ ) from the macrophage cytoplasm towards plasma. In addition, iron must be oxidized to Fe 3+ (the ferric form of iron) to be taken in charge by transferrin, the plasma iron transport protein. Each molecule of transferrin may link two atoms of iron. Ceruloplasmin, a multicopper oxidase synthesised by the liver, ensures this oxidase activity . A transferrin saturation coefficient, corresponding to the ratio of plasma iron concentration versus total plasma transferrin concentration, can be calculated (normal range values 30–45%). The quantity of iron released daily by macrophages is close to 20 mg and ensures the maintenance of the plasma iron level throughout the day.
Enterocytes also play a role in the maintenance of iron concentration in plasma and therefore of iron stores. Indeed, every day, there are nonregulated and incompressible losses of iron, especially through cell desquamation, urine, feces and menses in women . Therefore, iron must be extracted from nutrients and absorbed to compensate for its losses. In addition, pregnancy and growth phases require more iron. Iron absorption takes place in the duodenum at the apical pole of enterocytes, through mechanisms involving specific transporters . Iron is then either addressed to ferritin and stored within the enterocyte, or directed towards the basolateral pole of the enterocyte. When stored in the enterocyte, iron is not absorbed due to enterocyte desquamation into digestive lumen. When addressed to basolateral pole of enterocyte, atoms of iron are in a position to be released in plasma, through the ferroportin protein . Like in macrophages, an oxidation phase occurs thereafter to ensure the association of iron to transferrin. This is performed by hephastin, a glycosylphosphatidylinositol (GPI) -anchored protein with ferroxidase activity .
Hepcidin is a peptide of 25 amino acids in its mature form, synthesised by hepatocytes and secreted in plasma . It targets the ferroportin protein which is especially located in macrophages and enterocytes. When interacting with ferroportin, hepcidin promotes its internalisation and then its degradation through the proteasome. Therefore, the hepcidin level, by limiting ferroportin expression at the cell membrane, controls the iron leakage into plasma and modulates both plasma iron and transferrin saturation levels. The plasma hepcidin level is regulated by various stimuli. Thus, secondary iron excess induces hepcidin expression through a molecular cascade involving the Bone Morphogenetic Protein 6 (BMP6) produced by iron-loaded hepatocytes and interacting at the cell membrane with a complex associating a specific BMP receptor , composed of two subunits, and haemojuvelin as a co-receptor. Then, phosphorylated BMP receptors induce a phosphorylation of cytoplasmic SMAD 1/5/8 proteins which then interact with SMAD4. The SMAD1/5/8/4 complex is translocated to the nucleus and interacts with a specific BMP-responsive element in the hepcidin promoter, thus inducing transcription of the hepcidin mRNA . The increase of plasma hepcidin concentration limits ferroportin expression at the cell membrane and therefore decreases the plasma iron levels to counteract the iron excess.
Other genes also play a role in the signal transduction related to iron: the HFE gene product is expressed at the cell membrane in association with the beta2 microglobulin and favours the signal linked to BMP6 ; the gene TFR2, coding the transferrin receptor 2, also plays a role in the iron-related regulation of hepcidin expression . Despite the demonstration of the positive role of these two genes in the control of hepcidin expression, precise mechanisms are not fully characterised. Inflammation also strongly promotes hepcidin expression, especially due to the increase of interleukin-6 levels which stimulate, through the cell receptor, the STAT3 pathway and therefore the transcription of the hepcidin gene, thanks to a specific binding site for phosphorylated form of STAT3 in the hepcidin promoter . During inflammation, such abnormally high hepcidin levels regarding the iron stores promote iron retention in macrophages and limit iron absorption. This plays a role in the development of anaemia related to chronic diseases. Conversely, hepcidin levels are decreased by hypoxia and anaemia. Mechanisms could involve i) the hypoxia transcription factor hypoxia-inducible transcription factor (HIF) and ii) a soluble signal linked to the increase of erythropoietic activity required to compensate hypoxia. The role of growth developmental factor 15 (GDF15) has been evoked .
Pathophysiology of iron overload in GH
The original description of GH corresponds to the disease related to the homozygous p.Cys282Tyr mutation in HFE gene which represents more than 95% of GH. However, other causes of GH have been described more recently. Most of the cases of GH are related to an inappropriately low hepcidin level leading to increased iron leakage from macrophages and enterocytes and then to an increase of plasma iron and transferrin saturation. Other types of GHs are linked to ferroportin gene mutations.
Hepcidin deficiency
Hepcidin deficiency has been described in haemochromatosis linked to mutations in hepcidin, haemojuvelin, HFE or transferrin receptor 2 genes . This leads to a plasma iron increase favouring the appearance of non-transferrin-bound iron (NTBI) which plays an important role in the development of iron overload .
Genes involved in hepcidin deficiency
It is obvious that homozygous or compound heterozygote mutations in the coding sequence of the hepcidin gene itself strongly inhibit hepcidin expression, therefore leading to extremely severe GH with juvenile expression. Fortunately, such mutations are exceptional . It is noteworthy that some mutations in the 5′-untranslated region of the mRNA or in the hepcidin promoter may have an impact on hepcidin expression .
Mutations (homozygous or compound heterozygote) in the haemojuvelin gene are very rare and also induce a juvenile form of GH . This impact is related to the major role of haemojuvelin protein in the transduction of BMP6-related signaling in hepcidin expression regulation .
The homozygous p.Cys282Tyr (C282Y) mutations of the HFE gene also favor the development of a situation of abnormally low hepcidin levels, leading to the development of iron overload . This mutation is found at the heterozygous state in 10% of the Caucasian population, and 3 per 1000 are homozygous and therefore exposed to the risk of iron overload development. The mutation alters the signal transduction linked to BMP6 . However, the penetrance of the disease is quite variable , ranging from an absence of bioclinical expression to severe organ damage compromising life expectancy. This suggests that other environmental or genetic factors could play a role in the penetrance of the disease. It is noteworthy that the p.His63Asp (H63D) polymorphism alone, present in 30% of Caucasians, does not induce the development of iron overload. Other rare deleterious mutations or deletions have been reported .
Homozygous or compound heterozygous mutations in the TFR2 gene also induce low levels of hepcidin by mechanisms not yet fully understood. It is noteworthy that some cases of the disease have been recorded in younger patients compared to HFE-related haemochromatosis .
Role of NTBI in the development of iron overload
When transferrin saturation increases, NTBI may appear in plasma . In normal situations, plasma iron is linked to transferrin, a protein secreted by hepatocytes, which ensures iron delivery to cells. Mechanisms of uptake implicate transferrin receptor 1, a cell membrane protein linking transferrin and allowing its endocytosis. This mechanism is controlled by the iron regulatory protein (IRP)/iron-responsive element (IRE) couple. In a condition of cell iron excess, the cytoplasmic IRPs do not further interact with the IREs which are nucleotidic sequences localised in the 3′-untranslated region of the transferrin mRNA. This allows degradation of transferrin mRNA and then limits transferrin iron uptake in condition of cellular iron excess . NTBI uptake is not downregulated in such situations . Therefore, iron continues to enter the cell despite iron overload. NTBI being especially uptaken by liver, pancreas and heart, this explains why it plays a key role in the development of iron overload.
Ferroportin disease
Ferroportin is the target of hepcidin, and mutations in the SLC40A1 gene also induce GH . It is noteworthy that the transmission of the disease is dominant. The mutation may have two different impacts which correspond to a loss or a gain of function.
Loss of function is the most frequent consequence of the mutation . In this case, the mutation limits either the expression of the protein at the cell membrane or its capacity to export iron to plasma. Therefore, iron egress is limited especially from macrophages which are overloaded. This explains that the phenotype of the disease is characterised by plasma iron and transferrin saturation which are either normal or decreased. Hepatocytes may also present some degree of iron overload which could be related to the fact that some of them express low levels of ferroportin or to another unknown mechanism, NTBI being undetectable in those patients. In addition, despite the decrease of ferroportin function, digestive iron absorption is not abolished, suggesting that other mechanisms involved in iron uptake may exist.
Gain of function applies to a few cases. The ferroportin gene mutation leads to structural changes with impossibility for hepcidin to interact with ferroportin which keeps its iron export capacity. Then, the disease is characterised by a phenotypic presentation similar to hepcidin deficiency with increase of plasma iron and transferrin saturation.
Diagnosis and phenotypic classification
Iron overload is suspected in two situations: in the presence of clinical symptoms (knowing that many of them are non-specific), and, most often, when plasma transferrin saturation is increased (in theory >45%, but in practice often >60% in men and >50% in women) or plasma ferritin is >300 μg l –1 in men and >200 μg l –1 in women.
Clinical symptoms suggestive of GH are: chronic asthenia, arthralgia, arthritis, chondrocalcinosis, skin pigmentation, hepatomegaly, unexplained liver disease, type 1 diabetes, osteopenia or osteoporosis, and cardiac symptoms (rhythm disturbances, cardiac failure).
For HFE -related haemochromatosis (type 1 haemochromatosis), the diagnosis is based on the association of increased transferrin saturation (TS) and the p.Cys282Tyr mutation in a homozygous state.
However, clinicians have to diagnose non-HFE haemochromatosis and rule out hyperferritinemia not related to haemochromatosis by checking for the main causes of non-haemochromatosis ferritin increase such as alcohol, inflammation, cell necrosis or dysmetabolic iron overload syndrome. A decision tree is proposed in Fig. 1 adapted from , based on suggestive symptoms and signs and/or hyperferritinemia.

Briefly, if TS is >45%, HFE testing is performed to find C282Y homozygous patients or, more rarely, compound heterozygote patients C282Y/H63D who have a much lower chance of developing iron overload in the absence of other environmental factors. When no mutations for HFE are found, non-HFE haemochromatosis is to be considered (type 2, 3 and 4B).
If TS is <45% and hyperferritinemia not related to haemochromatosis is ruled out, liver iron content has to be evaluated by magnetic resonance imaging (MRI) or biopsy. In the case of hepatic iron concentration (HIC) increase, ferroportin disease or hereditary aceruloplasminemia can be considered; if HIC is normal, the hyperferritin-cataract syndrome has to be investigated.
A phenotypic five-scale grading has been proposed to summarise the impact of the disease ( Fig. 2 ) and to make a decision for treatment. Stage 0 : genetic predisposition (genotype) without any clinical phenotype; stage 1 : stage 0 + isolated increased transferrin saturation (>45%); stage 2: increase in both transferrin saturation and serum ferritin (>200 μg l –1 in women and >300 μg l –1 in men): this is the cut-off for treatment; stage 3 : decrease of quality of life due to asthenia and/or arthropathy; and stage 4 : life-threatening symptoms such as liver cirrhosis, diabetes, cardiomyopathy and hepatocellular carcinoma.

The French National Health Authorities (Haute Autorité de Santé (HAS)) published recommendations (with an English version) for assessment of patients with type 1 haemochromatosis according to the phenotypic scale ( http://www.has-sante.fr/portail/upload/docs/application/pdf/hemochromatosis_guidelines_2006_09_12__9_10_9_659.pdf ).
Stage 0 and 1 . No tests are required, but a careful physical examination looking for asthenia, melanodermia, arthralgia and familial haemochromatosis is necessary. Ferritin and TS should be assessed every 3–5 years or in the case of new clinical signs.
Stages 2, 3 and 4. In addition to serum iron overload assessment and physical examination, four organs are targeted:
- –
Liver : Transaminases, liver ultrasound in the case of clinical hepatomegaly or transaminase increase. In the case of transaminase increase, hepatomegaly or ferritin above 1000 μg l −1 a liver biopsy is indicated. This is not for the diagnosis of haemochromatosis which is made on both serum iron parameters and genotyping, but to detect liver fibrosis and especially cirrhosis.
- –
Gonads : Hypogonadism is assessed in males, clinically and biologically with testosterone dosage.
- –
Bone : Dual X-ray absorptiometry (DXA) is indicated to diagnose osteopenia or osteoporosis in the case of osteoporosis cofactors such as hypogonadism in males and osteoporosis in females or cirrhosis.
- –
Heart : An echocardiography is required.
Diagnosis and phenotypic classification
Iron overload is suspected in two situations: in the presence of clinical symptoms (knowing that many of them are non-specific), and, most often, when plasma transferrin saturation is increased (in theory >45%, but in practice often >60% in men and >50% in women) or plasma ferritin is >300 μg l –1 in men and >200 μg l –1 in women.
Clinical symptoms suggestive of GH are: chronic asthenia, arthralgia, arthritis, chondrocalcinosis, skin pigmentation, hepatomegaly, unexplained liver disease, type 1 diabetes, osteopenia or osteoporosis, and cardiac symptoms (rhythm disturbances, cardiac failure).
For HFE -related haemochromatosis (type 1 haemochromatosis), the diagnosis is based on the association of increased transferrin saturation (TS) and the p.Cys282Tyr mutation in a homozygous state.
However, clinicians have to diagnose non-HFE haemochromatosis and rule out hyperferritinemia not related to haemochromatosis by checking for the main causes of non-haemochromatosis ferritin increase such as alcohol, inflammation, cell necrosis or dysmetabolic iron overload syndrome. A decision tree is proposed in Fig. 1 adapted from , based on suggestive symptoms and signs and/or hyperferritinemia.
Briefly, if TS is >45%, HFE testing is performed to find C282Y homozygous patients or, more rarely, compound heterozygote patients C282Y/H63D who have a much lower chance of developing iron overload in the absence of other environmental factors. When no mutations for HFE are found, non-HFE haemochromatosis is to be considered (type 2, 3 and 4B).
If TS is <45% and hyperferritinemia not related to haemochromatosis is ruled out, liver iron content has to be evaluated by magnetic resonance imaging (MRI) or biopsy. In the case of hepatic iron concentration (HIC) increase, ferroportin disease or hereditary aceruloplasminemia can be considered; if HIC is normal, the hyperferritin-cataract syndrome has to be investigated.
A phenotypic five-scale grading has been proposed to summarise the impact of the disease ( Fig. 2 ) and to make a decision for treatment. Stage 0 : genetic predisposition (genotype) without any clinical phenotype; stage 1 : stage 0 + isolated increased transferrin saturation (>45%); stage 2: increase in both transferrin saturation and serum ferritin (>200 μg l –1 in women and >300 μg l –1 in men): this is the cut-off for treatment; stage 3 : decrease of quality of life due to asthenia and/or arthropathy; and stage 4 : life-threatening symptoms such as liver cirrhosis, diabetes, cardiomyopathy and hepatocellular carcinoma.
The French National Health Authorities (Haute Autorité de Santé (HAS)) published recommendations (with an English version) for assessment of patients with type 1 haemochromatosis according to the phenotypic scale ( http://www.has-sante.fr/portail/upload/docs/application/pdf/hemochromatosis_guidelines_2006_09_12__9_10_9_659.pdf ).
Stage 0 and 1 . No tests are required, but a careful physical examination looking for asthenia, melanodermia, arthralgia and familial haemochromatosis is necessary. Ferritin and TS should be assessed every 3–5 years or in the case of new clinical signs.
Stages 2, 3 and 4. In addition to serum iron overload assessment and physical examination, four organs are targeted:
- –
Liver : Transaminases, liver ultrasound in the case of clinical hepatomegaly or transaminase increase. In the case of transaminase increase, hepatomegaly or ferritin above 1000 μg l −1 a liver biopsy is indicated. This is not for the diagnosis of haemochromatosis which is made on both serum iron parameters and genotyping, but to detect liver fibrosis and especially cirrhosis.
- –
Gonads : Hypogonadism is assessed in males, clinically and biologically with testosterone dosage.
- –
Bone : Dual X-ray absorptiometry (DXA) is indicated to diagnose osteopenia or osteoporosis in the case of osteoporosis cofactors such as hypogonadism in males and osteoporosis in females or cirrhosis.
- –
Heart : An echocardiography is required.
Different types of hereditary haemochromatosis
Type 1 haemochromatosis
This is by far the most frequent form of haemochromatosis. It concerns Caucasians and especially Celtic populations. It is due to the p.Cys282Tyr (C282Y) mutation of the HFE gene, located on chromosome 6. Homozygosity is the condition for developing the disease, even if the clinical penetrance is far from being 100%. Compound heterozygosity C282Y/H63D does not lead to clinical iron overload . One woman in one hundred and 25% of men with C282Y homozygosity have a phenotype of iron overload . This means that other genetic and environmental factors are needed to develop the disease and are susceptible to modify the disease expression. Combinations with mutations in HAMP or HJV genes are rare. BMP2 variants have been found to increase the penetrance of C282Y homozygosity. However, these situations are rare . Alcohol abuse, transfusions, dysmetabolic hepatosiderosis or chronic iron supplementation may represent environmental factors.
Type 2 haemochromatosis
It is also known as ‘juvenile hemochromatosis’. Mutations on two different genes can be involved. The type 2A hemochromatosis is related to mutations in the hemojuvelin gene ( HJV ) located on chromosome 1. Type 2B hemochromatosis is characterised by hepcidin gene ( HAMP ) mutations. This leads to an early and massive iron overload with predominant heart and endocrine damages. Fortunately, this type remains exceptional.
Type 3 haemochromatosis
It is a rare form of hemochromatosis related to mutations of transferrin receptor 2 ( TFR2 ). Phenotypic expression resembles that of type 1 hemochromatosis.
Type 4 haemochromatosis
It is more frequent than type 2 and 3 hemochromatosis. It is also called ‘ferroportin disease’ and is related to mutations in the ferroportin gene ( SCL40A1 ) on chromosome 2. It is the only type of hemochromatosis transmitted in an autosomal dominant way. The 4 A type is due to mutations on the SCL40A1 gene resulting in a loss of function and haemochromatosis with iron overload in the reticuloendothelial system: spleen macrophages and Kupffer cells with normal or low plasma iron and transferrin saturation. The 4 B type makes the ferroportin resistant to hepcidin which results in a gain of function leading to a permanent release of iron in the plasma. Recent data suggest a preeminent role of sex and environmental or acquired factors in the phenotypic expression of the disease .
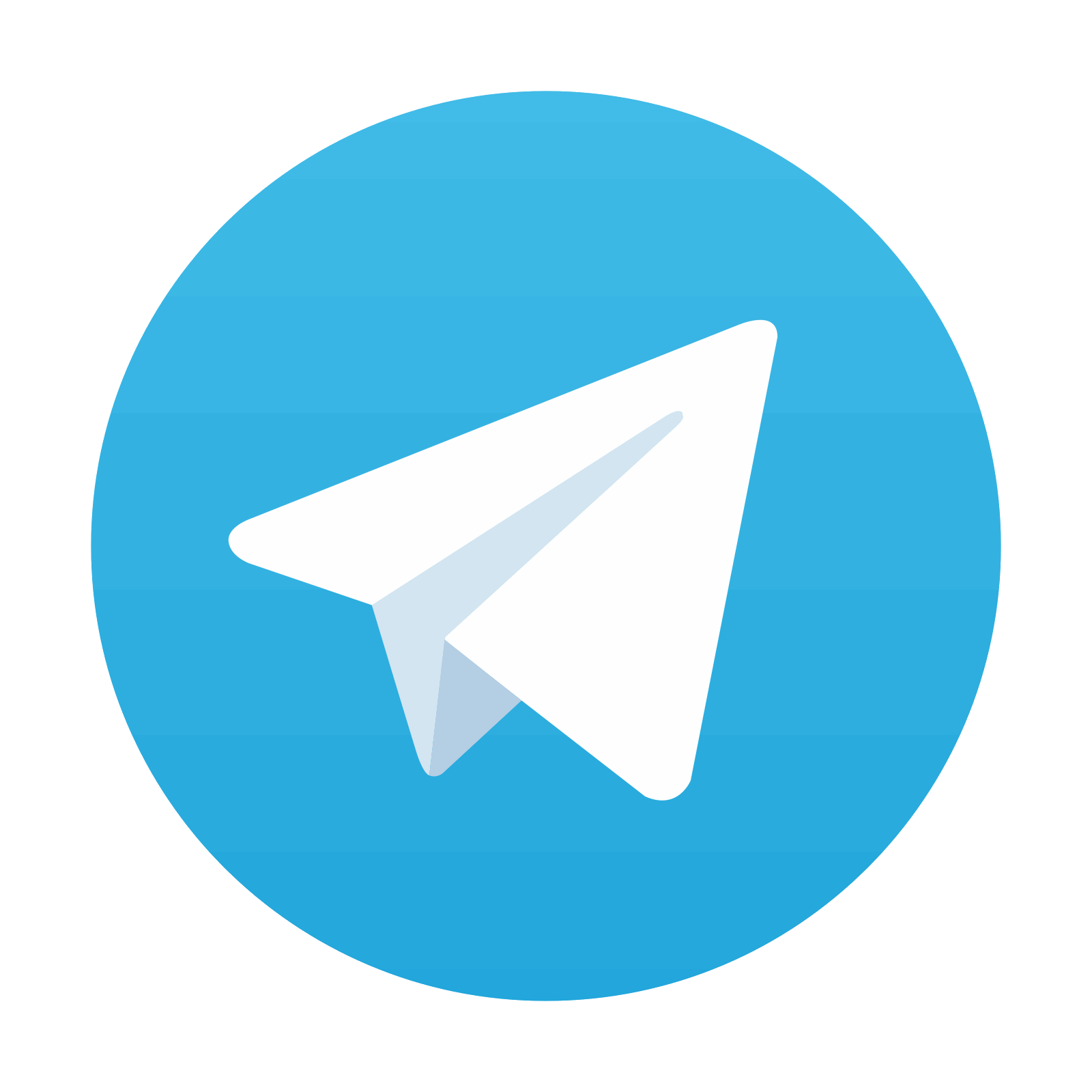
Stay updated, free articles. Join our Telegram channel

Full access? Get Clinical Tree
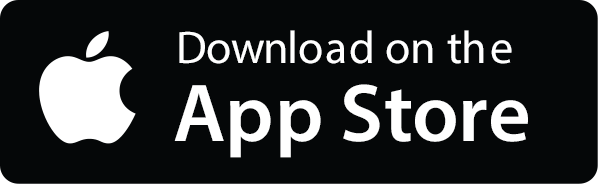
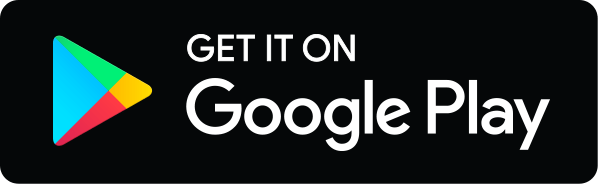