Key Points
- •
Serum urate levels are highly heritable and principally determined by the fractional excretion of uric acid in the kidney. The heritability of gout remains to be properly established.
- •
Genetic association studies have identified many common variants that contribute to both the variation of serum urate and gout risk. These variants are predominantly expressed in the proximal renal tubule epithelium, where they mediate urate anion transport.
- •
Marked differences in the frequency of predisposing genetic variants exist between populations.
- •
Genetic studies do not support the conclusion that elevated serum urate causes cardiovascular disease. Comparative genetic studies suggest there has been evolutionary advantage in having high serum urate levels.
- •
The absolute contribution of genetic variants to levels of serum urate is relatively small in clinical terms. The most important gene identified to date, SLC2A9, accounts for 3.5% of the variation of serum urate.
- •
Recent advances allow the evaluation of existing and novel uricosuric therapies. Future discoveries may identify novel pathways in the pathogenesis of gout.
Introduction
Humans have high basal levels of serum urate due to mutations of the uricase gene, which render it inactive; consequently, humans, almost uniquely among mammalian species, are prone to gout. Over and above this specieswide increased baseline level of serum urate, a familial clustering of gout cases has long been recognized, although this reflects the importance of shared environmental influence as much as heritability. Initial progress in identifying genetic variants predisposing to gout came from linkage studies performed in rare lineages with monogenic disorders associated with gout and led to advances in the understanding of purine formation and metabolism. Subsequent studies established the control of serum urate to be highly heritable and largely determined by the fractional renal excretion of uric acid. More recently, genomewide association studies of quantitative traits have succeeded in identifying common variants in the general population that are implicated in the renal regulation of urate. It is not surprising that many of these variants have in turn been associated with the development of gout. This chapter will review the evidence for a genetic contribution to hyperuricemia and gout and discuss the approaches that have been used to find the underlying genes. The specific variants identified will be discussed in detail as well as the general insights that genetic studies have given into the role of urate transport in health and disease.
Heritability of Serum Urate and Gout
Initial studies into the heritability of serum urate drew conflicting conclusions on the pattern of inheritance observed, perhaps due to the heterogeneity in serum urate that is observed between populations. One approach to attempt to control for environmental factors has been an analysis of the strength of correlation between identical (monozygotic [MZ]) or nonidentical (dizygotic [DZ]) twins. If stronger correlation is observed between MZ twins than between DZ twins, then this allows an estimate to be made of the inherited contribution to a given trait. Such studies have demonstrated serum urate and subsequently also the renal fractional excretion of urate (FE UA ) to be significantly influenced by genetic factors. A correlation of 85% in the FE UA was observed between MZ twins compared to 66% in DZ twins with the overall heritability estimated at 87%, although with broad confidence intervals. Subsequently, segregation analysis in families, attempting to control for known environmental factors, has also shown that serum urate has a significant heritable component (heritability 0.4) with the overall pattern of inheritance seen best explained by a complex model incorporating interaction between more than one major gene, several modifying genes, and further environmental factors. This conclusion is broadly supported by the results of genomewide association studies, which have identified multiple genes influencing serum urate.
Many of the genes associated with hyperuricemia, as well as further candidate genes, have in turn been established as contributing to the risk of gout. Estimating the overall size of gout heritability, however, remains difficult, with a dearth of published information. One recent attempt to measure this using a twin study found no evidence of a genetic contribution to incident gout, although the study was not powered to detect a small effect. That serum urate might be the predominant heritable trait, rather than gout itself, might seem counterintuitive, yet a similar phenomenon is observed in the field of osteoporosis; the heritabililty of bone mineral density is well established, yet that of the clinically relevant endpoint of hip fracture has proved difficult to ascertain. This paradox appears to have been resolved by studies showing high heritability of fracture in those under the age of 65 but almost no heritable component after the age of 80, presumably reflecting the major influence of environmental risk factors for falling in the elderly. In gout, establishing the relative importance of genetic and environmental influence remains a major challenge.
Methods of Identifying Susceptibility Genes
Linkage Studies
Initial success in the identification of contributory genes came from the analysis of rare syndromes of hyperuricemia and gout using linkage analysis. In these studies, markers spread across the genome are analyzed in families harboring a disease, and loci common only to those individuals carrying the disease are sought. This approach has proved most useful in identifying rare genetic variants with powerful effect, although it has not been able to identify common genetic variation affecting the general population. The genes involved were often associated with the overproduction of urate. New methods have been required to identify more common variants of modest to weak effects, namely a candidate gene approach or genomewide association studies (GWAS).
Candidate Gene Studies
In candidate gene studies, polymorphic variants in a gene with a putative role in regulation of urate or gout are analyzed. Typically, a case-control design is used for a categorical trait such as gout, and statistical analysis of variance (ANOVA) is used between genotypes for quantitative traits such as serum urate. Although such studies are relatively straightforward to perform, there is an inherent risk of identifying spurious associations. This is particularly true where the samples size is small, but it can also be caused by mistakes in the study design, such as where different populations are recruited as cases and controls. Better design and power of such studies will help reduce these problems, but confirmation of any result requires replication of the finding in independent cohorts. Alternatively, where only a few variants have been assessed in a gene of interest, it is possible to miss a true association caused by further variant at an unrelated part of the gene. While better understanding of conserved genetic regions now allows the comprehensive coverage of variance throughout a gene with analysis of relatively few polymorphisms, it is salutary to note that regulatory variants may be far removed from the gene itself.
Genomewide Association Studies
The availability of mass genotyping technology has allowed the analysis of common genetic variation across the entire genome in large populations. Simultaneous measurement of quantitative traits allows the identification of discrete genomic regions in which greater than expected genetic variation correlates with increased levels of the trait under study, such as serum urate. To date, no GWAS have been performed in a gout population specifically. A major advantage of this approach is that it is hypothesis-free and so allows the identification of entirely unexpected genes or regions of interest and, consequently, novel pathogenic pathways. Powerful, mass genotyping techniques also have the potential to produce spurious results due to the inherent risk of chance observations caused by multiple testing or, again, through unrecognized population stratification. The solution is to look for replication in different populations or, where available, meta-analysis of such studies.
Overview
A large number of genes involved in the pathogenesis of hyperuricemia and gout have been identified through these approaches, which are discussed in detail later and illustrated in Figure 7-1 . Although the precise role of many of the common variants remains to be established, the majority appear to be involved in controlling the renal excretion of uric acid. This is to be expected given that reduced FE UA is the primary mechanism of hyperuricemia in more than 90% of patients with gout.

While there have been great advances in understanding as a result of these approaches, the variants thus far described collectively account for only a small proportion of the observed variance in serum urate, let alone the risk of gout. Performing GWAS in gout may uncover variants involved in novel pathways that contribute to crystal deposition or the subsequent florid inflammation observed. Already, the astonishing advances in mass genotyping techniques mean that GWAS are being replaced by whole exome or indeed genome sequencing. These techniques offer great promise in identifying novel, probably rare, variants; however, there will be new challenges in the rigorous analysis of such large volumes of data. Of course, there will always remain the need to perform the painstaking work of demonstrating the functional consequences of suggested mutations.
Single Gene Disorders Associated With Gout
A number of rare disorders have been described that are typically complicated by hyperuricemia and gout. Such diseases are frequently caused by mutations in a single gene and have demonstrated that both overproduction of urate and diminished renal excretion of urate can result in hyperuricemia and clinical gout ( Table 7-1 ).
Disease | Locus | Inheritance | Gene | Phenotype |
---|---|---|---|---|
Syndromes of altered purine metabolism | ||||
HPRT related | Xq26-q27.2 | XD | Hypoxanthine guanine phosphoribosyl transferase ( HPRT 1 ) | Hyperuricemia, gout, neurologic dysfunction |
PRPS related | Xq22-q24 | XD | Phosphoribosyl pyrophosphate synthetase 1 ( PRPS 1 ) | Hyperuricemia, gout |
Syndromes of excessive cell death and urate generation | ||||
Glycogen storage disease type Ia | 17q21 | AR | Glucose-6-phosphatase | Growth retardation, hypoglycemia, hepatomegaly, hyperuricemia, gout, lactic acidosis |
Glycogen storage disease type Ib | 11q23 | AR | Glucose 6 phosphate transporter | Growth retardation, hypoglycemia, hepatomegaly, hyperuricemia, gout, lactic acidosis |
Glycogen storage disease type III | 1q21 | AR | Glycogen debranching enzyme | Early-onset hyperuricemia, gout |
Glycogen storage disease type V | 11q13 | AR | Muscle glycogen phosphorylase | Early-onset hyperuricemia, gout |
Glycogen storage disease type VII | 12q13.3 | AR | Muscle phosphofructokinase | Early-onset hyperuricemia, gout |
Syndromes of reduced renal excretion of uric acid | ||||
Medullary cystic kidney disease, type 1 | 1q21 | AD | Unknown | Variable penetrance: renal dysfunction, hypertension, gout |
Medullary cystic kidney disease, type 2 | 16p12.3 | AD/AR | Uromodulin | Progressive renal dysfunction, variable hyperuricemia, early-onset gout |
Familial juvenile hyperuricemic nephropathy | 16p12.3 | AD | Uromodulin | Progressive renal dysfunction, variable hyperuricemia, early-onset gout |
Lesch-Nyhan Syndrome
Inactivating mutations in the hypoxanthine-guanine phosphoribosyl transferase ( HPRT ) gene, an enzyme involved in the purine salvage pathway, can cause hyperuricemia and gout in the form of Lesch-Nyhan syndrome. As discussed in detail in Chapter 3 , this is an X-linked recessive disorder associated with overproduction of serum urate, neurologic dysfunction, and behavioral disturbance. The neurologic deficits appear unrelated to hyperuricemia and are more likely explained by disturbance of purine-mediated neurotransmission.
Uromodulin-Associated Kidney Diseases
Both autosomal dominant and recessive syndromes of progressive nephropathy and early-onset gout have been described associated with mutations of the uromodulin gene. A variety of mutations lead to the defective transport and apical membrane expression of uromodulin, a protein expressed abundantly in the thick ascending limb of the loop of Henle. An early feature of these diseases is reduced fractional excretion of uric acid. Defective uromodulin secretion caused by protein misfolding correlates directly with decreased urine osmolarity and decreased urinary uric acid excretion, although the mechanism remains uncertain. It is interesting to note that a polymorphism associated with diminished uromodulin secretion is associated with a reduced risk of hypertension.
Other Monogenic Disorders
As reviewed in Chapter 3 , X-linked dominant inheritance of primary hyperuricemia and gout is seen with activating mutations in phosphoribosyl pyrophosphatase synthetase, an enzyme involved in urate synthesis. Gout and hyperuricemia can also complicate glycogen storage diseases and some other rare inborn errors of metabolism through the overproduction of serum urate induced by cell breakdown (see Table 7-1 ).
Candidate Genes Regulating Hyperuricemia and Gout
Great progress has been made in understanding the pathogenesis of hyperuricemia and gout through genetic association studies. The genes identified are listed alphabetically and summarized in Table 7-2 . The majority of genes identified in the regulation of serum urate are expressed in the renal proximal convoluted tubule epithelium, as illustrated in Figure 7-2 , emphasizing the importance of this region in the regulation of serum urate. ABCG2 may also act by mediating urate efflux into the small intestine, where urate can ultimately be degraded by uricase expressed in colonic bacterial flora. Similarly, extrarenal effects of SLC2A9 appear to be important given that variants of SLC2A9 associated more closely with the serum level of urate, rather than the FE UA .
Gene | Explained Variability Serum Urate | Effect Size in Gout | Populations in Whom Association With Gout Shown |
---|---|---|---|
ABCG2 | 0.57% | 1.7–2.0 | African American, Caucasian, Chinese, Japanese, Polynesian |
GCKR | 0.13% | … | … |
LRRC16A | 0.12% | … | … |
PDZK1 | 0.19% | … | … |
SLC2A9 | 3.53% | 1.3–5.0 | Caucasian, Chinese, Polynesian |
SLC16A9 | 0.17% | … | … |
SLC17 locus | 0.19% | 1.2–1.9 | Caucasian, Japanese |
SLC22A11 | 0.19% | … | … |
SLC22A12 | 0.13% | 1.2–1.8 | Chinese, Mexican American, Solomon Island |
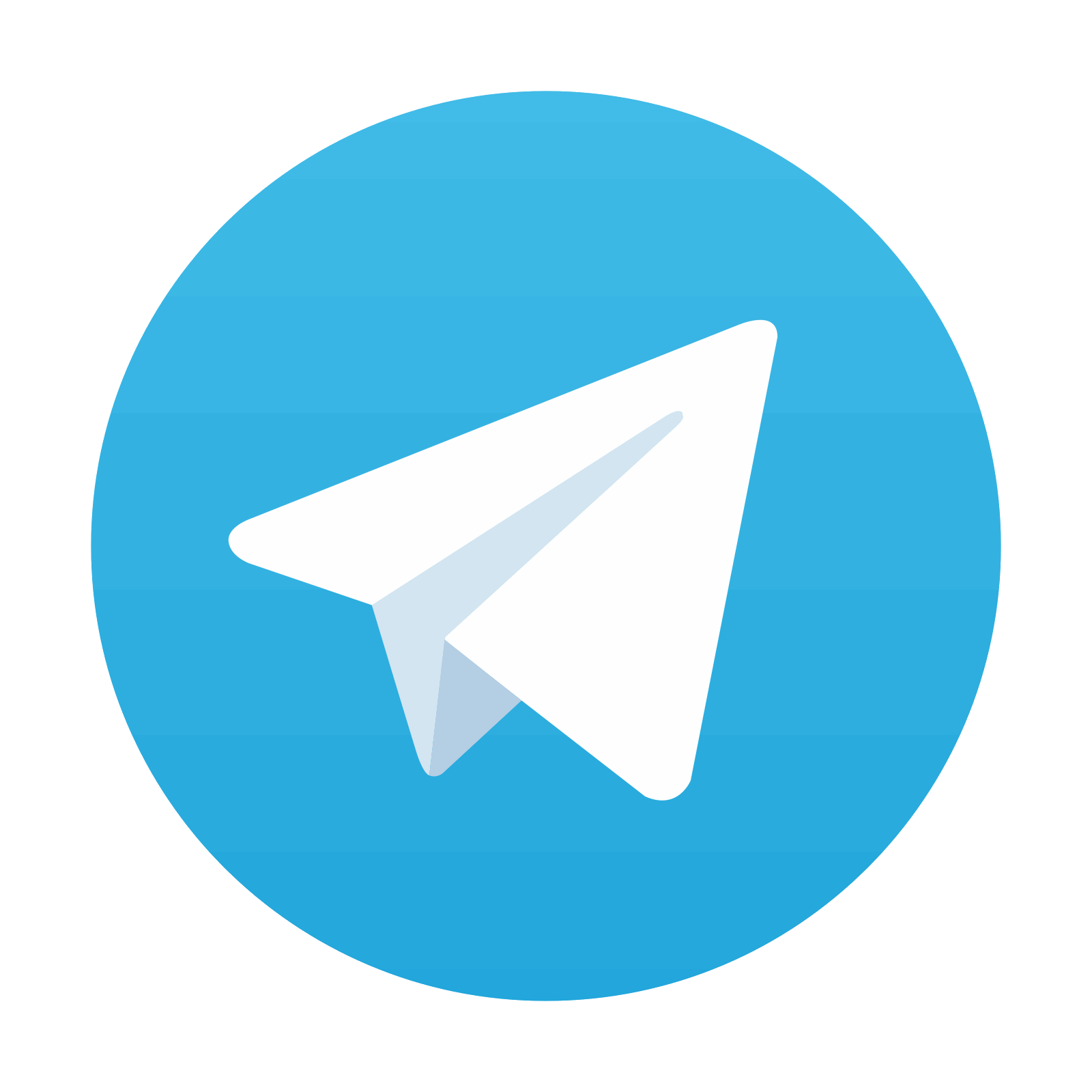
Stay updated, free articles. Join our Telegram channel

Full access? Get Clinical Tree
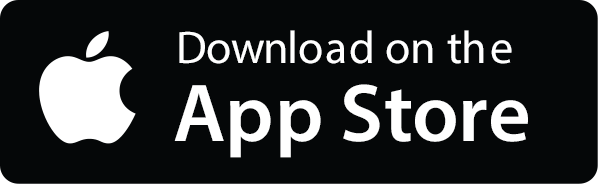
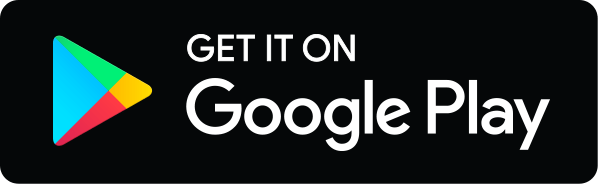
