Fracture Repair
Hamish Simpson, BM, BCh, DM (Oxon), MA (Cantab), FRCS (Eng & Ed)
James Ryaby, PhD
Dr. Simpson is a member of a speakers’ bureau or has made paid presentations on behalf of Orthofix, Inc.; has received research or institutional support from Biocomposites, DePuy, A Johnson & Johnson Company, Orthofix, Inc., Smith & Nephew, and Stryker; and serves as a board member, owner, officer, or committee member of the British Orthopaedic Association, the British Orthopaedic Research Society, the Combined Service Orthopaedic Society, and the Orthopaedic Trauma Care Foundation Global Research Committee. Dr. Ryaby serves as a paid consultant to or is an employee of Orthofix, Inc.; has stock or stock options held in Orthofix, Inc.; and has received research or institutional support from Orthofix, Inc.
Keywords: fracture healing, fracture nonunion, osteoblast
INTRODUCTION
The WHO recognized trauma as a major healthcare epidemic with over 16,000 people dying each day and injury accounting for 16% of the global burden of disease.1 In the United States, approximately 60 million fractures occur each year.2 People in low-income settings are far more likely to suffer complications from traumatic injury than those in high-income settings.3 For every death linked to trauma there are many more injured, which causes considerable suffering. In addition, fractures often occur in patients of working age, and this can cause substantial economic hardship. Thus, fractures are one of the major causes of morbidity and create a significant drain on a country’s resources.
Fragility fractures in the older population create an additional layer of morbidity as they result in sustained loss of independence, and many patients are fearful that they may never regain their independence. As a consequence of this, 80% of patients suffering a fracture have stated that they would rather die than sustain a hip fracture.4
There is therefore a pressing need to ensure that patients’ fractures of all ages heal uneventfully in a timely manner. However, up to 9% of fractures fail to unite5 and cause considerable additional hardship. To minimize the rate of nonunion, it is essential to understand the principles of fracture repair.
However, bone healing is a complex process involving the activation of thousands of genes and the release of multiple growth factors and cytokines coupled with the proliferation and differentiation of progenitor cells,6 which all has to occur in a favorable mechanical environment.
FRACTURE PREVENTION AND REPAIR MECHANISMS
The skeleton is vital for a diverse range of mechanical activities essential for the existence of the human, such as respiration, mastication, locomotion, and protection of vital structures such as the brain; as well as biological functions such as calcium homeostasis and hematopoiesis. There are therefore a number of features of bone that maintain its integrity. These include both fracture prevention and fracture repair mechanisms. The basic processes that are relevant for fracture repair can be considered under the following categories:
1. Fracture prevention
2. Bone homeostasis
3. Bone maintenance
4. Wound healing
FRACTURE PREVENTION
Materials such as glass are brittle materials. A small crack in one region can propagate across the structure resulting in failure. Composite materials such as fiberglass and carbon fiber have numerous boundaries within the structure that prevent a crack from propagating across the material. Bone has such a composite structure with the organic component (primarily collagen) and the mineral component (primarily calcium hydroxyapatite). However, cracks can occur in the composite structure and, although they will not propagate instantaneously, they can accumulate in the material, and if they are not removed (see bone maintenance below), they can eventually result in catastrophic failure.
BONE HOMEOSTASIS
It has long been recognized that bone adapts to the loads to which it is subjected. This was encapsulated in Wolff’s Law7 in 1892. The original title was the law of transformation of the bone in which Wolff described the relationship between bone geometry and the mechanical influences on bone. Subsequently, Harold Frost proposed an updated concept—he coined the term, mechanostat to encapsulate the homeostatic concept of bone suggesting that bone growth and bone loss were stimulated by the local mechanical elastic deformation of bone. If a local region of bone experienced too high a strain in response to an applied load, then bone formation would be stimulated. Conversely, if no strain was experienced in response to an applied load then bone resorption would occur (Frost HN8). It is now generally considered that microscopic fluid movement within the lacunar-canalicular system is the major physical stimulus that osteocytes within bone sense.9,10
In a landmark paper by Rubin and Lanyon, the frequency of application of load rather than its magnitude was explored.11 Remarkably they demonstrated that only four consecutive cycles per day of external loading was sufficient to prevent disuse remodelling and maintain the bone mass. If there were fewer than this, disuse osteoporosis occurred. Thirty six cycles per day at 0.5 Hz resulted in considerable periosteal and endosteal new bone formation. Importantly, neither the size nor the character of the bone changes were affected by any additional increase in the number of loading cycles after 36 cycles. A possible mechanism for this daily memory was suggested by Skerry et al,12 who reported that the matrix became aligned with loading and maintained this structure for approximately 24 hours.
CLINICAL NOTE During fracture repair, a gradually increasing load would be expected to result in a stronger callus but maximum stimulus of the callus would be expected to be achieved after approximately 40 cycles of loading. Significantly, more cycles of loading beyond this would not be expected to provide any additional repair stimulus, but may put the callus at risk of mechanical breakdown and hypertrophic nonunion. This suggests that, in the early stages of fracture repair, progressively increasing the load patients take through the fractured limb but limiting the number of loading cycles may be most appropriate.
BONE MAINTENANCE
During regular activity, small amounts of subclinical damage occur to the bone in the form of microcracks. These microcracks are the structural manifestation of cyclical loading-dependent failure at the microscopic level. The extent of microdamage has been shown to be higher in individuals of older than 40 years.13 Bone has a remarkable system of removing these microcracks. Bone is thought to perceive and react to this through an osteocyte-mediated mechanism. The formation of linear microcracks leads to regulated (apoptotic) death of osteocytes in the region of the crack. Dying osteocytes trigger expression of receptor activator of nuclear factor-κ B ligand (RANKL) by neighboring surviving osteocytes, which results in osteoclast formation and bone resorption. If, however, the rate at which the cracks are accumulating exceeds the rate at which they are removed, then a fracture line will slowly propagate across the whole bone and result in a stress fracture. This is classically seen in the metatarsals of new recruits to the army but can also be seen in individuals with less intense physical activity, whose repair mechanisms are slowed. The removal of these microcracks requires osteoclasts to tunnel to the site of the microcracks and resorb bone. The exposed surfaces of the wall of the tunnel, behind the osteoclasts, are covered in osteoblasts that lay down layers of new lamellar bone. This whole structure is referred to as a cutting cone. These cutting cones are responsible for remodelling.
CLINICAL NOTE
Agents such as diphosphonates and denosumab block the maturation of osteoclasts and prevent the formation of cutting cones. Prolonged usage of these agents results in accumulation of the microcracks and eventually to atypical fractures.
Primary bone healing utilizes the process of remodelling and, therefore, may be inhibited by diphosphonates and denosumab.14
WOUND HEALING
The body’s standard response to injury is an acute inflammation response. This involves an initial stage of hematoma formation, followed by a phase of inflammation and demolition, and finally a phase of ingrowth of granulation into the tissue. In the inflammatory phase, the fibrin exudate and neutrophil polymorph infiltrate are replaced with a mononuclear cell infiltrate, and this indicates the onset of the demolition phase in which macrophages engulf fibrin and cellular infiltrate. Following this, granulation tissue is formed by the proliferation and migration of the surrounding connective tissue elements consisting of capillary loops and fibroblastic cells. This process of healing is used in a specialized way in secondary bone repair.
TYPES OF FRACTURE REPAIR
PRIMARY BONE HEALING (DIRECT OR OSTEONAL BONE REPAIR)
Primary bone healing occurs when accurate reduction and rigid internal fixation has been performed. The central components of primary bone healing are “cutting cones” which consist of a spearhead of osteoclasts that cut a space in bone. Within this space, there is a central blood vessel and the walls of the cavity behind the osteoclasts are lined with osteoblasts, which lay down new bone. Multiple cutting cones traverse the fracture gap perceiving it to be a “giant microcrack.” Eventually, the fracture line is obliterated as it is removed and is replaced with new bone by the osteoblasts (Figure 1).
In order for a fracture to heal by primary bone healing, the fracture needs to be fixed rigidly (ie, the strain of the facture surface needs to be less than 2%) and there needs to be no significant gap between the bone surfaces (ie, less than ˜ 0.1 mm). This process does not have an inflammatory phase but does require active osteoclasts and may, therefore, be impaired by agents that inhibit osteoclasts such as diphosphonates.
GAP HEALING
Gap healing is a modified form of primary bone repair. This occurs if a fracture has been rigidly fixed but the gap at the fracture site is in the order of a few hundred microns. In this situation, immature bone is initially laid down along the fracture surface of each bone end so that the gap now becomes small enough to allow cutting cones to traverse the fracture as in primary bone healing.
SECONDARY BONE REPAIR
The majority of fractures heal by secondary bone repair which is a form of wound healing. It can be considered to consist of five stages—ie, (1) hematoma, (2) inflammation, (3) soft callus, (4) hard callus, and (5) remodeling; however, in practice, there is a continuum and many of these phases overlap (Figure 2).
HEMATOMA
Immediately after a fracture, bleeding from the bone surfaces and from the surrounding soft tissue occurs. This blood clot is rich in a number of factors such as VEGF (vascular endothelial growth factor). These factors are important in initiating the inflammatory response and recruiting cells and blood vessels to the site of injury. It has been shown that removal of the
hematoma results in delayed fracture healing and the absence of the hematoma in open fractures is likely to contribute to the impaired healing in these patients.15
hematoma results in delayed fracture healing and the absence of the hematoma in open fractures is likely to contribute to the impaired healing in these patients.15
INFLAMMATORY PHASE
In the inflammatory phase, neutrophil polymorphs infiltrate the hematoma and these are then replaced with a mononuclear cell infiltrate of macrophages, which engulf fibrin and red cells. In addition, other inflammatory cells such as T-cells, B-cells, and mast cells also play a role. Inflammatory cytokines such as tumor necrosis factor-alpha (TNF-α), the interleukins (ILs), specifically IL-1, IL-6, IL-11, and IL-18, recruit inflammatory cells and stimulate angiogenesis.16 TNF-α acts through its receptors TNFR1 and TNFR2, which are expressed on osteoblasts and osteoclasts. TNFR2 is only present after injury, indicating it may have a particular role in fracture repair. IL-1 is secreted by macrophages, which regulates the expression of the cyclo-oxygenase enzymes (Cox1 and Cox2) that make prostaglandins at the fracture site.17 NSAIDS inhibit these enzymes and may inhibit the repair process. In addition, IL-1 induces osteoblasts to produce IL-6 and stimulates angiogenesis at the fracture site. IL-6 also stimulates angiogenesis and in addition stimulates the differentiation of osteoblasts and osteoclasts.18 Factors in the complement cascade also have a role in fracture repair; however, at present this is poorly defined.19,20
SOFT CALLUS
In this phase, organization of the blood clot occurs with invasion of capillaries and fibroblasts. The macrophages, present at the fracture site, begin to change their phenotype from a proinflammatory one induced by toll-like receptors (TLR) to an anti-inflammatory phenotype.
Fibroblasts and chondrocytes lay down a fibrocartilaginous matrix between the fracture ends. A bridge of soft callus forms where the tissue is being subjected to a strain of less than 20%. The muscle and the deep cambial layer of the periosteum contribute to the external callus. The medullary tissue and the bone ends contribute to the internal callus. Mesenchymal stem cells (MSCs) are derived from these tissues, and recent
evidence indicates that they are recruited from the circulation (Granero-Molto et al21 and Kitaori et al22). Stromal cell derived factor-1 and its receptor CXCR-4 appear to be crucial for the homing of appropriate MSCs to the fracture site.21,22,23 However, lineage tracing work indicates that most of the cells are derived from the periosteum, endosteum and bone marrow.24
evidence indicates that they are recruited from the circulation (Granero-Molto et al21 and Kitaori et al22). Stromal cell derived factor-1 and its receptor CXCR-4 appear to be crucial for the homing of appropriate MSCs to the fracture site.21,22,23 However, lineage tracing work indicates that most of the cells are derived from the periosteum, endosteum and bone marrow.24
TNF-α also has a key role in recruiting osteoprogenitor stem cells, stimulating apoptosis of hypertrophic chondrocytes and enhancing the recruitment of osteoclasts to the calcified cartilage callus.25
HARD CALLUS
In some respects, adult fracture repair recapitulates embryonic endochondral ossification (Figure 3). In both processes, MSCs condense at the sites of bone formation. The process involves activation of transcription factors such as Hoxa 4, Cart 1, CBFA 1, and Sox 9 as well as growth factors such as those of the transforming growth factor beta (TGF-β) superfamily (including the bone morphogenic proteins [BMPs]).26
In addition members of the Wnt family of molecules, which are known to have a major role in development, have also been shown to contribute to fracture repair and to regulate the differentiation of MSCs.27
As the recruited MSCs differentiate into chondroblasts and osteoblasts, they are stimulated to form type 2 and type 1 collagen, by members of the TGF-beta superfamily, such as BMP-2 which is important for the initiation of healing. BMP-5 and BMP-6 are important for intramembranous ossification and TGF-β2 and growth/differentiation factor-5 (GDF-5) for chondrogenesis during endochondral ossification.28
In this phase, the matrix becomes mineralized; calcium-containing granules are accumulated in the cytoplasm and then transported to the extracellular matrix where they combine with phosphate and precipitate and become the nidus for apatite crystal formation.29
As the soft callus becomes stiffer, the tissue strains reach a level where bone can form and osteoblasts are able to ossify the tissue. Provided there are regions where the callus is now under the breaking strain of bone (ie, approximately 2%), a bridge of hard callus is able to form. If there is excessive movement at this stage and there are no zones where the strain is under 2%, then a hypertrophic nonunion will occur. The hard callus initially consists of a “repair” form of bone (ie, woven bone) which consists of type 3 as well as type 1 collagen.
REMODELLING
Once the bone ends have been joined together by a solid bridge of woven bone, this tissue is gradually replaced with lamellar bone by a cutting cone process called remodeling. This new bone is laid down along the lines of stress in response to the mechanical requirements of the bone. The process of secondary bone healing is remarkable in that the bone regenerates without a scar and reforms healthy new bone.
MECHANICS OF FRACTURE REPAIR
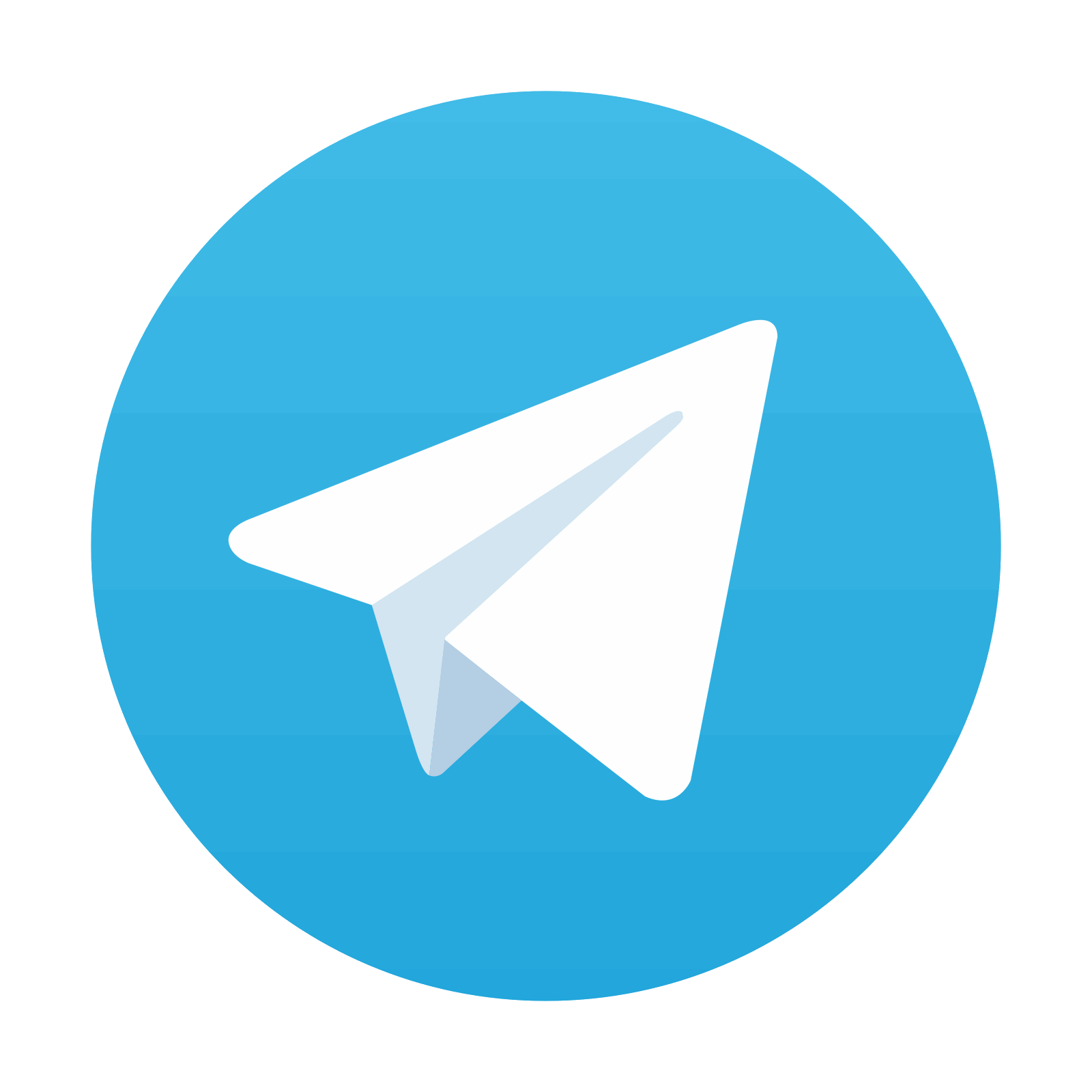
Stay updated, free articles. Join our Telegram channel

Full access? Get Clinical Tree
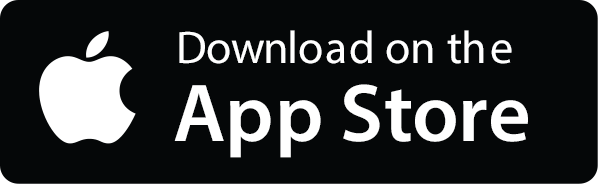
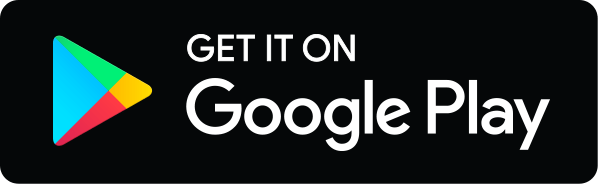