Lumbar disk degeneration (LDD) is a common musculoskeletal condition. Genetic risk factors have been suggested to play a major role in its cause. This article reviews the main research strategies that have been used to study the genetics of LDD, and the genes that thus far have been identified to influence susceptibility to LDD. With the rapid progress in genomic technologies, further advances in the genetics of LDD are expected in the next few years.
Low-back pain (LBP) is a common musculoskeletal disorder and a global burden. Approximately 70% to 80% of people have experienced LBP at some point in their life. The annual prevalence of LBP ranges from 15% to 45%, but is largely dependent on the population being studied and surveillance methods. Low-back pain can lead to diminished function, loss of productivity, loss of work, psychological distress, and increased health care costs. As such, LBP poses considerable financial burden to nations through the direct cost of LBP treatments and the indirect cost due to loss of working productivity. In the United States, one of the most frequent reasons for medical consultation is LBP. Therefore, the impact of LBP on society is substantial, and understanding its development is imperative (see article by Karppinen and colleagues elsewhere in this issue).
Although LBP is a prevailing disorder, the factors that contribute to LBP are still not fully understood. A variety of factors, such as occupation, biopsychological factors, cardiovascular disorders, smoking, and obesity, have been suggested to be associated with LBP. Conversely, British twin studies showed that there is excessive concordance of LBP for monozygotic (MZ) twins compared with dizygotic (DZ) twins, resulting in heritabilities ranging from 52% to 68% for various lumbar disk degeneration (LDD) phenotypes. In addition, in a study by Battie and colleagues of Finnish twins, heritability estimates ranged from 30% to 39% for various definitions of back pain problems during the prior year. Apparently, genetic factors play a vital role in the cause of LBP.
Intervertebral disk (IVD) degeneration is one proxy by which genetic risk factors act to influence LBP, as revealed by studies that found IVD degeneration to be associated with LBP. Although LBP is a multifactorial condition, discogenic origin can be mediated by such mechanisms as nerve ingrowth in degenerated disks, nerve root compression from herniated disks, and inflammatory responses of surrounding tissues in response to disk pathology. Because of the close relationship between LBP and LDD, and because both LDD and LBP have a large genetic component in their cause, it is worthwhile to investigate the genetics of LDD to unveil the mechanism by which LDD, and thus LBP, develop. Thus, this article discusses and raises awareness of genetic factors related to LDD. Furthermore, this article also addresses the genetic-based technologies, methodologies, and study designs that have been developed to assess LDD.
Genetic component of LDD
The first step in studying the genetics of a condition is to ask whether there is a genetic component for a disease or its symptoms. The initial step in approaching this issue is to determine whether there is familial aggregation, that is, whether a higher number of occurrences of diseased individuals exist among family members than nondiseased individuals. On that basis, various studies were performed to decipher whether familial aggregation is present in LDD. Varlotta and colleagues found that, in adolescent patients with disk herniation, a larger proportion of individuals had a positive family history of disk herniation than in control subjects. This finding was subsequently further supported by other investigators, such as Frino and colleagues and Matsui and colleagues. According to a study by Postacchini and colleagues consisting of patients with discogenic LBP, the investigators noted a large proportion of first relatives who had experienced discogenic LBP and who had undergone disk surgery. Moreover, based on a study by Simmons and colleagues, patients who had undergone spine surgery had a greater frequency of relatives who had experienced LBP and sciatica. Other reports have also noted a positive family history of LBP and disk degeneration in patients who had had spine surgery. These reports and others have indicated the presence of familial aggregation for LDD and, as such, the possibility of the influence of a genetic component on LDD.
Although studies have shown an association between familial aggregation and LDD, they only suggest a possibility of a genetic component, because it is still necessary to differentiate between familial aggregation that is caused by social-behavioral factors from that attributed to genetic factors. This differentiation can be achieved through twin studies that assess differences in disease concordance rates between MZ twins and DZ twins. If there is a strong genetic background for a disease, MZ twins should have a greater concordance rate in disease status than DZ twins. From the twin studies, heritability, defined as the proportion of a population’s phenotypic differences caused by genetic variation, can be estimated. In 1995, based on 155 identical male twins of the Finnish Twin Spine Study, Battie and colleagues found that genetic risk factors play a major role in the development of LDD as noted on magnetic resonance imaging (MRI), explaining 77% of the variability. In 1999, and based on the UK Twin Study, Sambrook and colleagues assessed LDD based on MRI of 172 MZ twins and 154 DZ twins, and noted a 74% heritability rate of LDD. The findings from these seminal studies based on Finnish and UK twins greatly substantiated the role of genetics as a causal risk factor in the development of LDD.
Although the importance of genetics can be established, there are 2 interesting points to note. First, there is no single gene that is responsible for causing LDD. Based on a study by Livshits and colleagues assessing an Arabic pedigree, the investigators reported that a family history was found to be a risk factor for the development of LDD. A simple monogenic mendelian pattern of inheritance was rejected, suggesting that there could be a more complex mode of inheritance for LDD involving multiple genes. Second, genetic factors may interact with environmental factors in the causal framework of LDD. For example, based on early reports of the Finnish Twin Study, cigarette smoking status was found to greatly increase the ability to predict LDD. Therefore, because gene-environment interaction effects may be present and often may be complex to disseminate, this presents a challenge to the identification of the disease mechanism of LDD.
Defining the phenotype of disk degeneration
Throughout the years, the integrity of the intervertebral disks has been assessed by various imaging modalities, such as plain radiographs, discography, computed tomography (CT), and MRI. Standing plain radiographs are commonly used to assess disk space height and sclerosis of the end plate as well as other spinal and alignment abnormalities; however, this imaging lacks the ability to assess the soft tissue of the disk. Discography entails injection of provocative material directly into the disk to identify the discogenic origin of the pain source. This methodology is often uncomfortable to the individual and may accelerate LDD because of the direct disk injury that may be induced by the needle puncture. CT is a method widely used for chest and abdominal imaging. However, it is less useful for examining soft tissues of the disk and is associated with excessively high levels of ionizing radiation exposure. Because the hallmark of disk degeneration entails the loss of water and proteoglycan content in the nucleus pulposus with structural changes of the disk and adjacent end plate (see article by Chan and colleagues elsewhere in this issue), this process entails various stages of degeneration that are best assessed with imaging that is sensitive to such alterations. MRI is a noninvasive method of imaging that allows direct evaluation of the soft tissues of the disk and, as such, is a desirable method, or rather a more sensitive method than the alternative imaging modalities for intervertebral disk imaging in assessing the phenotype of LDD. Throughout the years, MRI technology has further been developed to assess the integrity of the disk in a more sensitive and quantitative manner (see article by Majumdar and colleagues elsewhere in this issue). Nonetheless, because of the variety of imaging modalities in existence and the presence of numerous classification schemes in assessing disk degeneration, this has posed a dilemma in adopting a universal methodology to assess the phenotype of LDD. Therefore, heterogeneity exists in the imaging phenotype of LDD between various observational cohort and genetic studies.
Integration of knowledge of the human genome for genetic studies
The study of genetics was pioneered by Gregor Mendel who developed Mendel’s laws of genetics, which allowed differences in genetic material (ie, DNA) of organisms to be related to appearances or conditions that are observable (ie, phenotypes). Two major factors have advanced genetics even further: knowledge of all the variants present in our genome, and advancement in capturing these variations, or genotyping technologies.
Each unit of DNA consists of an alkaline structure, known as a base pair. Single-nucleotide polymorphisms (SNPs) are single base pair changes among individuals. Microsatellites are short, repeated DNA fragments. Copy number variations (CNVs) are longer (>1 kb, kilo base pair) DNA fragments that have a different number of copies compared with a reference genome. Before the first decade of the twenty-first century genetic studies mainly focused on microsatellite and SNP markers. Nowadays, considerable attention has been given to the importance of CNVs. Presently, in comparison with SNP and CNVs, microsatellites are seldom used for genetic studies.
Genome projects for human genetics
Improvements in genotyping technologies have enriched the knowledge on the relationship between genetic sequence and disease. The large-scale study of this relationship was made possible by the Human Genome Project, which provided the first reference human genome sequence. Later, the HapMap project was initiated, with the main goal of capturing the pattern of SNPs, the most common type of genetic variation among individuals, within the human genome. The initial stages of the project included Yoruban individuals from Africa, white individuals from the United States, Chinese, and Japanese individuals. Around 3.1 million SNPs were successfully genotyped. The success of the HapMap project was a big step forward in supporting genetic studies. It improved understanding of the linkage disequilibrium (LD) pattern within the human genome, making it possible to choose only 1 or a small number of tagging SNPs within a high-LD (low recombination rate) region to genotype to obtain almost complete common variant information on a particular genomic region. This process helps save a huge amount of resources for genetic studies. Recently, the 1000 Genome Consortium has completed its initial sequencing of 179 individuals, signifying the next era of genetic studies, which consists of sequencing each base pair of DNA, known as deep resequencing, as opposed to genotyping, which captures only known varying regions of the genome. In terms of disease genetic study, sequencing information makes it possible to study rare and previously unknown sequence variants. The 1000 Genome Project data are also valuable in allowing the genotypes of SNPs not genotyped in the experiment to be predicted by a computational process known as imputation. With the denser map, locations of disease-causing variants can be determined with more precision.
Genotyping technologies
Modern genotyping technologies are divided into 2 main categories: (1) those that are suitable for genotyping a larger number of SNPs and are thus suitable for genome-wide association studies (GWAS), and (2) those that are optimal for large samples size and are thus suitable for replication studies. The most popular GWAS genotyping platforms are Ilumina’s Infinium BeadChip and Affymetrix GeneChip, whereas other assays such as Perlegen and Invader are also available. Illumina BeadChip and Affymetrix GeneChip are now capable of genotyping more than 1 million SNPs and CNVs in the genome. Because of the design of the products, Illumina BeadChip has a higher genomic coverage, meaning that the SNPs of the product are more capable of capturing information about the genome. However, both Affymetrix and Illumina can also be used for genotyping smaller, more confined genotypic regions.
Sequenom MassArray is a platform used for a larger sample number with a small number of SNPs. I-plex assays for MassArray are used for genotyping up to 40 SNPs. Alternatively, the Taqman assay (from Applied Biosystems) uses a real-time method based on the polymerase chain reaction for SNP detection. Different alleles have different fluorescent probes. Therefore, the detection of different alleles can be achieved from signals given by their corresponding probes. The accuracy of this assay can reach up to 99%. Other genotyping assays, such as Pyrosequencing, are also available.
Main genetic study strategies
Linkage and Association Strategies of Genetic Studies
Genetic studies are divided into 2 main streams: (1) family linkage analysis, and (2) the case-control association approach. In family linkage analysis, the pattern of inheritance of a disease is compared with the pattern of disease markers. If a particular region of markers is observed to be inherited in the same way as the disease, then the disease-causing variant may lie within the region. Family data are required to perform linkage studies.
In the case-control association approach, unrelated individuals with extreme disease statuses, namely the case group (those with the phenotype) and the control (those without the phenotype) group, are recruited. The allele frequencies of the genetic variants between cases and controls are compared. Disease-causing variants are identified by searching for markers that show statistically significant differences in allele frequencies between the 2 groups.
Association within family trios can also be performed using the transmission disequilibrium test (TDT). This test is conducted by observing in heterozygous parents whether there is one allele with higher transmission frequency to the offspring than the other. This design is better than the case-control design because it avoids false-positives caused by the differences in population composition between the case and control groups. However, the power of this test is lower than that of the case-control design because the 2 parents are approximately equivalent in information to only 1 unrelated control subject.
Depending on the SNP selection strategy, the case-control association approach can be divided further into the candidate gene and genome-wide approaches. The candidate gene approach has the main advantage that it is more specific, because the selection of SNPs is based on prior knowledge on the biology of the disease. When a high-throughput genotyping platform is not so readily available, this approach has been shown to be successful in identifying several genetic risk variants for some complex diseases. Currently, because of the availability of whole-genome genotyping platforms, the genome-wide approach for association studies has been made possible. The main advantage of this approach is that regions of interest need not be confined in particular genomic regions, relying on investigators’ knowledge. Therefore, novel genetic variants are more readily identified.
Strengths and Weaknesses of Linkage and Association Approaches
Linkage studies were attractive at the time when genetic marker maps and genotyping technologies allowed few markers to be studied ( Table 1 ), because linkage can be detected at long distances from a disease susceptibility loci. Alternatively, an important disadvantage of linkage studies is that they are powerful only when the mutation has a high probability of causing disease (high penetrance). Association studies rely on LD between genetic markers and disease susceptibility loci, and this typically requires the 2 loci to be very close to each other. In consequence, association studies were for a long time confined to the study of specific candidate genes supported by a strong hypothesis, and a genome-wide screen using association was not feasible until the recent developments in genotyping technologies mentioned earlier. An important advantage of association rather than linkage is that association has greater power to detect a locus with small or modest effect size. However, linkage is more robust to allelic heterogeneity, which refers to the presence of multiple risk alleles at a locus. With modern sequencing technology, linkage and association are best considered to be 2 complementary ways of using genetic markers to study the genetic basis of simple and complex diseases.
Association (Candidate Gene Approach) | Association (Genome-Wide Approach) | Linkage | |
---|---|---|---|
No knowledge of gene functions required | × | ✓ | ✓ |
Localization to small genomic region | ✓ | ✓ | × |
Cost | ✓ | × | ✓ |
Families not required | ✓ | ✓ | × |
Not easily affected by population stratification | × | × | ✓ |
Power to detect common alleles (MAFs>5%) of modest effect | ✓ | ✓ | × |
Power to detect rare alleles (MAFs<1%) | × | × | ✓ |
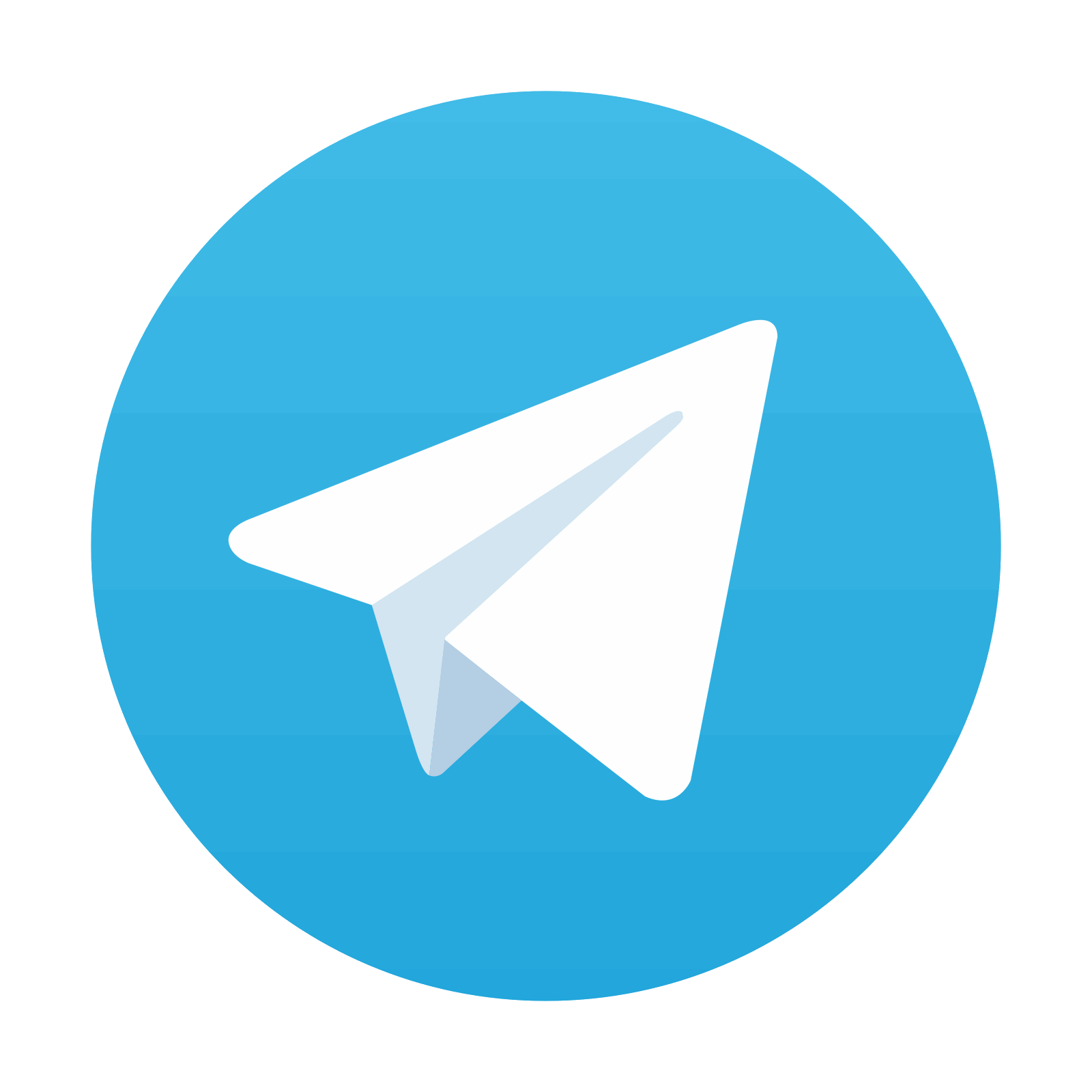
Stay updated, free articles. Join our Telegram channel

Full access? Get Clinical Tree
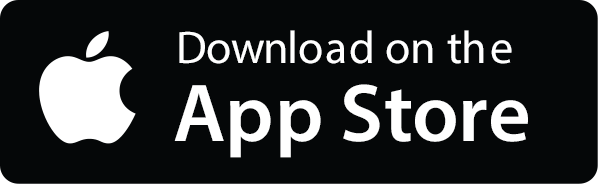
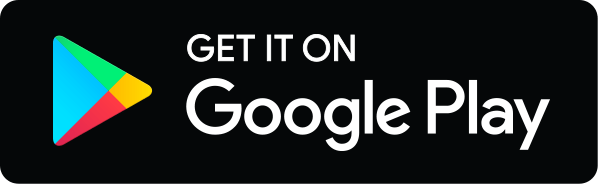
