Amyotrophic lateral sclerosis (ALS) was initially known as Charcot’s sclerosis, named after the French neurobiologist and physician Jean-Martin Charcot who first described this type of muscular atrophy in the early nineteenth century. In the United States, ALS became widely known as Lou Gehrig’s disease after the famous baseball player who succumbed to the disease in the late 1930s. Currently, ALS is the most common motor neuron disease, with a worldwide incidence of 8 cases per 100,000 population per year. Familial forms constitute approximately 5% to 10% of all cases. Onset increases with age, with a peak in the seventh decade and a slight preponderance (relative risk, 1.3–1.5) among men compared with women. Rapid progression of motor neuron loss leads to death an average of 3 to 5 years after symptom onset. The cause of ALS remains unknown and there is still no curative therapy.
Amyotrophic lateral sclerosis
Amyotrophic lateral sclerosis (ALS), initially known as Charcot’s sclerosis , was named after the French neurobiologist and physician Jean-Martin Charcot who first described this type of muscular atrophy in the early nineteenth century . In the United States, ALS became widely known as Lou Gehrig ‘ s disease after the famous baseball player who succumbed to the disease in the late 1930s. Currently, ALS is the most common motor neuron disease, with a worldwide incidence of 8 cases per 100,000 population per year . Familial forms constitute approximately 5% to 10% of all cases. Onset increases with age, with a peak in the seventh decade and a slight preponderance among men compared with women. Rapid progression of motor neuron loss leads to death an average of 3 to 5 years after symptom onset . The cause of ALS remains unknown and there is still no curative therapy.
The term amyotrophy refers to peripheral denervation producing lower motor neuron signs of weakness, muscular atrophy, and fasciculations. Upper motor neuron signs of spasticity and hyperreflexia are appreciated as the disease advances, because of sclerosis of the lateral corticospinal tracts. Symptom onset is typically characterized by weakness or clumsiness in an upper extremity with or without fasciculations. In a subset of cases, presenting weakness in the bulbar musculature leads to progressive dysphagia and dysarthria. Bulbar onset infers a rapidly poor prognosis with life expectancy 1 to 2 years after the onset of symptoms.
As the disease progresses, widespread limb and trunk weakness leads to a rapid decline in physical function. Regardless of the initial site of disease manifestation, weakness in the bulbar and respiratory muscles places patients at risk for aspiration pneumonia, which is often the eventual cause of death.
Although the events leading to neuronal toxicity are not clearly understood, ALS is now widely accepted to most likely be a multifactorial disease. Many factors, not necessarily related, have been shown to participate in motor neuron cell death in ALS, including glutamate toxicity, oxidative stress, neurofilament accumulation and neuroinflammation . These pathways are not mutually exclusive and may act individually or in unison to cause motor neuron disease .
Diverse neurologic disorders such as Alzheimer’s disease, Parkinson’s disease, HIV-associated dementia, and other neurodegenerative diseases share common pathologic themes. Understanding these commonalities may help in developing new neuroprotective strategies . One common feature is neuroinflammation.
Key players in neuroinflammation
Microglia
The primary mediators of neuroinflammation are microglia cells, the resident macrophage-like population of the central nervous system (CNS) . Studies in the past 2 decades have shown involvement of microglia in many acute and chronic neurologic diseases, such as stroke, trauma, Alzheimer’s disease, and multiple sclerosis .
In the unperturbed, healthy CNS, the microglia phenotype is called resting microglia , characterized by a small cell body with fine, ramified processes and minimal surface-antigen expression. On injury to the CNS, microglia are swiftly activated and participate in the pathogenesis of neurologic disorders. It now is widely accepted that substances released from damaged cells trigger microglial activation, leading to acute responses and long-term reorganization of the cell phenotype .
Once activated, microglia exert their effects on neurons and macroglia (astrocytes and oligodendrocytes) through the release of cytotoxic substances such as oxygen radicals, nitric oxide, glutamate, proteases, neurotoxic cytokines, and cytoprotective agents such as growth factors, plasminogen, plasminogen activator, and neuroprotective cytokines . The effects of microglia are themselves modulated by astrocytes and neurons through cytokines and neurotransmitters, producing complex interactions among microglia, neurons, and astrocytes .
Astrocytes
Astrocytes are the most numerous glial cells. They constitute 50% to 60% of CNS cells and share a common lineage with neurons and oligodendrocytes. Although initially regarded as mere “in-between” neurons ( glia is the Greek word for glue ), astrocytes are now known to come in as many shapes and with as many functions as neurons.
Astrocytes provide crucial metabolic support to neurons , regulate local blood flow depending on neuronal activity , and shape and modulate synaptic strength . They actively maintain the blood–brain barrier and are the key players in removing excess glutamate from the CNS . Together with microglia cells they resemble the innate immune response “squat” , and feedback loops between astrocytes and microglia regulate the expression of pro- and anti-inflammatory cytokines in many neuropathologic conditions .
Key players in neuroinflammation
Microglia
The primary mediators of neuroinflammation are microglia cells, the resident macrophage-like population of the central nervous system (CNS) . Studies in the past 2 decades have shown involvement of microglia in many acute and chronic neurologic diseases, such as stroke, trauma, Alzheimer’s disease, and multiple sclerosis .
In the unperturbed, healthy CNS, the microglia phenotype is called resting microglia , characterized by a small cell body with fine, ramified processes and minimal surface-antigen expression. On injury to the CNS, microglia are swiftly activated and participate in the pathogenesis of neurologic disorders. It now is widely accepted that substances released from damaged cells trigger microglial activation, leading to acute responses and long-term reorganization of the cell phenotype .
Once activated, microglia exert their effects on neurons and macroglia (astrocytes and oligodendrocytes) through the release of cytotoxic substances such as oxygen radicals, nitric oxide, glutamate, proteases, neurotoxic cytokines, and cytoprotective agents such as growth factors, plasminogen, plasminogen activator, and neuroprotective cytokines . The effects of microglia are themselves modulated by astrocytes and neurons through cytokines and neurotransmitters, producing complex interactions among microglia, neurons, and astrocytes .
Astrocytes
Astrocytes are the most numerous glial cells. They constitute 50% to 60% of CNS cells and share a common lineage with neurons and oligodendrocytes. Although initially regarded as mere “in-between” neurons ( glia is the Greek word for glue ), astrocytes are now known to come in as many shapes and with as many functions as neurons.
Astrocytes provide crucial metabolic support to neurons , regulate local blood flow depending on neuronal activity , and shape and modulate synaptic strength . They actively maintain the blood–brain barrier and are the key players in removing excess glutamate from the CNS . Together with microglia cells they resemble the innate immune response “squat” , and feedback loops between astrocytes and microglia regulate the expression of pro- and anti-inflammatory cytokines in many neuropathologic conditions .
Neuroinflammation in amyotrophic lateral sclerosis
Although microglia and neuroinflammation are implicated in nearly all disorders of the CNS, the role of microglial cells in ALS recently came into focus. In histopathologic studies of human ALS tissue, strong activation and proliferation of microglia have been described in the primary motor cortex, brainstem motor nuclei, and ventral horns of the spinal cord: all areas with motor neuron loss . Activated microglia were seen in areas with not only severe motor neuron death but also mild neuronal death . Furthermore, a recent positron emission tomographic (PET) scan study showed that microglial activation strongly correlated with clinical signs of upper motor neuron loss in sporadic ALS .
Evidence implicating activation of microglia in motor neuron disease was also seen in studies on mutant SOD1 (mtSOD1) transgenic mice, the predominant animal model of ALS. These studies showed that microglial activation occurred in the earliest stages of motor neuron degeneration, even before symptoms of weakness . Several groups reported expression of proinflammatory mediators, such as interleukin (IL)-1β, IL-6, cyclooxygenase-2 (COX-2), inducible nitric oxide synthase (iNOS), or tumor necrosis factor (TNF)-α, even preceding the development of clinical signs . The expression profile of these inflammatory molecules was microglia/macrophage-like.
Elevated levels of MCP-1 and IL-6, for example, were also reported in patients who had ALS . Motor neurons are exquisitely sensitive to neuroinflammation. Several studies have shown that mediators associated with neuroinflammation, such as TNF-α, IL-1β, or FAS ligand (FasL), can readily trigger motor neuron apoptosis .
In ALS, in contrast to other neurodegenerative diseases such as multiple sclerosis or Alzheimer’s disease, influx of peripheral immune cells such as lymphocytes or neutrophils is rare and mainly associated with end-stage disease . However, recent progress in understanding T-cell subsets has led to experts to question whether a neuroprotective T-cell response is initially mounted in ALS but fails because of unknown reasons . Therefore, in ALS, neuroinflammation is solely sustained by the interactions of microglia, neurons, and macroglia.
Growth factors are a group of proteins promoting the growth and survival of cells. Important neurotrophic growth factors include neural growth factor, brain-derived neurotrophic factor, insulin-like growth factor I, and vascular endothelial growth factor (VEGF) .
Dysregulation of several growth factors have been implicated in ALS . For example, deletions in the VEGF promoter caused ALS-like symptoms in mice , and patients who have ALS show reduced VEGF levels in cerebrospinal fluid. In the CNS, astrocytes and microglia—the main mediators of neuroinflammation—are also the main sources of neurotrophic factors . Neuroinflammation leads not only to an increase in inflammatory products but also is usually accompanied by the down-regulation of neurotrophic factors . This withdrawal of trophic support could add insult to injury and finally drive damaged motor neurons into apoptosis .
The increased expression of inflammatory factors or down-regulation of growth factors could also explain how damage to lower motor neurons (spinal cord) could propagate to upper motor neurons (cortex). Proapoptotic inflammatory signals (eg, TNF-α, FasL) in the spinal cord could be detected by the axonal projections of upper motor neurons and trigger apoptosis in these cells. Likewise, the down-regulation of VEGF, for example, in the target area of an upper motor neuron could lead to apoptosis of this cell from failure of trophic support ( Fig. 1 ) .
The (mis)conception of cell autonomous effects
Motor neuron cell loss was long seen from a neurocentric viewpoint as a “disease from within the motor neuron.” Recently, it became clear that cell–cell interactions must be considered part of the cascade leading to cell death. Therefore, motor neuron degeneration occurring from intrinsic dysfunction and was called cell autonomous , even though motor neuron–autonomous seems to be the more correct term. When surrounding cells contribute to the event leading to motor neuron loss, the effect would be called non–cell autonomous . Clearly, neuroinflammation would be a non–cell autonomous effect from the motor neuron viewpoint.
Compelling evidence from the mtSOD1 transgenic animal models of ALS showed the involvement of non–cell autonomous effects in the pathogenesis of motor neuron disease. In wild-type/mtSOD1 chimeric mice, wild-type motor neurons surrounded by mtSOD1 glia were damaged, whereas mtSOD1 neurons surrounded by wild-type glia were healthier . This finding strongly indicates a non–cell autonomous disease mechanism and the critical involvement of cell types other than neurons.
Very recently, using an elegant approach, mtSOD1 was selectively removed from microglial cells and macrophages through a CD11b-driven Cre/LoxP system. These animals showed a dramatically slowed disease progression. Removing mtSOD1 from motor neurons, however, delayed the onset of disease but had little influence on disease progression . Although some open questions remain about these data pertaining to limited recombination efficiency, the results clearly indicate a role for mtSOD1 within microglial cells in the disease process.
Similar data were obtained through crossing the mtSOD1 into the PU.1 knockout strain, which are unable to develop myeloid and lymphoid cells without bone marrow transplantation. Disease in mtSOD1/PU.1 −/− animals receiving wild-type bone marrow progressed considerably slower compared with mtSOD1 bone marrow controls . This article and an earlier report showed that the expression of mtSOD1 in microglial cells leads to an increase in their cytotoxic potential, because they release increased amounts of superoxide, nitrate, and TNF-α in vitro .
Clearly, microglial intracellular mtSOD1 leads to unidentified changes in microglial phenotype with relevance to disease progression. In contrast, the activation of microglial cells by extracellular mtSOD1 was recently shown . The authors showed that chaperone-associated mtSOD1 secreted from neuronal cells triggered microglial activation and in turn led to motor neuron death in vitro. Taken together, both pathways, intracellular and extracellular mtSOD1, could trigger a vicious neuroinflammatory circle with microglia at center stage.
Astrocytes have recently reappeared on the center stage of ALS . They have been implicated early on as key players in the glutamate toxicity hypothesis because their transport capacity seems hampered in ALS . However, recent data showed that mtSOD1 within astrocytes changes their properties and that they secrete an unknown factor leading to motor neuron degeneration .
Using their mtSOD1 CreLloxP system, the Cleveland group used a GFAP-Cre mouse to excise mtSOD1 from astrocytes. In contrast to the CD11b/microglial Cre model, the recombination efficiency was very high. Although genetic ablation of mtSOD1 in astrocytes did not affect the onset of disease, it significantly slowed its progression. The removal of mtSOD1 from astrocytes reduced the activation of microglial cells, as assessed using MAC-2 and iNOS staining. These indirect effects on microglia also show the close relationship of the two arms of the CNS’ immune system.
Non–cell autonomous has been used to define the involvement of other cells contributing to motor neuron death. Both microglia and astrocytes seem to play crucial roles in the motor neuron’s demise in ALS, although this may imply that mtSOD1 has a cell autonomous effect on glial cells. How microglia or astrocytes actually transmit these effects to motor neurons and induce their loss remains to be shown. The fact cannot be excluded that the mtSOD1 protein accumulation in microglia or astrocytes could also induce intrinsic loss of properties, such as a diminished neuroprotective function, which may be one mechanism contributing to the loss of motor neurons. Nevertheless, the inflammatory cascade doubtlessly plays a important role in ALS, lending credibility to the idea that therapeutic modulation of inflammation may help treat this currently incurable disease ( Table 1 ).
Pharmaceutical class | Drug name | Animal model trials | Clinical trials (n of patients enrolled) | Comments | References |
---|---|---|---|---|---|
Antioxidant | Coenzyme Q(10) (ubiquinone) | SOD G93A a | No effect on survival and possible gender effect on neurologic scores, which were improved for men only; effect on weight loss | ALS Therapy Development Institute (TDI) | |
Anti-inflammatory molecules | Prednisolone | SOD G93A | No effect on survival and onset of symptoms; possible weight loss from treatment | ALS TDI | |
Immunosuppressive treatment | No effect | Werdelin et al, | |||
Minocycline | SOD G93A | Delayed disease onset and prolonged survival in a dose-dependant manner; contradictory results; slight or no effect of minocycline | Kriz et al, Van Den Bosch et al, ALS TDI Diguet et al, Zhang et al, | ||
Additive neuroprotective effect when coupled with creatine | |||||
Multicenter, randomized, placebo-controlled phase III (412) | Harmful side effect on patients who have ALS | Gordon et al, | |||
Thalidomide | SOD G93A | Various doses tested, gender differences, and slight increase of survival | ALS TDI Kiaei et al, | ||
Reduce production of proinflammatory molecules (TNF) | |||||
| Cotreatment with riluzole | Charite University Hospital (Thomas Meyer), Dartmouth Hitchcock Medical Center | |||
Celebrex/ celecoxib | SOD G93A | Cox-2 inhibitor treatment delayed onset of symptoms and prolonged survival | Drachman et al, | ||
Celebrex treatment results are inconclusive but not confirmatory; problem in validity of doses used | ALS TDI | ||||
Double-blind, placebo-controlled study (300) | No biologic effect in patients | Cudkowicz et al, | |||
Growth factors | CNTF | Phase II/III randomized, placebo-controlled, double-blind study (730) | Adverse side effects of rHCNTF (weight loss, anorexia, and cough) resulting from withdrawal of treatment; no beneficial effect? | ALS CNTF Treatment Study Group | |
Phase I study ex vivo gene therapy | Continuous delivery presented no limiting side effects | Aebischer et al, | |||
GDNF | SOD G93A (rat) | Prevent motor neuron loss but fail to maintain functional neuromuscular function | Suzuki et al, | ||
SOD G93A | Retrograde transport (GDNF in muscle) slowed down disease progression whereas GDNF overexpressed in astrocytes had no effect | Li et al, | |||
VEGF | SOD G93A | Prolonged survival and delayed onset | Azzouz et al, | ||
Reduced astrogliosis and increased number of neuromuscular junctions | Zheng et al, | ||||
SOD G93A (rat) | Delayed onset and prolonged survival; particularly effective for most severe forms of ALS | Storkebaum et al, | |||
Immunosuppressors | Cyclosporin A | SOD G93A | 12% extension survival; difference of effect between gender | Karlsson et al, Kirkinezos et al, | |
Double-blind study (74) | Beneficial only in men on early onset | Appel et al, | |||
FK 506 | SOD G93A | No beneficial effect on survival and symptoms | Anneser et al, | ||
HDAC inhibitors | Sodium phenyl butyrate | SOD G93A | Extends survival | Ryu et al, | |
Sodium valproate | SOD G93A | Increases survival and delay onset | ALS TDI | ||
Double-blind, randomized study (173, ongoing) | UMC Utrecht | ||||
Trichostatin A | SOD G93A | No effect on survival and symptoms | ALS TDI |
Current therapy in amyotrophic lateral sclerosis: neuroprotective, anti-inflammatory, or both?
Although a sizable number of medications have been tested in ALS, there is currently only one FDA-approved drug, riluzole, available for therapy . Riluzole, believed to exert its effect through presynaptic reduction of glutamate release, prolongs survival by a mere 3 months and does not clearly improve quality of life or functional parameters in patients who have ALS .
Although most studies indicate that riluzole works on neurons, some argue that microglia could be a target of the drug. Microglia can release high levels of glutamate, creating the possibility that riluzole could also slow down disease progression through an anti-inflammatory effect on microglia .
The advent of ALS animal models has prompted a surge in preclinical studies. The predictability of disease onset and progression makes these animals useful for pharmacologic trials . Although some concern has been expressed about the inherent limitations of mtSOD1 models (eg, they resemble only a small population of patients who have ALS, several pharmacologic results from these models do not hold true in humans ), these animals are the only models currently available and have already greatly advanced understanding of ALS .
The current list of compounds in preclinical and clinical studies is long and includes targets for all suspected causes (eg, glutamate toxicity, oxidative stress) . Most relevant for this article, however, are the group of drugs that are believed to exert their effects through an anti-inflammatory mode of action.
Celecoxib
Cyclooxygenase-2 (COX-2) is an enzyme central to the production of prostaglandins (PG), a family of powerful lipid mediators of inflammation . COX-2 has been shown to be involved in many inflammatory diseases, including rheumatoid arthritis, inflammatory bowel disease, and multiple sclerosis . In the CNS, COX-2 is constitutively expressed in a subset of neurons in vitro and in vivo , but can be readily induced through injury in most neurons and astrocytes .
Activated microglia also express COX-2 and produce considerable amounts of PGE2, which has been shown to be detrimental to neuronal survival . Both COX-2 and PGE2 are elevated in the CNS of patients who have ALS .
Celecoxib is a COX-2 inhibitor that was introduced as an improved therapy for rheumatoid arthritis. It is currently being pursued as a therapeutic approach in neurodegenerative disease . In mtSOD1 mice, celecoxib prolonged survival and reduced microglial activation as assessed through immunohistochemistry . Unfortunately, clinical studies in humans have not shown efficacy, and celecoxib, although safe and well tolerated, did not improve muscle strength nor reduce motor neuron loss during 12 months of treatment .
Minocycline
Minocycline, known as a semisynthetic second-generation tetracycline antibiotic, is also an inhibitor of microglial activation . It has been shown to be neuroprotective in models of ischemia, Parkinson’s disease, and spinal cord injury. Its function is most likely through inhibition of microglial activation . In mtSOD1 mice, minocycline significantly slowed disease onset and progression and reduced microglial activation . Based on these data, phase I/II trials are currently underway for the use of minocycline in ALS . Despite these encouraging results, the molecular mechanisms underlying these effects are still unclear. Proposed actions of minocycline are p38 mitogen-activated protein kinase inhibition and inhibition of mitochondrial cytochrome c-release .
Histone deacetylases inhibitors
Transcriptional dysregulation has recently been recognized as a important factor in neurodegeneration and there is good indication that it also plays an important role in ALS . Inhibitors of histone deacetylases (HDAC), the central regulators of transcriptional activity, have received increasing attention as potential tools to interfere with transcriptional dysregulation . HDAC inhibitors were first developed as antitumoral agents, but they also seem to have strong anti-inflammatory properties .
Treatment with different HDAC inhibitors improved the survival and delayed motor neuron impairment in mtSOD1 mice . A phase II clinical trial involving 40 patients who have ALS is currently underway to establish human safety for one type of HDAC inhibitor . Suberoylanilide hydroxamic acid, a third-generation HDAC inhibitor, was shown to suppress cytokine expression in macrophage and mononuclear cells , supporting the hypothesis that HDAC inhibitors might exert their effects on macrophage/microglia activation.
Thalidomide
Thalidomide, originally introduced as a sedative and withdrawn from the market because of its devastating teratogenic effects, has recently received renewed interest as an anti-inflammatory drug . Thalidomide inhibits the expression of cytokines such as TNF-α, which are linked to neuroinflammation in many neurodegenerative diseases, including ALS. In the mouse ALS model, thalidomide significantly increased survival ; however, the molecular mechanisms of this effect remain unknown.
Taken together, these reports stress the attractiveness of anti-inflammatory therapy as a therapeutic option in ALS . Different classes of drugs, such as minocycline, celecoxib, thalidomide, and HDAC inhibitors may act through a shared anti-inflammatory mechanism. To provide a more tailored (and hopefully more successful) anti-inflammatory therapy, the shared and specific effects of each drug must be understood in the context of ALS-associated neuroinflammation. Once better understood, targeted interference with neuroinflammatory processes could be a tool to develop new therapeutic approaches.
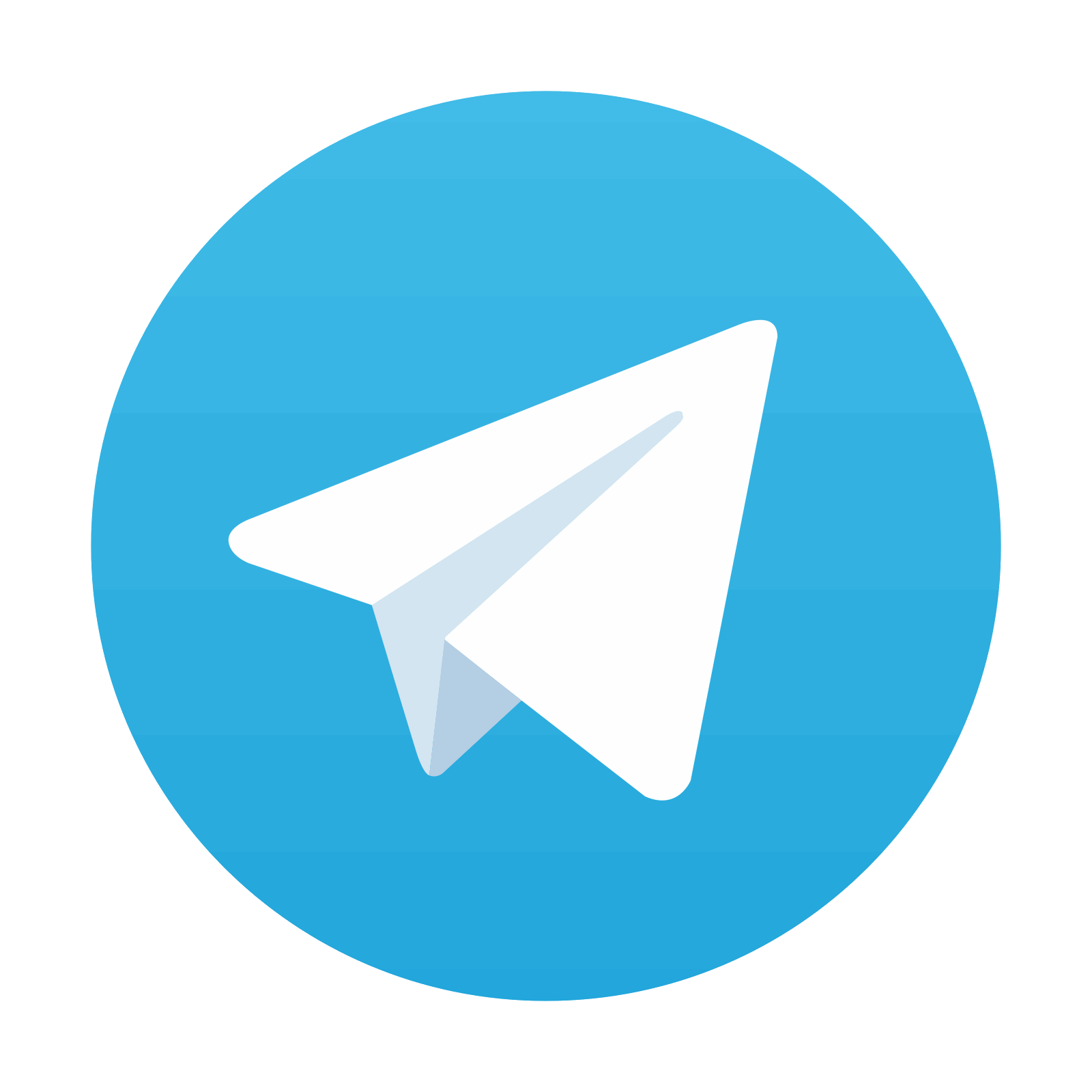
Stay updated, free articles. Join our Telegram channel

Full access? Get Clinical Tree
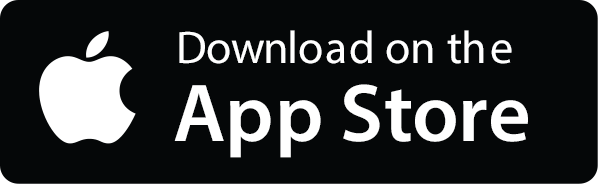
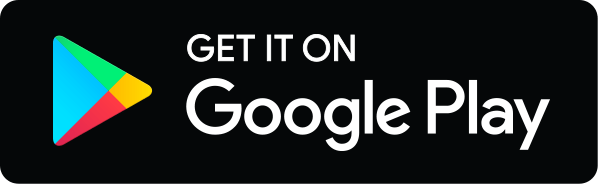