The world is facing an unprecedented demographic shift in the human population. The number of people aged 65 or older is projected to increase from 524 million in 2010 to nearly 1.5 billion by 2050.1,2 With this demographic shift, a corresponding increase in the prevalence of mobility limitations and other disabilities are also expected. The prevention and postponement of mobility limitations for the aging population is of major public health importance and the role of rehabilitation specialists will be tremendously emphasized.
Older adults are diverse in their functional level ranging from senior Olympians to those who are dependent in mobility and activities of daily living (ADL). The need of older individuals to stay active and prevent functional decline also varies based on the individual’s functional level. However, there are universal physiologic changes which occur with aging. In addition, reduced reserve in their organ systems may predispose older adults more vulnerable to injuries and hinder their healing regardless of their functional level. In this chapter, we will discuss the key information for rehabilitation specialists to provide optimal care for the geriatric population, including physiologic changes in organ systems with aging, their relevance in common conditions including falls and mobility limitations, and specific considerations during interventions for the geriatric population.
Distinguishing what changes are expected with age from those attributed to disease is essential in the assessment of the older adult. In general, most physiologic functions decline at a rate of 1% per year beginning at age 30.3 Age-related physiologic changes and their clinical significance are summarized in Table 83–1.4–6 Changes in the musculoskeletal system have particular relevance to mobility decline and are elaborated further.
Organ/System | Age-Related Changes |
Musculoskeletal System | 1.5%–2% decline in muscle strength/year after age 40. Preferential loss of type II fibers. Decline in muscle power is more pronounced than muscle strength. (see more details in the text) |
Neurological System | Depletion of neurons in brain, and loss of anterior horn cells. Decreased nerve conduction velocities. |
Cardiovascular system | Cardiac output (CO) decreases after the 3rd decade at a rate of 1%/year. Causes for ↓ in CO: ↓ in inotropic response to catecholamines, ↑in myocardial stiffness, and progressive stiffening of the aorta (↑afterload). Decrease in aerobic capacity (↓VO2max). |
Respiratory system | Linear ↓ in vital capacity: Men (26 mL/year), Women (22 mL/year) after age 20. Residual volume (RV) ↑ with age. Total lung capacity (TLC) remains constant. RV/TLC ↑ from 20% at age 20 to 35% at age 60. ↑ in alveolar-arterial oxygen difference (A-a)O2 due to mismatch of ventilation and perfusion. Predisposition to pneumonia due to depression of immune system function, aspiration of oropharyngeal secretions, slower mechanical clearing of tracheobronchial tree by mucociliary apparatus and less effective cough. |
Kidney/Urinary System | ↓ in number of glomeruli (1,000,000 at age 30 to 700,000 by age 65). ↓ in creatinine clearance. ↓ in glomerular filtration rate (GFR): 10%/10 years after age 30. No change in serum creatinine concentration with age due to age-related ↓ in creatinine production (↓ in muscle mass). Drugs cleared by glomerular filtration (e.g., aminoglycosides, digoxin, penicillin, tetracycline) may have prolonged half-life even the dosage is adjusted based on serum creatinine concentration. ↓ in ability to concentrate and dilute urine: ↑ vulnerability for dehydration and hyponatremia. Urinary incontinence (17% of men, 23% of women ≥ age 65), and overflow incontinence due to possibly reduced and delayed sensation of urge to urinate. |
Gastrointestinal System | Presbyesophagus- ↓ in peristaltic response, ↑ in nonperistaltic response, a delayed transit time. ↓ in relaxation of the lower sphincter during swallowing—may lead to dysphagia and reduced calorie consumption. Atrophic gastritis (40% of those ≥ age 65): risk of achlorhydria, deficient intrinsic factor secretion, ↓ pepsinogen production. Hypotonic colon: longer stool transit time, dehydration, which eventually causes chronic constipation. Loss of control in internal and external anal sphincters. Prevalent diverticulosis (50% in those ≥ age 80). Liver function shows little change with aging, yet drugs (e.g., diazepam) are metabolized more slowly with aging. |
Endocrine System | ↓ in number and function of insulin-producing beta cells. More inactive precursor proinsulin released in response to a glucose challenge and development of progressive peripheral insulin resistance with age. ↓ in lean body mass with relative ↑in adiposity (↑ in fat cell size, not in number of fat cells) |
Sexual Function | Men produce less sperm and sexual responses are delayed by 50 years of age. Impotence in male is NOT a normal part of aging. Women after menopause have ↓ in size of uterus and ovaries and ↓ in vaginal secretion. |
Skin | The turnover rate of cells in the stratum corneum ↓ with age (e.g., 50% longer to reepithelialize blistered skin at age 65 compared to the young). ↓ in epidermal cell growth and division and ↓in number of dermal blood vessels contribute to risk of pressure ulcers. Less activity of sweat glands, hydration is difficult to assess by skin alone. |
Hearing | Presbycusis—high-frequency sounds become harder to hear along with difficulty in distinguishing the changes in tone and speech due to loss of hair cells and fibrous changes in the cochlea. Difficulty having a conversation in large crowded rooms with background noise. Conductive hearing loss from middle or outer ear canal (e.g., cerumen accumulation in external canal). |
Eyes/Vision | Dry eyes from ↓ tear production. Three common disease conditions (cataract, glaucoma, macular degeneration-central vision loss). |
The cross-sectional area of muscle, which is the major determinant of muscle strength, declines at a rate of 1% to 1.4% per year after age 50, due to a reduction in muscle fiber size and numbers.7 The annual decline in muscle strength is greater than that of muscle mass; 1.4% to 2.5% depending on muscle group and angular velocity of the movement.7 The decline in isokinetic strength in muscles of the lower limb is larger than those of the upper limb. In spite of decline in muscle mass, the overall body weight may not change due to relative increase in fat mass (sarcopenic obesity).8 In part, these changes are due to neuronal death and decreased collateral innervation seen with increased age (see Fig. 83–1).
Figure 83–1
Overview of the age-related changes that may occur in the motor innervation of older adults’ skeletal muscle fibers. (Reproduced with permission from Manini TM, Gundermann DM, Clark BC. Aging of the Muscles and Joints. In: Halter JB, Ouslander JG, Studenski S, High KP, Asthana S, Supiano MA, Ritchie C, eds. Hazzard’s Geriatric Medicine and Gerontology, 7e New York, NY: McGraw-Hill; 2017.)

The ability of skeletal muscle to generate force and movement may be compromised by a compilation of decrease in cortical and spinal excitability, incomplete activation of motor units (central motor drive), peripheral nerve dysfunction, reduced cellular mechanism and energy supply, loss of hormonal influences, changes in the excitation-contraction coupling mechanisms, or by alterations in the contractile elements of muscle cells9 (see Fig. 83–2).
Figure 83–2
Potential sites and physiological mechanisms that regulate strength. The neuromuscular system contains several potential sites that can affect voluntary force or power production, such as excitatory drive from supraspinal centers, a-motor neuron excitability, antagonistic muscle activity, motor unit recruitment and rate coding, neuromuscular transmission, muscle mass, excitation-contraction (EC) coupling processes, and muscle morphology and architecture. There is evidence of aging-induced alterations at nearly every denoted site within the system such as, but not limited to, decreased cortical excitability, decreased spinal excitability, decreased maximal motor unit discharge rate, slowed nerve conduction, alterations in muscle architecture, decreased muscle mass, increased myocellular lipid content, and excitation-contraction uncoupling. (Reprinted with permission from Manini TM, Clark BC. Dynapenia and aging: an update. J Gerontol A Biol Sci Med Sci. 2012;67(1):28–40)

The intrinsic ability of individual muscle fibers to generate force is reduced in both fiber types among older adults compared to the young. However, type II muscle fibers may be reduced disproportionally compared to type I fibers in both the upper and lower limb muscles10 (Fig. 83–3).
Sarcopenia is a syndrome characterized by progressive and generalized loss of skeletal muscle mass and strength with a risk of adverse outcomes such as physical disabilities, poor quality of life, and death.8 Sarcopenia can be primary related to aging or secondary when one or more causes (e.g., bed rest, organ failure, malignancy, inadequate nutrition) are present.8 For the diagnosis of sarcopenia, the European Working Group on Sarcopenia in Older People (EWGSOP) recommends using the presence of both low muscle mass and low muscle function (strength or physical performance).8 To measure muscle mass, dual energy x-ray absorptiometry (DEXA) is clinically recommended over computer tomography or magnetic resonance imaging due to low cost and radiation risk.11 Skeletal muscle mass index (SMI, Appendicular skeletal muscle mass/height2) is the parameter used to determine muscle mass.8,11 For muscle strength measurement, handgrip or knee flexion/extension strength is recommended.8 Physical performance is evaluated by short physical performance battery or usual gait speed.8,11 The cutoff value of muscle mass (SMI) for the diagnosis of sarcopenia is SMI below 2 standard deviation (SD) from the mean value of SMI in young adults.8,11 The cutoff for the handgrip strength is 20 kg for women and 30 kg for men and the values lower than these are considered low strength.8,11 Gait speed slower than 0.8 m/s is considered as reduced physical performance.8,11
The prevalence of sarcopenia varies widely depending on the population and diagnostic criteria: 1% to 29% among community-dwelling older adults and 14% to 33% among the long-term care population.12 Sarcopenia is present in other syndromes including cachexia and frailty. Cachexia is a complex metabolic syndrome associated with underlying illness and characterized by muscle loss.8,13 Most cachectic individuals have sarcopenia; however, most sarcopenic individuals may not have cachexia. Frailty is a geriatric syndrome resulting from age-related cumulative declines across multiple systems with a reduced capacity of the individual to withstand stress, thus increasing vulnerability to adverse health outcomes including falls, hospitalization, and death.8
The definition of frailty is based on the phenotypic features: presence of ≥3 of the following-unintended weight loss, exhaustion, weakness, slow gait speed, and low physical activity.14 The concept of frailty is larger than the physical domain encompassing psychological and social domains, although frail older individuals usually have sarcopenia.14,15
More recently, muscle power (the product of the force and velocity of muscle contraction) has been recognized as a more discriminant predictor of functional performance in older adults compared to muscle strength (the ability to generate maximal muscle force).16,17 Older adults with low muscle power output had a twofold to threefold greater risk of significant mobility impairment compared to individuals with low muscle strength.17 Muscle power has been shown to decline earlier and more rapidly than muscle strength with aging.18 The hypothesized mechanisms for muscle power decline include preferential loss of type II fibers which can generate higher power, impairment in neuromuscular activation, fat infiltration into muscle, alteration in hormonal status, and inflammatory mediators.19
Bone is a composite structure consisting of extracellular organic matrix (collagen and non-collagen proteins) mineralized with calcium phosphate and hydroxyapatite, cells (osteoblasts, osteoclasts, stromal cells), lipids, and water. Bone turnover via resorption and formation is continuous throughout life with 5% to 10% of the total adult skeleton being replaced every year.20 Typically, individuals reach their peak bone mass by the age of 15 to 25, which is then usually maintained for approximately 10 years. By the early 30s, total bone mass starts to decrease at a rate of 1% per year.21 Women experience steeper loss in bone mass shortly after menopause. The clinical implications of bone loss with aging is described in detail under osteoporosis later in the chapter.
Clinicians should not automatically consider gait changes in older adults as an inevitable consequence of aging. Gait velocity and stride length of older adults are reduced by 10% to 20% in their 70s compared to their 20s.22 Stance width and double support time increase with aging which likely reflects as an effort of older individuals to produce stable gait pattern. Abnormal gait pattern is further described under neurological disorders later in the chapter.
There are a number of definitions for a fall coded W00-W19 in International Classification of Disease-10 (ICD-10). The World Health Organization (WHO) defines a fall as inadvertently coming to rest on the ground, floor, or other lower level, excluding intentional change in position, to rest on furniture, wall, or other objects.1,23
Falls occur in 30% to 60% of older adults every year and 10% to 20% of these results in injury, hospitalization, and/or death.1,24 One in three older adults living in the community fall annually and a higher percentage experience fall and injuries in long-term care settings.24 Direct injuries associated with falls are fractures, head/spinal cord injuries, and lacerations. Secondary complications of falls are hypothermia, pneumonia pressure ulcers, rhabdomyolysis, venous thromboembolism, and dehydration. In addition to physical injury, falls can predispose older adults to develop a fear of falling which can create a downward spiral of restriction in physical activities, deconditioning, depression, and social isolation.25
A fall is often a result of interactions between environmental hazards and the person’s accumulated age-related changes in functioning and disease processes. Prevention strategies address both sides of these interactions: reducing the environmental hazards and managing age-related impairments and diseases. Intrinsic fall risk factors include older age, prior fall, disability of lower limb (Odds ratio; OR 3.8), balance and gait abnormality (OR 1.9), foot problems (OR 1.8), vision impairment, postural hypotension, impaired cognition (OR 5.0), depression, and urinary incontinence.24,26 Extrinsic fall risk factor include environmental hazards, sedative use (OR 28.3), and polypharmacy. The risk of fall increases linearly with the higher the number of risk factors: 8% with no risk factors to 78% with ≥ 4 risk factors26 (see Fig. 83–4).
The Centers for Disease Control and Prevention (CDC) offers a practical guide, Stop Elderly Accidents, Deaths, and Injuries (STEADI)—Older Adult Fall Prevention, to identify older individuals at risk of falls, modifiable risk factors, and offer effective interventions.27 In brief, all older adults are asked key screening questions: fell in past year, feels unsteady when standing or walking, or worries about falling. If they answer affirmatively, gait, strength, and balance is further evaluated (e.g., Timed Up and Go [TUG] test). An older adult who takes ≥ 12 seconds to complete the TUG is at high risk of falling.27 Physical Examination should include (1) gait, balance, mobility level, and lower extremity joint function, (2) neurological examination including muscle strength, (3) cardiovascular status (e.g., postural blood pressure), (4) visual acuity, and (5) examination of feet and footwear. Regardless of fall risk, at least 800 International Units (IU) of vitamin D per day +/− calcium, strength and balance exercise, and overall education about falls are recommended.27 Additional measures addressing hypotension, medication reconciliation, foot problems, optimizing vision, home safety, and referral to rehabilitation are recommended for patients with higher risk of falls. Multifactorial interventions are summarized in Table 83–2 based on the American Geriatrics Society/British Geriatrics Society Clinical Practice Guideline for Prevention of Falls in Older Persons and STEADI from the CDC.27,28
Risk Factor | Interventions |
Polypharmacy Medications increasing risk of falls: Psychotropics, Serotonin reuptake inhibitors (SSRIs), Tricyclic antidepressants (TCA). *SSRIs increase fall risk as much as TCA.28 | Stop or reduce
|
Weakness, Balance, and/or Gait Impairment |
|
Postural hypotension (↓ in systolic blood pressure ≥ 20 mm Hg or ↓ in diastolic blood pressure ≥ 10 mm Hg or feeling lightheadedness upon upright position from supine) |
|
Vision impairment (e.g., cataract) |
|
Vitamin D supplement |
|
Foot problems Footwear that fits poorly, has worn soles, high heels, not laced or buckled when worn |
|
Environmental hazards |
|
Lack of education about fall prevention |
|
Osteoporosis is a skeletal disease characterized by low bone mass and microarchitectural deterioration of bone tissue with a consequent increase in bone fragility and susceptibility to fracture. Type I osteoporosis is seen in women and is thought to be estrogen-dependent accelerated bone loss after menopause. In this situation, resorption exceeds the rate of bone formation possibly due to increased cytokine production and subsequent promotion of osteoclastic activities in the estrogen-deficient state. Type II osteoporosis occurs with aging, affecting both genders. The mechanism is due to a decrease in number and activity of osteoblasts which leads to decreased bone formation. The degree of osteoporosis is defined by bone mineral density (see Table 83–3).
Category | Definition by Bone Density |
Normal | BMD is within 1 standard deviation (SD) of a young normal adult (T score is greater than −1.0) |
Osteopenia | BMD is between 1 and 2.5 SD below a young normal adult (T score is −1 to −2.5) |
Osteoporosis | BMD is 2.5 SD or more below a young normal adult (T score is less than −2.5) |
Severe or established osteoporosis | BMD is 2.5 SD or more below a young adult with 1 or more fragility fractures |
Worldwide, 9 million fractures occur annually, secondary to osteoporosis.29 One in three women and one in five men over age 50 will experience osteoporotic fractures.30–32 As rates of osteoporosis in the United States increase,33 the risk of osteoporotic fracture is also projected to increase. For example, by 2050, the incidence of hip fracture is estimated to increase by 310% in men and 240% in women.34 The incidence of fragility fractures by skeletal sites is: vertebral (27%), wrist (19%), hip (14%), pelvis (7%), and other (33%) with the highest cost associated with hip fractures in the United States (72% of total care cost; Fig. 83–5).33
Each year, over 300,000 people of age 65 and older are hospitalized for hip fractures.35 Lifetime risk of hip fracture is 17.5% in Caucasian women and 6% in Caucasian men above age 50.32 The vast majority (> 95%) of hip fractures are due to falls. Hip fractures typically occur from falling sideways, as opposed to falling backward onto the buttock, which has much lower rates of fractures.24,36 Hip fractures increase disproportionately when compared to wrist fractures after age 75, reflecting loss of the ability to protect the hip by bracing the fall with one’s wrist.24 Women experience 75% of all hip fractures due to more frequent falls and greater prevalence of osteoporosis than men.35 Women can lose as much as 35% of their cortical bone and 50% of their trabecular bone 30 years after menopause.37 Long bones typically respond to loss of bone mass by increasing their diameter, but the thickness of the cortex itself decreases.38 In addition to increasing age (≥ 75), female gender, and osteoporosis, other risk factors for hip fractures include muscle weakness, foot deformities, sensory impairment, polypharmacy, impaired cognition, depression, use of alcohol and benzodiazepines, orthostatic hypotension, and environmental hazards (e.g., loose cords; see Table 83–4).
Osteoporosis/Fracture | Falls |
Women > 65 years of age | Advanced age |
Men > 70 years of age | Dementia |
White or Asian race | Previous falls |
Low body weight (< 127 lb or body mass index < 20) | Low body weight |
Family history of osteoporosis | Low muscle strength |
Personal history of fragility fracture | Poor nutrition |
Fragility fracture in first-degree relative | Polypharmacy |
Long-term use of glucocorticoids | Use of long-acting benzodiazepines |
Alcohol > 2–3 drinks per day | Poor vision |
Estrogen deficiency < 45 years | Self-rated poor health |
Testosterone deficiency | Difficulty in rising out of chair |
Low calcium intake | Resting tachycardia |
Vitamin D deficiency | Vitamin D deficiency |
Sedentary lifestyle | Sedentary lifestyle |
Current tobacco use |
FRAX algorithm is used to predict the 10-year probability of fracture using information; country, bone mineral density, age, gender, and clinical risk factors—personal history of fracture; low body mass index; use of oral glucocorticoid therapy; rheumatoid arthritis, osteoporosis secondary to another condition; parental history of hip fracture; current smoking; and alcohol intake of three or more drinks per day.
The presenting symptom of hip fracture is usually groin or upper thigh pain. Patients may or may not be able to walk depending on the severity of injury.37 For displaced fractures, the leg will be externally rotated and shortened. Differential diagnosis includes pelvis fracture, spine injury, spinal stenosis, trochanteric bursitis, muscle tears, and knee injuries. Plain radiograph of the anteriorposterior (AP) pelvis allows side-to-side comparison and preoperative planning. An AP view of the affected hip with leg in internal rotation should be obtained to best visualize the femoral neck for possible fracture lines (see Fig. 83–6). A cross-table lateral projection of the hip is useful for visualizing posterior comminution of the fracture.37,39 For patients with high index of suspicious, yet with inconclusive, plain radiographs, a magnetic resonance imaging (MRI) scan is recommended for its high sensitivity to detect fractures.37
There are three broad categories of hip fractures based on the location of the fracture: femoral neck (intraarticular, most common, approximately 45%–50% of hip fractures), intertrochanteric, and subtrochanteric fractures39 (see Fig. 83–7).
Femoral neck fractures are intraarticular fractures associated with high risk of nonunion and avascular necrosis of the femoral head. Nondisplaced stable fractures can be treated with pinning. Hemiarthroplasty or total arthroplasty is offered for displaced femoral neck fractures in older adults. There are three operative approaches to hip arthroplasty: posterolateral, anterolateral, and anterior approach. The posterolateral approach has an advantage of no abductor damage but the disadvantage is a higher risk of dislocation. Anterolateral and anterior approach has a low risk of dislocation; however, there is a possibility of abductor damage (anterolateral approach) and is technically more demanding (anterior approach).39 Subtrochanteric fractures are located in the proximal part of the femoral shaft below the lesser trochanter and are less common than femoral neck or intertrochanteric fractures. Subtrochanteric fractures are typically treated with an intramedullary hip screw.
Patients are discharged to a variety of settings for their recovery after hip fracture. Although the cost of rehabilitation at an inpatient rehabilitation facility (IRF) is more costly than that of a skilled nursing facility (SNF),40,41 rehabilitation at an IRF setting may be cost saving in the longer term due to lower readmission rates and superior functional outcomes.42,43 The readmission rate was the lowest when patients were cared for at the IRF compared to home or SNF after hip replacement or hip fracture.42,44
Those who underwent arthroplasty are instructed on hip precautions, which are movement restrictions to prevent hip dislocation and are based on the approach of surgery. No hip flexion over 90 degrees, no internal rotation, or adduction crossing midline is the precaution for posterolateral approach. An abduction pillow is often used to maintain hip precautions during rehabilitation. For the anterior approach, no precaution protocol of postoperative rehabilitation did not increase dislocation, although extension and external rotation may be recommended by some surgeons. Primary complications are nonunion and osteonecrosis after femoral neck fracture, prosthetic loosening, hip dislocation, infection, and fracture (see Table 83–5).
Anterior Approach Dislocation Precautions | Posterior Approach Dislocation Precautions | Global Dislocation Precautions |
|
|
|
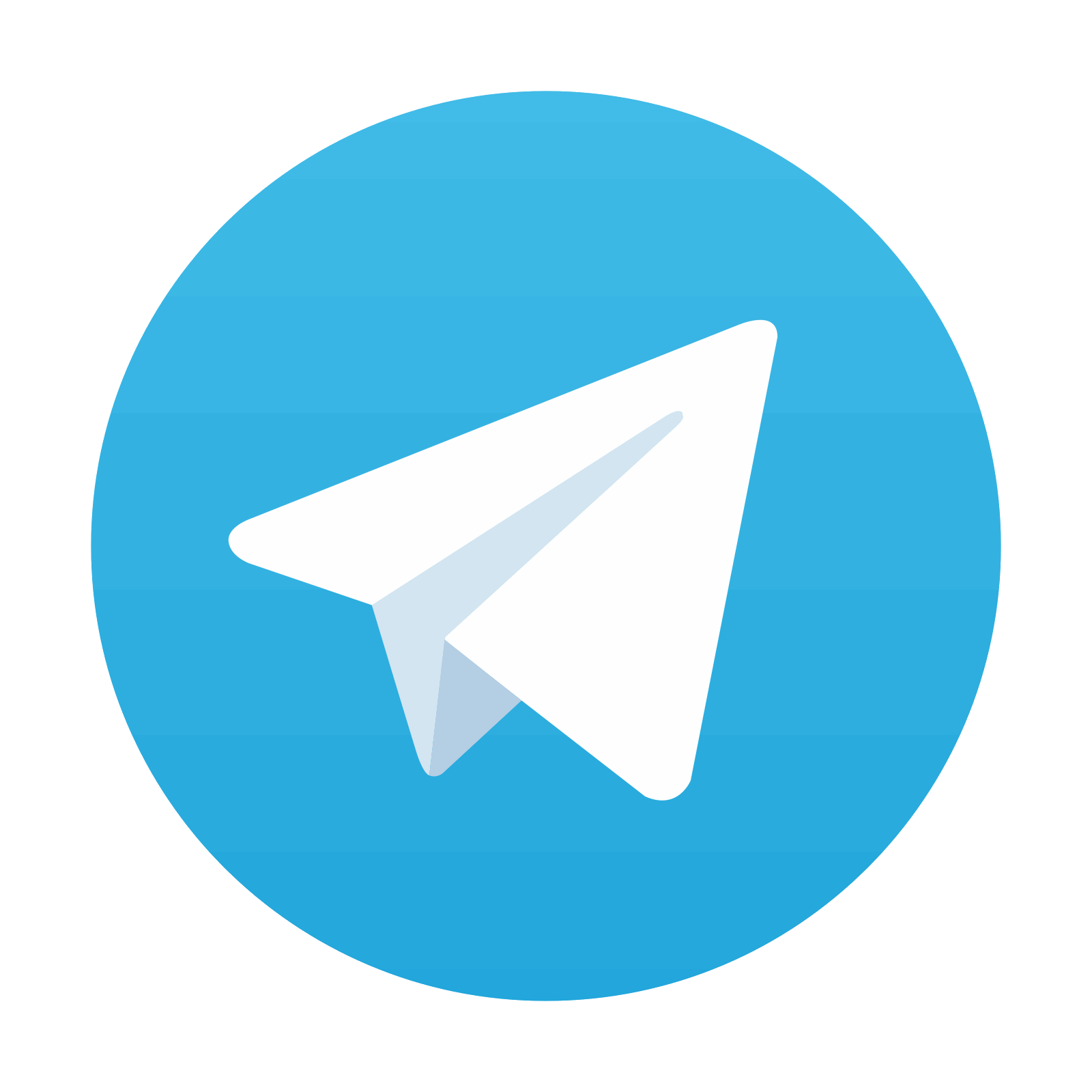
Stay updated, free articles. Join our Telegram channel

Full access? Get Clinical Tree
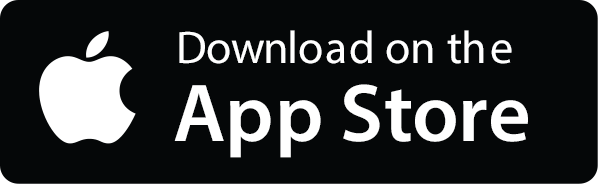
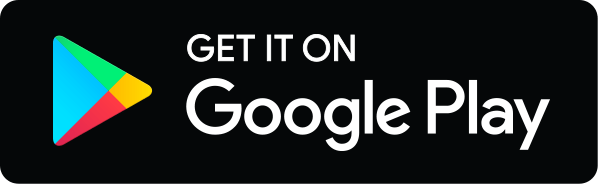
