Introduction
Although once considered rare among skeletally immature patients, anterior cruciate ligament (ACL) injuries have increased in frequency among children and adolescents over recent years. , The observed increase in the incidence of ACL injuries may be related to any combination of a rise in youth sports participation, single-sport specialization, increased training time and intensity, and enhanced recognition of ACL tears with improved diagnostic modalities. In fact, the rate of ACL reconstruction (ACLR) in children under 15 years of age increased by 924% between 1994 and 2006. With several studies reporting poor functional outcomes, higher rate of instability, new meniscal tears, and degenerative knee changes with nonoperative management, , , there has been an increasing trend toward earlier surgical management. The current literature describes a number of surgical techniques for pediatric ACLR. Early operative management has been recommended to restore knee stability and to prevent progressive chondral and meniscal damage, however, techniques vary among surgeons and there is not a consensus on a single operative technique. , , Although a number of complications described in adult patients apply to children and adolescents, the pediatric population is also uniquely at risk for additional issues before, during, and after surgery. Recognition and management of these issues is of paramount importance when caring for ACL injuries in children.
Preoperative Issues
Stiffness and Quadriceps Weakness
Patients with an acute ACL injury are often found to have knee stiffness at initial presentation due to inflammation and hemarthrosis. It is important to address this stiffness before surgery because poor preoperative motion is a major risk factor for postoperative motion deficits. To assess the effects of preoperative rehabilitation on postoperative functional outcomes, patients from the Delaware-Oslo ACL cohort were compared to those from the Multicenter Orthopaedic Outcomes Network registry. The authors reported that patients who were treated with extended preoperative rehabilitation had significantly higher functional outcomes and return-to-sports rates than those who did not receive preoperative rehabilitation. Similarly, others have advocated for the role of aggressive preoperative rehabilitation focusing on achieving full knee extension and quadriceps strength to reduce the chance for postoperative arthrofibrosis. The authors of this chapter recommend operative intervention only full range of motion (ROM) has been achieved, pain and effusion have subsided, and no extensor lag is present on physical examination.
Timing of Anterior Cruciate Ligament Reconstruction
The optimal timing of ACL reconstruction (ACLR) remains a controversial subject. It is generally recognized that the risk of postoperative arthrofibrosis is increased by poor preoperative ROM and quadriceps control, as well as reconstruction prior to resolution of the associated inflammation and effusion. , A classic study suggests this risk to be as high as 18% when ACLR is performed within 1 week following the injury, compared with 6% when it was delayed by more than 4 weeks. Subsequent investigations link early ACLR (within 3–4 weeks following the injury) to increased risk of postoperative stiffness and lack of full extension. ,
Conversely, several studies report increased rates of meniscus tears, chondral damage, and sports-related disability with delayed surgery. , , , The rate of medial meniscus tears is reported to be as high as 36% when ACLR is delayed for more than 6 weeks. With a similar delay, the odds of developing a lateral meniscus tear are 1.45 times higher. These odds increase to 2.82 when reconstruction is delayed for more than 12 weeks after injury. In a recent metaanalysis, nonoperative or delayed ACLR (30.3 ± 25.7 months after injury) was associated with a 34-fold increase in the risk of knee instability compared with early ACLR (12.9 ± 14.2 months after injury). In light of the trends favoring early surgical stabilization, the authors recommend deferring reconstruction until full range of motion is achieved, but generally not for more than 3 months after injury because this can significantly increase the risk of meniscal and chondral damage. Of note, early ACLR is especially advisable in the setting of multiligament injury. Although ligament reconstructions can be staged, delayed ACLR heightens the risk of interim meniscal and chondral injury in these patients. ,
Posterolateral Corner Injury
The posterolateral corner (PLC) of the knee primarily consists of the lateral collateral ligament (LCL), popliteus tendon, popliteofibular ligament, arcuate ligament, and joint capsule. In adults, a PLC injury is identified in up to 48% of all multiligamentous injuries of the knee, with only 28% of all PLC injuries occurring in isolation. , Conversely, the incidence of concomitant ACL and PLC injury was reported to be as high as 37.3% in a study of 102 children and adults. PLC injury often necessitates surgical management because nonoperative treatment in the setting of marked pathologic laxity may be associated with poor outcomes resulting from varus and rotational instability of the knee. , In patients undergoing ACLR, an unrecognized or untreated PLC injury results in significant strain on the ACL graft and high risk of graft failure. Additionally, instability resulting from an untreated grade III PLC injury can transfer excessive loads to the medial joint compartment, potentially resulting in degenerative changes in the long term. The PLC should be scrutinized in preoperative evaluation to avoid the potentially catastrophic consequences of a missed diagnosis. Physical examination maneuvers with proven utility in identifying PLC injuries include varus stress testing, the dial test, the reverse pivot shift test, and the external rotation recurvatum test. Table 12.1 describes these maneuvers. Magnetic resonance imaging (MRI) should be evaluated closely after a thorough physical examination.
Varus stress test | The varus stress test is performed with patient in supine position with knees flexed between 20 and 30 degrees. The examiner’s fingers are placed over the joint line, stabilizing the distal femur. Then a varus stress is loaded on the knee. Varus gapping occurs in patients with compromised posterolateral structures. |
Dial test | The dial test can be performed with the patient in either the supine or prone position with 30 and 90 degrees of knee flexion. It assesses the increased external rotation of the tibia compared to the contralateral uninjured knee. Increased external rotation at 30 degrees but not at 90 degrees indicates an isolated injury to posterolateral structures, whereas increased external rotation at both angles suggests injury to both posterolateral structures and the posterior cruciate ligament (PCL). , |
Reverse pivot shift test | The reverse pivot shift test can be performed with the patient supine on the examination table with knee flexed to between 70 and 80 degrees and external rotation and valgus force applied to the tibia. The knee is then allowed to be straightened. The test is considered positive when the lateral tibial plateau shifts from a position of posterior subluxation to a position of reduction as the flexed knee is brought into extension, resulting in a characteristic clunk. |
External rotation recurvatum test | The external rotation recurvatum test is performed with the patient supine by lifting the great toe and observing the relative amount of genu recurvatum present. The knee with a PLC injury will fall into relative hyperextension laterally, and the tibia will be externally rotated into relative varus. |
If a PLC injury with marked pathologic laxity is diagnosed along with ACL rupture, both should be addressed surgically. Primary repair of the LCL and popliteofibular ligament is associated with a significantly higher failure rate compared to reconstruction (37% vs. 9%, respectively). Therefore the authors tend to perform reconstruction of the PLC in all cases of concomitant injury. Reconstructions of the ACL and PLC can be performed simultaneously or in a staged fashion, with maintenance of knee ROM and stability key factors to consider.
Intraoperative Issues
Tunnel Malpositioning and Posterior Wall Blowout
Femoral tunnel malposition is the most frequently identified technical issue during ACLR, with the rates varying between 36% and 79%. The most common errors were too vertical (36%), too anterior (30%), or both (27%). A vertical femoral tunnel will result in rotational instability, while an anterior tunnel causes tightness in flexion and laxity in extension. Acceptable tunnel position is crucial because up to 25% of malpositioned femoral tunnels can result in graft failure. Malposition of the femoral tunnel has also been reported as the primary reason for reoperation in 52% of revision reconstructions. Although there is some debate about the ideal position of the femoral tunnel, it should generally be positioned posterior to the lateral bifurcate ridge for a single bundle ACLR with a few millimeters of bone remaining at the back of the posterior condyle ( Fig. 12.1 ). The tunnel should be near the 9- or 10- o’clock position in the coronal plane (in the right knee; 2- or 3- o’clock in the left) to ensure that the graft is relatively horizontal.

Although less frequent than femoral tunnel malposition, the incidence of tibial tunnel malposition ranges from 6% to 20%. , , A misplaced tibial tunnel can result in iatrogenic meniscal damage during drilling, intercondylar notch impingement (anterior or lateral malposition), or posterior cruciate ligament impingement (posterior or medial malposition). This tunnel should be placed between the medial and lateral tibial eminences and in line with the posterior edge of the anterior horn of the lateral meniscus ( Fig. 12.2 ). Radiographically, this tunnel can be placed between the medial and lateral eminences. Arthroscopically it should be within the ACL footprint in line with the anterior edge of the posterior lateral meniscus.

Technical intraoperative issues such as suboptimal degree of flexion during identification of the femoral footprint and arthroscopic image distortion have been described as common causes of tunnel malpositioning. Initial arthroscopic visualization of the lateral intercondylar wall through an anteromedial portal should be performed to properly identify peripheral regions prior to femoral drilling. The lateral wall should be visualized to observe the lateral intercondylar and bifurcate ridges as well as the outline of the cortical bone and overlying cartilage ( Fig. 12.3 ). The authors recommend at least 90 degrees of initial knee flexion when assessing the lateral wall, and then further flexion to 110 degrees for tunnel creation to maximize tunnel length and avoid cortical breach.

A femoral tunnel that is placed too posterior and too proximal can result in “blowout” of the posterior wall of the lateral intercondylar notch. Similar to the aforementioned issues of femoral tunnel malpositioning, this can be avoided by ensuring appropriate visualization of the lateral wall prior and employing provisional pin placement prior to drilling. If breach of the posterior cortex does occur, it is important that the surgeon does not rely on interference fixation within the notch, but instead converts to several salvage techniques which include the use of suspensory fixation, the two-incision technique, and cross-pin constructs.
Graft Choice
Various types of grafts, including hamstring tendon (HT) autografts, bone-patellar tendon-bone (BPTB) autografts, quadriceps tendon (QT) autografts, and allografts are used for ACLR in pediatric patients. In skeletally immature patients, soft tissue autografts are more commonly used over BPTB autografts because the latter may increase the risk of growth disturbance if a bone plug is placed across the physis. Limitations of HT grafts include a risk of saphenous nerve injury, , loss of knee flexion, and residual hamstrings weakness. Recently published studies utilizing American and Scandinavian registries show that, compared with BPTB grafts, HT grafts are more likely to require revision surgery, with the relative risk ratio ranging from 1.4 to 2.3. The higher rates of revision surgeries with HT autograft is likely due to the fact that HT grafts have a higher load to failure. Adding more strands to an HT graft makes it stronger and and potentially lowers the chances of graft failure.
Although BPTB autograft may have a lower rate of failure, there is increased risk of patellar fracture (up to 1.3%), anterior knee pain (up to 60%), knee extension deficits, , and arthrofibrosis (1.7-fold increase in the risk) compared with HT autograft. Additionally, BPTB autograft may exacerbate symptoms associated with preexisting conditions like concurrent patellar tendonitis, instability, maltracking, increased Q angle, or patellofemoral pain. Therefore, in the setting of significant patellofemoral symptoms, HT and QT autografts are reasonable alternatives. Additionally, grafting of the patella harvest site with bone harvested from the proximal tibia can minimize the risk of patellar fracture associated with BPTB.
QT autograft is another option that is gaining popularity but is less studied in the existing literature, especially in children. QT autograft is unique because it can be harvested with or without a patellar bone plug and is a reliably robust graft. Early evidence suggests that the rate of postoperative anterior knee pain may be lower than with BPTB grafts. When harvested with a bone plug, the risk of developing many of the same complications seen with BPTB autograft increases, including patellar fracture and knee extension weakness. The risk of these complications might be minimized by using QT without bone. The superiority of QT versus QT bone grafts is not yet clear based on current literature.
Allograft may also be used for ACLR but must be done with caution in young athletes. Recent data suggest that this is not used as the primary graft of choice owing to a higher failure rate in young, active patients. A study comparing allograft versus autograft tissue for ACLR in adolescents found that graft failure was 4.4 times greater in patients receiving allografts compared to patients receiving autografts. In an another study of patients younger than 18 years, the risk of revision surgery was found to be 15 times greater for patients initially treated with allografts compared to those treated with autografts. In the primary reconstruction setting in a young athlete, allograft is not a first choice, but can be used as an adjunct or considered in revision settings.
Although there is debate regarding optimal graft choice, the authors typically use QT autograft for the majority of reconstructions, especially for procedures performed in skeletally immature patients. BPTB autografts are sometimes used in older adolescents and revision reconstructions.
Graft Size
Graft size is a frequently discussed topic in pediatric ACLR. In this population, patient size may pose limitations on the type and size of graft utilized. Furthermore, if an all-epiphyseal technique is used to spare the physes, restricted epiphyseal space may necessitate a graft that is smaller than preferred. A graft diameter of 8 mm or more is considered optimal in the adult patient. Adult studies suggest that a diameter less than 8 mm increases the risk of failure nearly 7-fold, whereas the odds of graft rupture decreases by a factor of 0.82 with each 0.5 mm increase in graft diameter from 7 to 9 mm. However, the optimal graft diameter for pediatric ACLR remains controversial attributed to a lack of strong evidence on the subject.
Because HT autograft is often used in pediatric ACLR, it is important to recognize patient factors that are associated with tendon diameters less than 7 mm. These include height less than 140 cm, weight less than 50 kg, thigh circumference less than 37 cm, and body mass index less than 18 kg/m 2 . , Additionally, the tendons can be amputated prematurely during harvesting if fascial bands are not freed sufficiently, resulting in an undesirably short graft. We recommend the following tips to obtain the maximum length from grafts. Flex the knee while dissecting the soft tissues away from the hamstrings while manually palpating for fascial bands and using a right-angle clamp to pull the bands distally into the wound and incising the bands to allow for incision of the bands under direct visualization. In the event that the HT graft is thinner than desired, the overall diameter can be increased by folding one or both tendons as needed to create a graft with anywhere from 5 to 8 strands ( Fig. 12.4 ). , Although tendon length could theoretically be a limiting factor when fashioning a graft with more than four strands, this is rarely an issue in the authors’ experience. Augmentation with allograft is another option, although the literature reports mixed results with this technique. A study of 88 pediatric patients reported a failure rate of 11.9% with hybrid 6-strand grafts compared with 28.3% with 4-strand hamstring autografts. However, in a larger study of 355 pediatric patients, Pennock et al. concluded that augmentation of small grafts with allograft may actually lead to higher retear rates with earlier graft failure. The authors recommend aiming for a graft of 8 mm or larger in skeletally mature patients while balancing maximal graft size and available space for drilling in skeletally immature patients. Folding the graft to create additional strands, thereby increasing diameter, is preferred over supplementation with allograft.

Postoperative Issues
Arthrofibrosis/Stiffness
Although more common in adults, arthrofibrosis is a well-described complication following ACLR in pediatric patients. The true incidence of this problem in children is difficult to discern, as only one study has been published exclusively on pediatric patients. In this study of 902 patients, the rate of arthrofibrosis after ACLR was 8.3%. Significant risk factors were preoperative stiffness, female sex, age of 16 to 18 years, concomitant meniscal repair, and use of patellar tendon autograft. On the other hand, arthrofibrosis is less common in males aged 13 years and younger and in those undergoing reconstruction with an iliotibial band or HT autograft. Perhaps the best way to avoid this complication is to ensure full preoperative ROM, either with or without formal physical therapy.
Management of postoperative arthrofibrosis depends largely on timing and severity. Possible interventions include physical therapy, casting, dynamic splinting, continuous passive motion, manipulation under anesthesia, and arthroscopic lysis of adhesions. In one study, dynamic splinting restored significant motion, reducing the need for manipulation under anesthesia and surgical lysis of adhesions. In patients with persistent knee extension deficits beyond 3 months following ACLR, advanced imaging with MRI should be obtained to assess for anterior impingement with a cyclops lesion. Arthroscopy may also be warranted in those showing persistent knee stiffness despite focused rehabilitation for at least 3 months. Fig. 12.5 depicts an arthroscopic view of a cyclops lesion.

Graft Failure
Graft rupture following ACLR is a major complication in the pediatric and adolescent population. Although the exact frequency is somewhat unclear, it is well accepted that this number is higher than in adults, where graft failure occurs in approximately 3% to 4% of patients. In fact, the odds of retear have been shown to decrease by 9% with each year of increasing age. This is most likely because younger patients have a higher level of activity, making them more susceptible to reinjury. Technical factors such as graft type, graft size, and tunnel placement have all been suggested as risk factors for graft failure. In a retrospective review of 98 revision reconstructions in adolescents, Akhtar et al. found that the graft previously used for primary ACLR was HT autograft in 54% of cases and BPTB in 28%. Allograft has been shown to result in graft failure rates of up to 37% in young patients. , Although the influence of graft diameter on failure rate is unclear in the pediatric population, diameter smaller than 8 mm has been shown to contribute to higher retear rates in adults. Malposition of the femoral tunnel is another well-described risk factor for graft rupture, especially in early failures. In adults, up to 52% of all retears may result from tunnel misplacement.
In order to reduce the risk of graft failure, the authors recommend careful anatomic tunnel placement with special focus on avoiding a vertical femoral tunnel, fashioning a graft that is larger than 8 mm in diameter, and pretensioning the graft at 15 to 20 lbs on a graft board. In the event that graft rupture is diagnosed, the PLC must be examined closely for concurrent injury. The surgeon must also evaluate retained implants, position of the previous tunnels, and all other potential reasons for failure. If the tunnels are too large or otherwise unusable for revision surgery, they can be filled with bone graft, and revision ACLR can be performed later in a staged fashion. The authors will strongly consider an extraarticular anterolateral ligament (ALL) reconstruction or tenodesis in all revision cases. In an effort to lower the risk of graft failure, the authors also consider ALL reconstruction during primary ACLR for patients with knee hyperextension greater than 10 degrees and/or ligamentous laxity indicated by a Beighton–Horan score greater than 4 ( Table 12.2 ).
Description | Bilateral Testing | Scoring (max. points) |
---|---|---|
Passive dorsiflexion of the fifth metacarpophalangeal joint to ≥90 degrees | Yes | 2 |
Passive hyperextension of the elbow ≥10 degrees | Yes | 2 |
Passive hyperextension of the knee ≥10 degrees | Yes | 2 |
Passive apposition of the thumb to the flexor side of the forearm, while shoulder is flexed 90 degrees, elbow is extended, and hand is pronated | Yes | 2 |
Forward flexion of the trunk, with the knees straight, so that the palms rest easily on the floor | No | 1 |
Total | 9 |
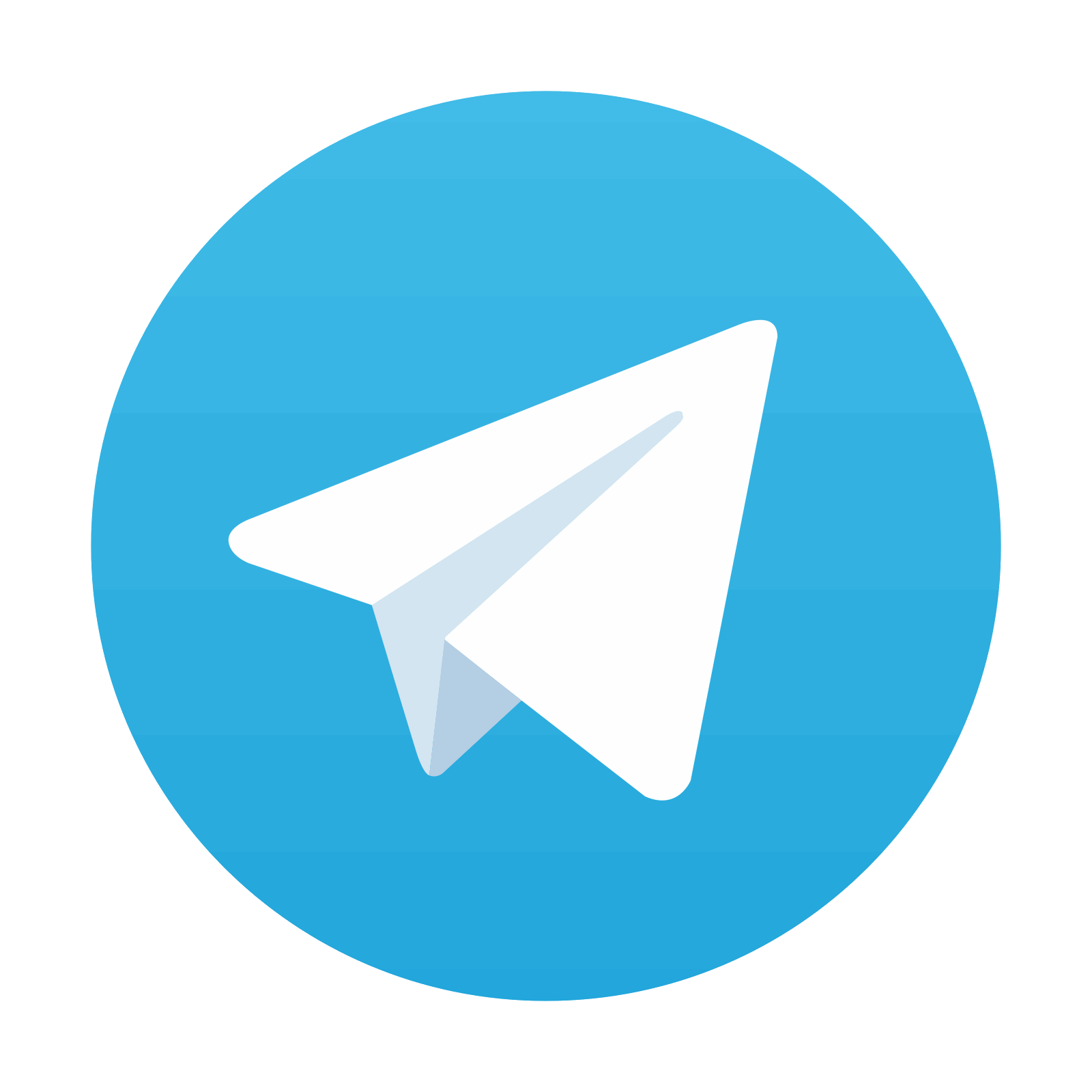
Stay updated, free articles. Join our Telegram channel

Full access? Get Clinical Tree
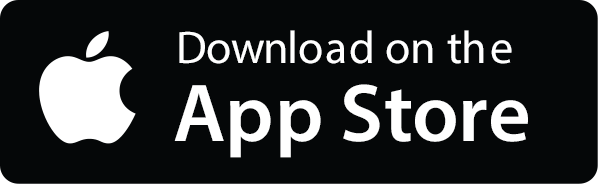
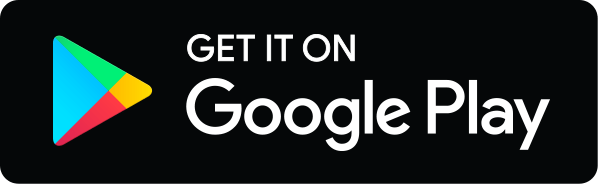
