Gait Analysis in the Child With a Limb Deficiency
Michael Aiona MD, FAAOS
Neither Dr. Aiona nor any immediate family member has received anything of value from or has stock or stock options held in a commercial company or institution related directly or indirectly to the subject of this chapter.
ABSTRACT
Gait analysis is an objective measure to analyze the motion and forces across the joints of the lower extremity. It can define deviations from normal gait and assist in clinical decision making. Gait analysis performed on children with limb deficiency is limited compared with that performed on adults. Energy consumption is greater in children with limb deficiency, with increasing cost as the level of deficiency moves more proximal, similar to adults, although exceptions exist. Children generally place more demands and force across the nonprosthetic side. The biomechanical aspects of the prosthetic foot design can affect the forces across the knee, allowing the clinician and prosthetist to choose which characteristics would be best for the individual patient. However, despite mechanical advantages of certain prosthetic foot designs, patient choice is not always toward the more advanced device. In a specific subpopulation of patients treated with a rotationplasty, controversy still remains regarding the efficacy of a mechanical knee compared with a biologic substitute (ankle) as gait studies differ in their findings. Regardless, the remaining length of the femoral segment and comparative knee axis height are important factors in overall function.
Keywords:
gait analysis; limb deficiency; pediatric
Introduction
It is important to know the historical development of motion analysis. The components of a gait analysis, including a description of the three ankle rockers, are key concepts. A literature review will highlight some objective findings relevant to the treatment of the child with a limb deficiency, divided into transfemoral and transtibial levels of deficiency. One specific condition, proximal femoral focal deficiency, is important to discuss with the noted differences in findings, along with gait analysis application and future developments.
History
Upright ambulation is one key evolutionary development that distinguishes humans from other mammals. Whether this occurred to free the upper extremities for more advanced tasks, see over the vegetation, or for more efficient locomotion is still debated. Although Aristotle authored the first known writings on human walking centuries ago, there was limited scientific investigation into this unique human characteristic as observation alone was not sufficiently precise because of perspective and the rapidity of movement. The advent of photography and a wagered bet among some industrialists initiated advancements that led to further insights into ambulation.
Known for his photographic skill documenting the landscapes in the Pacific Northwest for US government, Muybridge developed the technique of sequential serial photographs taken in rapid sequence during the movement of interest, in a quest to determine whether a galloping horse at some point had all four limbs off the ground (because this was not visible to the naked eye). This technique used multiple cameras (24) to take photographs in a timed sequential fashion, requiring the timed sequencing of light exposure and the camera shutter. Indeed, at some point the horse’s hoofs were all off the ground during a gallop.1 He invented the zoopraxiscope, which allowed the pictures to be shown in sequence rapidly, thus the beginning of a motion picture.
This success encouraged Muybridge, while at the University of Pennsylvania, to document many other movements, both in animals and humans. Many photographic sequences of human movement, including ballet, were documented by Muybridge and published in magazines and journals and combined in the book Animal Locomotion: An Electro-Photographic Investigation of Connective Phases of Animal Movements. Two more books were published, including The Human Figure in Motion, the first extensive book exploring many human movements.2 Over time, this foundational work was part of the slow but steady scientific investigation into human gait. Others worked on these techniques with a Frenchman, Étienne-Jules Marey, developing a technique called chronophotography, which took 12 pictures per second. Eventually Thomas Edison and
colleagues developed the kinetograph, where photographic film was in a long linear loop and was able to be shown through a projector, the Kinetoscope. He has been named the father of motion pictures for this development.
colleagues developed the kinetograph, where photographic film was in a long linear loop and was able to be shown through a projector, the Kinetoscope. He has been named the father of motion pictures for this development.
Although descriptions of gait have appeared over the centuries, there are drawbacks to observational gait analysis alone. The single point of observation is unable to incorporate all three dimensions of movement and the rapidity of movement makes precision limited. The addition of video adds more accuracy with its ability to slow down movement, but is not anatomic and lack biomechanical analysis, the ability to measure force.
Combining technical advances and scientific interest, Braune and Fischer3 incorporated their anatomic measurements to further elucidate the biomechanical functions of the musculoskeletal system, providing the foundation for much of the present-day modeling used in computerized gait analysis. Inman et al4 at the University of California, San Francisco and Berkeley, with the multidisciplinary team focused research on the biomechanical aspects of gait as it related to prosthetic design, applying their research to the clinical management of WWII veterans with a limb deficiency. Lamoreux5 developed an exoskeleton to attach to the pelvis and lower extremities with attached potentiometers to more precisely quantify kinematics during walking at various speeds on a treadmill. With the amount of manual work needed to extract and process the data significant and time consuming, it was difficult to move beyond the domain of the research community to the clinical setting.
With computer hardware and software technology advancing, the ability to perform mathematical calculations in a rapid manner held promise for the fledgling field of gait analysis and led to the development of a computerized three-dimensional (3D) gait analysis system. Combining the processing power of computers with the dense amount of data collected, the timely production of the graphs and numerical data became achievable with less human effort. The resultant increase in commercially available 3D gait analysis systems for researchers and clinicians made its clinical application a more widespread reality. Currently, a number of clinical laboratories for both the adult and pediatric population analyze human movement to improve performance and function and manage pathologic conditions.
Gait Analysis
The advantage of instrumented 3D gait analysis is its ability to define lower limb segment motion that is joint centered in three dimensions. It can provide information regarding gait symmetry; parameters such as cadence, step length, and support time; and pathologic motions. Most importantly, it can quantify force, power, and moments across joints that observational analysis is unable to provide.
Evaluation
Each motion analysis center will perform, at minimum, a clinical assessment consisting of a physical examination, 3D joint-centered kinematics, and kinetics. A technical and clinical summary with interpretation of the findings is part of the report. Additional evaluations may include electromyography, foot pressure analysis, foot kinematics, truncal motion, and oxygen consumption, although not all centers have these technical abilities. Because the focus of this chapter is gait analysis in the child with a limb deficiency, the three components of a standard assessment will be presented.
Clinical Assessment
A clinical history is obtained, with a focus on previous treatment and specifically the issue being assessed in that particular subject. A physical examination begins with a static alignment assessment of the trunk and lower extremities in a standing position with spinal alignment. Lower extremity alignment, limb length, and foot alignment with particular attention to any significant deformities or differences are assessed and documented. Dynamic assessment includes range of motion of the hip, knee, and ankle along with strength assessment. Motor control and tone assessment may also be evaluated. These important measures add valuable information in the final clinical interpretation of the gait study along with any treatment recommendations.
Kinematics
Kinematics describe the motion of lower extremity joints and limb segments, including the trunk. A set of retroreflective sphere-shaped markers are attached to anatomic points on the trunk and lower extremities specific to the proprietary motion analysis system used (Figure 1). Infrared cameras track the marker movement, with the frequency of data collection dependent on the rapidity of the movement of interest (Figure 2). The data are processed through
proprietary algorithms to generate a graphical representation of the trunk and lower extremity segment movements in three dimensions (Figure 3). Most of the clinical laboratories assume the foot is a rigid segment, although some have recently developed segmental foot models.
proprietary algorithms to generate a graphical representation of the trunk and lower extremity segment movements in three dimensions (Figure 3). Most of the clinical laboratories assume the foot is a rigid segment, although some have recently developed segmental foot models.
![]() FIGURE 1 Clinical photograph showing typical retroreflective marker set on anatomic positions. (Courtesy of Motion Analysis Center, Shriners Hospital for Children, Portland, Oregon.) |
![]() FIGURE 2 Photograph shows typical motion analysis with the walkway and cameras. (Courtesy of Motion Analysis Center, Shriners Hospital for Children, Portland, Oregon.) |
A complete gait cycle is defined from initial foot contact until it once again contacts the ground. Stance phase, 60% of the gait cycle, is divided into components: loading response, midstance, terminal stance, and preswing. Swing phase (40%) has three components: initial, mid, and terminal swing. Each of these events has defined specific kinematic and timing components.
Walking requires power generation to move the body center of mass forward. The displacement magnitude in all three planes while moving forward determines the amount of energy required, with less excursion translating into less energy consumption. Saunders et al6 described the major determinants of gait, which focused on movements of the pelvis and lower limb that minimized the displacement of the center of mass in normal gait, which is approximately 5° in all three planes. The greater compensatory motion at each joint to maintain this minimal excursion, the greater the energy cost as well. For example, athletes in the 110-m hurdle sprint are virtually performing leg splits to clear the hurdles
rather than jumping over the hurdles to minimize the up-and-down movement of their center of mass.
rather than jumping over the hurdles to minimize the up-and-down movement of their center of mass.
Kinetics
Kinetics in gait describes the forces affecting the motion of the limbs. Maintaining an upright posture requires controlling the bending moment across each joint. Some joints such as the hip three degrees of freedom, whereas others such as the ankle are much more limited to one plane of motion. When a foot is on the ground, body weight (force) is applying a bending moment across the joints of the lower extremity. The moments at each joint can be calculated using the components of the ground reaction force (GRF), measured by force plates embedded in the walkway, the motion graphs, and anthropomorphic data. The magnitude of this moment (M) is the product of the distance (D) from the joint axis of rotation times body weight (M = F × D) and can be resolved into three planes: sagittal, coronal, transverse (Figure 4). It is
bending the joint into a specific direction. The body controls this movement tendency by a reactionary force generated through muscle contraction and joint stabilizing soft tissue such as ligaments. Eccentric contraction, the elongation of a muscle while contracting, controls the velocity of joint motion to ensure the coordinated smooth movement of the trunk, pelvis, and joints of the lower extremity. Most of the muscle function is eccentric in normal walking.
bending the joint into a specific direction. The body controls this movement tendency by a reactionary force generated through muscle contraction and joint stabilizing soft tissue such as ligaments. Eccentric contraction, the elongation of a muscle while contracting, controls the velocity of joint motion to ensure the coordinated smooth movement of the trunk, pelvis, and joints of the lower extremity. Most of the muscle function is eccentric in normal walking.
Power (W) is joint angular acceleration times joint moment calculated at any point of the gait cycle. Concentric muscle contraction produces most of the power at the ankle, A2 push-off and the hip, H3 pull-off. The knee generates minimal power and is under more eccentric control and absorbing power.
Ankle Rockers
One specific area of focus is the design of various prosthetic foot/ankle replacements. This component is part of virtually all prosthetic devices, except for foot fillers in those with partial foot absence. The prosthetic foot is an important modulator of force across the ankle with extension to proximal joints. Three distinct ankle movements in stance phase were described by Perry and Burnfield7 as the three ankle rockers.
The first is ankle plantar flexion from initial heel contact to foot flat, comprising the first 15% of the gait cycle. This passive motion is produced by the GRF applied at the heel, posterior to the axis of rotation of the ankle, causing it to plantarflex. This motion is controlled by eccentric contraction of anterior compartment musculature of the tibia, mostly the tibialis anterior (Figure 5). It is important to note the GRF at this point is posterior to the axis of the knee, causing it to move into flexion. This must be controlled by eccentric contraction of the quadriceps (Figure 6).
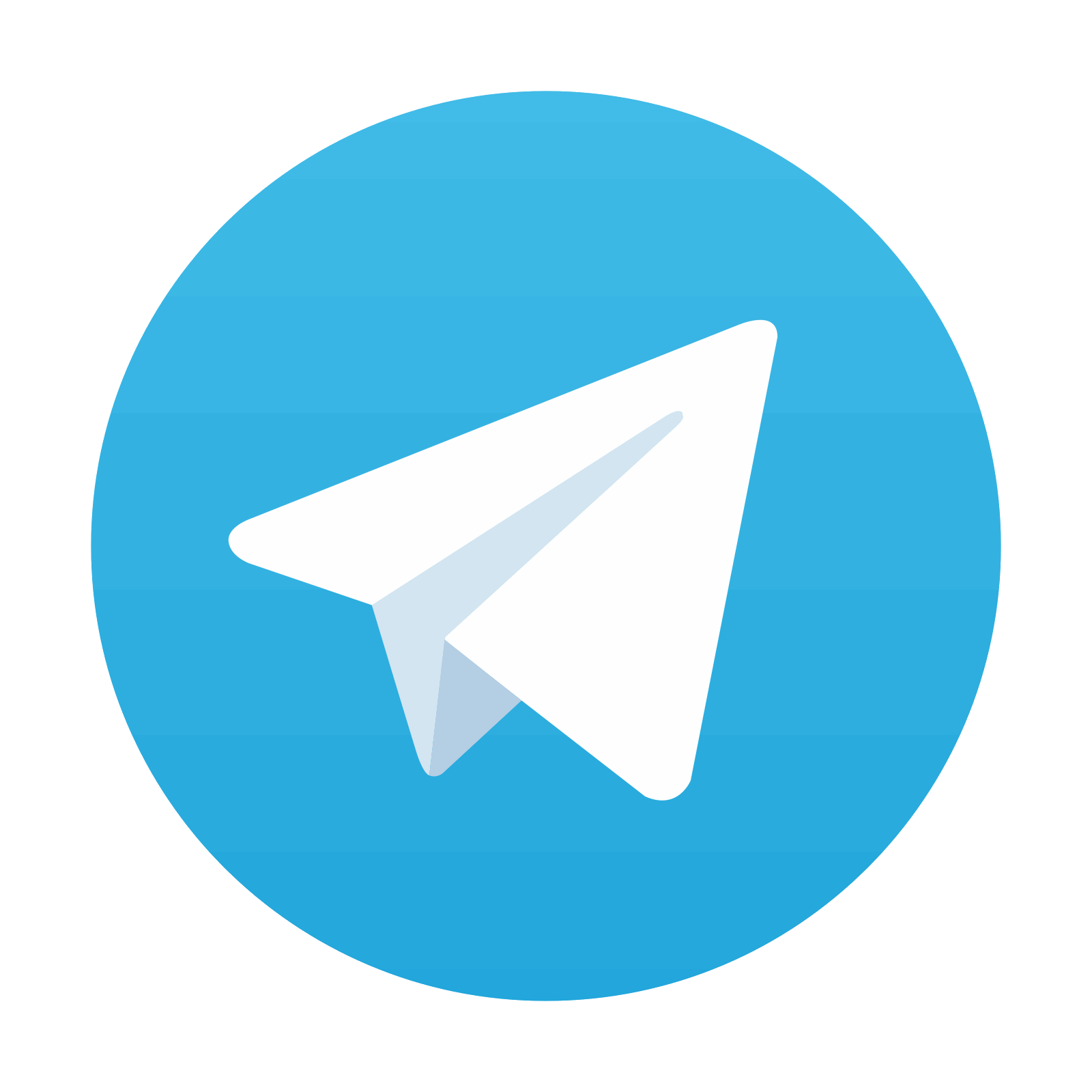
Stay updated, free articles. Join our Telegram channel

Full access? Get Clinical Tree
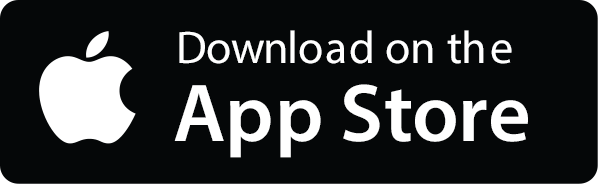
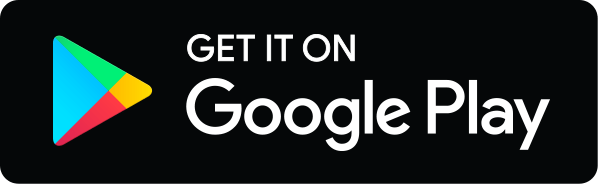