CHAPTER OBJECTIVES
After studying this chapter, the learner will be able to:
Describe a pedicled and a free flap.
Assess and determine an appropriate classification for a specific flap.
Distinguish the vascular anatomy of the skin, muscle, fascia, and perforator flaps.
Integrate the concepts of angiosomes and venosomes and their effects on flap design.
Discuss flap physiology, including delay phenomenon and tissue expansion.
Assess and monitor a flap for tissue viability.
Recognize common flap complications.
Define the types of skin grafts.
Recognize skin anatomy relevant to flaps.
Integrate skin graft healing physiology into a plan of care.
Recognize signs of graft failure.
Explain relevant elements of donor site selection.
Primary closure of a surgical wound is the simplest and fastest way of approximating wound margins; however, in many instances it is neither feasible nor desirable to close a wound with this method. Using principles of moist wound healing results in a wound being left open to heal by secondary intention. Primary closure results in minimal scarring and re-epithelialization; wounds left to heal by secondary intention may have more extensive scarring with subsequent contraction and deformity.
When there is a relative or absolute soft tissue deficit appropriate for coverage, a flap or skin graft can be used to fill the defect. The extent of the deficit and its location, among other factors, dictate the type of coverage needed. The first section of this chapter discusses and illustrates different types of flaps, followed by a succinct description of flap monitoring and common complications. The second section demonstrates the principles and applications of skin grafting.
While this chapter is not an exhaustive reference on the subject, it is intended to be comprehensive to wound care providers, focusing on essential concepts related to flaps and grafts that will help the clinician understand the indications and postoperative care. For those readers who require more detailed information on the topics presented in this chapter, a thorough reference list is provided for further study.
A flap is a unit of vascularized tissue that may be transferred from one part of the body to another.1 It is more simply defined as specific tissue that is mobilized on the basis of its vascular anatomy.2 A flap may contain a single tissue (for example, skin, muscle, fascia, fat, bone, tendon, nerve) or a combination of tissues. A flap may also be comprised of enteric components such as jejunum, colon, stomach, or omentum. The critical concept to understand about flaps is the relationship to its blood supply, which is necessary for the flap to survive. Because of the thickness and/or composite nature of flaps, the tissue being transferred cannot initially survive by diffusion from the recipient bed; it must have its own inflow and outflow of blood. A flap is termed pedicled if it maintains its blood vessel continuity at all times and is raised and/or transposed on the pedicle; it is termed a free flap if the vein and artery of the pedicle are cut and re-anastomosed to other blood vessels. The term free tissue transfer may also be used to describe a free flap.
The dichotomy between a pedicle and a free flap is easy to understand but fails to classify flaps in a useful way. Over the years, different classifications have been used to categorize flaps according to various predominant factors. Some of the useful classifications are vascular supply, tissue composition, transfer method, and vascular orientation.
Classification by vascular supply is based on the type of vascularization of the flap and is utilized for cutaneous flaps, whether alone or combined with another tissue.
This concept is only applied to skin. Random cutaneous flaps are based on random nondominant contributions from the dermal and subdermal plexus.1 Rather than having a definite blood vessel system to supply its surface, a random flap relies on the interconnection between specific vascular territories and the vascular skin plexus.1 The critical concept in a random flap is its width-to-length ratio. A length twice as long as its base (ratio 2:1) is generally considered the limit for most tissue; however, it may be greater in the face and scalp but smaller in the lower extremity.3 Exceeding a 2:1 length-to-width (base) ratio predictably results in distal flap necrosis. A classic example of a random flap is a transposition flap as illustrated in FIGURE 9-1.
FIGURE 9-1
Random flap Illustration of a random pattern flap in a transposition rectangular flap (2:1 ratio). (Used with permission of Losee JE, Gimbel M, Rubin J, Wallace CG, Wei F. Plastic and reconstructive surgery. In: Brunicardi F, Andersen DK, Billiar TR, Dunn DL, Hunter JG, Matthews JB, Pollock RE, eds. Schwartz’s Principles of Surgery. 10th ed. New York, NY: McGraw-Hill; 2015:chap. 45.)

An axial flap is based on longitudinal vessels along the pathway of the flap. The significant advantage of an axial flap over a random flap is the capability to extend the design outside the 2:1 ratio. In fact, an axial flap can be as long as the length of the blood vessel included in the flap. In this type of flap, the main axial artery with its venae comitantes gives rise to multiple branches that feed and drain the cutaneous portion adjacent to the flap. A classic example is the groin flap, based on the superficial circumflex iliac artery.
A musculocutaneous flap involves a combination of skin and muscle. The vascular pedicle supplies a muscle, which at the same time provides perforator branches to the skin. An example of a musculocutaneous flap is the transverse rectus abdominis flap (TRAM) used frequently in breast reconstruction.
In a fasciocutaneous flap, the fascia is the recipient of a named vessel. Once the artery reaches the fascia, it gives perforator branches to the skin. An example of a fasciocutaneous flap is the anterolateral thigh flap (ALT) with the lateral circumflex femoral artery (LCFA) as a pedicle. The artery reaches the vastus lateralis and its fascia before giving perforator branches to the skin.
When perforator branches do not have to go through a muscle or a fascial layer, they travel between structures. The space where these vessels travel is termed a septum; therefore, the term septocutaneous flap. Interestingly, the ALT flap, with the same pedicle (LCFA), may have perforator branches traveling in the septum between the vastus lateralis and the rectus femoris muscles, reaching the skin without crossing through the vastus lateralis or its fascia. In this case, the flap is labeled a septocutaneous flap.
The tissue composition classification is based on the actual tissue type involved in the flap, which can be a single tissue or a composite of tissues. The vascular supply is indirectly implied as being included in the flap and, therefore, is not named.
A single tissue flap is referred to by its main tissue. For example, a flap containing only the rectus abdominis muscle is a muscle flap; a flap composed of the temporoparietal fascia is a fascial flap. A flap can also contain skin, bone, omentum, or colon. (This list is not exhaustive.)
When more than one tissue are combined into a flap, it is labeled using the main tissues involved. Examples include a musculocutaneous flap or an osteofasciocutaneous flap. Many potential combinations are possible.
Classification by transfer method refers to the spatial displacement of a flap from a fixed point or a base. Consequently, it applies only to local pedicle flaps.
Advancement flap describes the procedure when a flap is advanced directly forward into a defect without any rotation or lateral movement (FIGURE 9-2).4
FIGURE 9-2
Example of advancement flap Advancement flap describes the procedure when a flap is advanced directly forward into a defect without any rotation or lateral movement.4

A rotational flap describes the procedure in which a semicircular flap is rotated about a pivot point into the defect to be closed (FIGURE 9-3).5
FIGURE 9-3
Example of rotational flap A rotational flap is a semicircular flap that is rotated about a pivot point into the defect to be closed.5

A transposition flap is a combination of rotation and advancement of a single flap to close a defect (FIGURE 9-4).
An interpolation flap is a two-stage tissue flap in which the base of the flap is not immediately adjacent to the recipient site.6 For this type of flap, normal tissue is interposed between the base of the flap and its insertion. The forehead flap is a classic example (FIGURE 9-5).
FIGURE 9-5
Example of interpolation flap An interpolation flap is a two-stage tissue flap in which the base of the flap is not immediately adjacent to the recipient site.6 In this type of flap, normal tissue is interposed between the base of the flap and its insertion. The forehead flap is a classic example, as shown here as a paramedian forehead flap.

A flap can be named according to the direction of its blood flow. This classification is not specific but is used to describe a specific pattern of vascularization.
An anterograde flow flap maintains the normal pattern of the arterial flow. For example, a pedicle radial forearm flap based proximally has an anterograde arterial flow in the radial artery.
In a retrograde flow flap, the arterial blood flow does not travel through the usual pattern but instead has its flow reversed due to transection of the anterograde dominant blood flow. An example is a reverse posterior interosseus flap in which the arterial flow in the posterior interosseus artery (PIA) originates normally from the ulnar artery and the common interosseus artery. In this flap, when the PIA is transected proximally, blood is flowing reversely from an anastomotic branch of the anterior interosseus artery located in the distal forearm.
A flow-through flap can be used to bridge an interrupted arterial flow by anastomosing the proximal and distal pedicle, thereby restoring flow distally. The first flow-through flap was used to connect the external carotid to the facial artery with a radial forearm flap. In this case, the radial artery was used to reestablish arterial circulation.
A venous flap has no arterial pedicle, only a venous pedicle(s). The physiology of these flaps is more complex and only small flaps can survive on venous inflow only.7
Flaps are based on an absolute understanding of their vascular supply. Without a thorough appreciation of the delicate and complex vascular anatomy of each specific tissue, the surgeon cannot harvest a flap in a safe and efficient way. Most flaps are composite flaps with each component having its own vascular territory. For the sake of clarity, in this text a perforator artery is defined as an artery that supplies the skin; a branch artery is defined as an artery that supplies a muscle or fascia.
The skin can be nourished via direct connections from an artery; otherwise, the artery has to penetrate through various tissues, the most important being muscle and fascia. Once the artery reaches the skin, its vascular supply forms five different plexuses.2,5 A vascular plexus can be simply described as a network of anastomoses between blood vessels (FIGURE 9-6).
The skin microcirculation can be described using another model. The arterioles and venules within the dermis form two horizontal plexuses: an upper horizontal network in the papillary dermis and a lower horizontal plexus at the dermis-subcutaneous tissue junction. Muscle and fat tissues host the perforating vessels, which ultimately create the lower plexus. The newly formed arterioles and venules connect to the upper horizontal plexus, irrigating epidermal appendages at the same time (FIGURE 9-7).2,6
Muscle flaps are, in their simplest form, individual muscles harvested with the vascular pedicle. However, muscles are not vascularized uniformly and the understanding of the vascularization pattern is of paramount importance when harvesting a muscle flap. In a landmark article published in 1981, Mathes and Nahai described five patterns of muscle vascular anatomy (FIGURE 9-8 and TABLE 9-1).8
Type I: One vascular pedicle. In this type of flap, the entire muscle is dependent on its main blood supply, meaning that the entire muscle can survive on a single artery and vein. The disadvantage of a type I muscle flap is that if the vascular pedicle is severed, the muscle will not survive. The tensor fascia lata or the gastrocnemius are muscles that can be used for type I flaps.9
Type II: One or more vascular pedicle(s) and minor pedicle(s). Muscles in this group have a dominant pedicle that can sustain the whole muscle and a minor blood supply that is incapable of solely maintaining muscle viability. In this case, a muscle flap can be harvested on a dominant pedicle and minor pedicles can be ligated without affecting the subsistence of the flap. Examples include the gracilis or rectus femoris muscles.
Type III: Two dominant pedicles. These muscles have two dominant pedicles that can each maintain adequate blood flow if harvested individually. As long as one of the two pedicles is intact, the flap will remain viable. A classic example is the rectus abdominis in the TRAM flap with two main vascular trunks. A pedicled TRAM uses the superior epigastric artery and veins while a free TRAM utilizes the inferior epigastric system. Another example is the pectoralis minor muscle, which can be harvested using the thoracoacromial artery or the lateral thoracic artery.
Type IV: Segmental vascular pedicles. These muscles are vascularized by multiple branches that are each responsible for the survival of a segment of the muscle. The origin and insertion of the muscle are considered pedicles. The primary limitation of a type IV muscle becomes obvious during flap harvest. Since each segment is not well connected to the other vascular segment, only a limited number of branches can be safely ligated and still maintain survival of the entire muscle flap. If too many branches are severed, segmental flap necrosis will occur. Examples include the sartorius or the external oblique muscles. These muscles cannot be used as a free tissue transfer but are useful as a rotational pedicle flap. Sartorius flaps, raised by ligation of two to three branches, can be used to cover an exposed femoral artery and vein.
Type V: One dominant and secondary segmental pedicles. In these muscles, the multiple segmental pedicles are capable of adequately vascularizing the flap as well as the single dominant pedicle. The flap can then be based on either of the pedicles for a pedicle flap. If free tissue transfer is performed, then the flap must be based on the dominant pedicle. Examples include the latissimus dorsi or the pectoralis major. A latissimus dorsi flap can be distally based on its lumbar branches or it can be used as a free tissue based on the thoracodorsal vascular trunk.
FIGURE 9-8
The vascular anatomy of muscles (experimental and clinical correlation) Patterns of vascular anatomy, first described by Mathes and Nahai in 1981, are also described in Table 9-1. (Used with permission from Mathes, N. Classification of the vascular anatomy of muscles: experimental and clinical correlation. Plast Reconstr Surg. 1981;67(2):177–187. https://journals-lww-com/plasreconsurg/Citation/1981/02000/Classification_of_the_Vascular_Anatomy_of_Muscles_.7.aspx.)

Classification | Vascular Supply | Example |
Type I | One vascular pedicle | Gastrocnemius |
Tensor fascia lata | ||
Type II | Dominant and minor pedicles (the flap cannot survive based only on the minor pedicles) | Gracilis |
Rectus femoris | ||
Type III | Two dominant pedicles | Rectus abdominis |
Pectoralis minor | ||
Type IV | Segmental pedicles | Sartorius |
External obliques | ||
Type V | One dominant pedicle with secondary segmental pedicles (the flap can survive based only on the secondary pedicles) | Pectoralis major |
Latissimus dorsi |
A muscle flap can be combined with other tissues to create a composite flap, the most common being the musculocutaneous flaps. Muscles give rise to multiple arterial branches, termed perforator arteries, which travel through the fascia to supply the skin. In order to achieve better vascularization of the skin, the connections between the muscle and skin are maximized during flap surgery. Every muscle that has skin in continuity can include a portion of skin when it is used for a flap. This attached section of skin is termed a skin paddle. A rectus abdominis or a latissimus dorsi muscle can easily be combined with a skin paddle. A pectoralis minor flap, however, does not have direct connection to any skin and, therefore, cannot include a skin paddle.
A fasciocutaneous flap is composed of skin, subcutaneous fat, and fascia. Its main vascular supply irrigates deep fascia that permits vascularization to a more or less well-defined skin territory. The first fasciocutaneous flap was described by Pontén in 198110 and a more formal classification was developed by Cormack and Lamberty in 1984. Four types of vascular patterns were originally identified.11
Type A: Multiple fasciocutaneous vessels. These flaps have multiple perforators oriented in the long axis of the flap in the predominant direction of the arterial plexus and at the level of the deep fascia. An example is a rotational flap in a lower extremity.11 As in segmental muscle flap (type IV), survival of the flap is directly related to the number of segmental vessels included in the flap.
Type B: Single fasciocutaneous vessel. These flaps have a single vessel responsible for vascularizing the entire flap. According to the authors, the essential characteristic of the flap is a T-junction on the single vessel feeding the fascial plexus.11 An example is the medial arm flap based on the superior ulnar collateral artery.
Type C: Multiple small fasciocutaneous vessels in a septum. These flaps have branches originating from an axis vessel; however, they travel between muscles in a septum. A classic example is the radial forearm flap where the numerous perforators to the skin and fascia originate from the radial artery and travel in between the brachioradialis (BR) and the flexor carpi radialis (FCR). The main difference between type A and type C is the fact that the main vessel is included in the flap in a type C. Therefore, a type C can be converted into a free tissue transfer but a type A cannot.
Type D: Osteomyofasciocutaneous flap. This flap is an extension of type C and as such is often included in this category. In this flap, the fascial septum is included as well as the adjacent muscle and bone along with their blood supply. An example is the radial forearm osteofasciocutaneous flap including the radius bone with a cuff of muscle including fascia and skin.
Fascial flaps do not need a skin paddle and are therefore often harvested as a pure fascial flap without a skin paddle. Examples include a temporoparietal fascia flap or a radial forearm fascia flap.
For fasciocutaneous flaps, another classification by Mathes and Nahai deserves mentioning because of its simplicity and frequency of use. This classification is based on the pathway of the perforator to the skin and includes three types (TABLE 9-2).2,3,5
Type A: Direct cutaneous perforator. In these flaps, a perforator artery originates from an axial vessel and travels directly to the skin without having to travel through a muscle or a septum. Examples include the groin flap, based on perforators from the superficial circumflex femoral artery or the temporoparietal fascia based on branches from the superficial temporal artery.
Type B: Septocutaneous perforator. In these flaps, perforators travel from the originating vessel to the skin between muscles via a septum. The primary advantage of these perforators is that during harvesting of the flap, the vessel course is easily identifiable in a septum and because of the absence of side branches, dissection is rapid and straightforward.
Type C: Musculocutaneous perforators. Perforators that travel through a muscle to access the skin are termed musculocutaneous perforators. Unlike type B perforators, vessels in muscle are more difficult to dissect if the muscle is not included in the flap. Close proximity of muscle fibers to blood vessels and numerous small branches makes dissection of perforators more tedious.
Classification | Vascular Supply | Example |
Type A | Direct cutaneous vessel that penetrates the fascia | Temporoparietal fascial flap |
Type B | Septocutaneous vessel that penetrates the fascia | Radial artery forearm flap |
Type C | Musculocutaneous vessel that penetrates the fascia | Transverse rectus abdominis myocutaneous flap |
The concept of perforator flaps emerged from the postulate that a single perforator artery could vascularize a definite skin territory and its subcutaneous component without having to include the adjacent muscular tissue. The first reported perforator flap was described by Koshima in 1989 in which an abdominal skin paddle was harvested without the rectus abdominis muscle, including only the skin and adipose tissue, and was vascularized by a single perforator of the deep inferior epigastric artery.12 This flap is now known as the deep inferior epigastric perforator (DIEP) flap.
Numerous perforator flaps have since been described. When an artery is dissected from its arborization into the skin to its proximal origin, it is termed a perforator flap. Perforator flaps differ from conventional musculocutaneous or fasciocutaneous flaps by way of their anatomic origin. Skin and subcutaneous tissue are supplied by the following five vascular plexuses: subepidermal, subdermal, dermal, subcutaneous, and fascial (sub- and suprafascial). Conventional flaps originate to vessels beneath muscle layers or within the fascia. In contrast, perforator flaps are supplied by the more distal subcutaneous or subdermal vessels, which allows for elevation of just the skin paddle without the requirement of other tissues.13
In an attempt to define and classify perforator flaps, Blondeel et al. defined a perforator flap as the following: “A perforating vessel, or, in short, a perforator, is a vessel that has its origin in one of the axial vessels of the body and that passes through certain structural elements of the body, besides interstitial connective tissue and fat, before reaching the subcutaneous fat layer.”14 Some authors make a distinction between a direct (only goes through fascia before reaching skin) and an indirect perforator (goes through other tissues before reaching skin).15 In 2003, the Gent consensus was published proposing a classification and a nomenclature scheme (TABLE 9-3 and FIGURE 9-9).14
FIGURE 9-9
The Gent classification illustrated (Used with permission from Blondeel PN, Van Landuyt KHI, Monstrey SJM, et al. The “Gent” consensus on perforator flap terminology: preliminary definitions. Plast Reconstr Surg. 2003;112(5):1378–1383. https://journals-lww-com/plasreconsurg/Abstract/2003/10000/The__Gent__Consensus_on_Perforator_Flap.24.aspx.)

Type 1: Direct perforators perforate the deep fascia only. The perforator artery runs from its main vessel directly to the deep fascia. |
Type 2: Indirect muscle perforators predominantly supply the subcutaneous tissues. A perforator travels through a muscle but mainly vascularizes a skin territory; few branches are going into the muscle. |
Type 3: Indirect muscle perforators predominantly supply the muscle but have secondary branches to the subcutaneous tissues. |
Type 4: Indirect perimysial perforators travel within the perimysium between muscle fibers before piercing the deep fascia. |
Type 5: Indirect septal perforators travel through the intermuscular septum before piercing the deep fascia. |
This classification was simplified in 2003 with only three types of flaps included (TABLE 9-4 and FIGURE 9-10).16 Despite these efforts, controversy still exists among the definition of perforator flaps, and there have been calls to refine the terminology.2,17
Type 1: Indirect “muscle” or myocutaneous perforators traverse through muscle to pierce the outer layer of the deep fascia to supply skin. |
Type 2: Indirect “septal” or septocutaneous perforators traverse only through a septum to reach the deep fascia and the skin. |
Type 3: “Direct” perforators perforate the deep fascia only. (From Blondeel PN, Van Landuyt K, Hamdi M, Monstrey SJ. Perforator flap terminology: update 2002. Clin Plast Surg. 2003;30(3):343–346.) |
To standardize nomenclature of perforator flaps, the Gent consensus proposed that “A perforator flap should be named after the nutrient artery or vessels and not after the underlying muscle. If there is a potential to harvest multiple perforator flaps from one vessel, the name of each flap should be based on its anatomical region or muscle.”14 For example, a perforator flap based on the thoracodorsal vessels, the main vessel for the latissimus dorsi, should be labeled a thoracodorsal artery perforator flap (TAP flap).
Since their discovery, over 350 perforators have been identified. Perforator flaps can be elevated as pedicled or free flaps.18 In 2009 Saint-Cyr et al. introduced the term “perforasome” to describe the cutaneous territories supplied by various perforator vessels (FIGURE 9-11).19 The authors mapped and compiled the perforators and their associated tissues via injection and imaging. This concept is similar to the term angiosome which describes the vascular territory supplied by each artery. (More information on angiosomes follows.)
FIGURE 9-11
Common perforasomes and flow direction (Used with permission from Saint-Cyr, M., Wong, C., Schaverien, M., Mojallal, A. & Rohrich, R. J. The perforasome theory: vascular anatomy and clinical implications. Plast Reconstr Surg. 2009;124:1529–1544. https://journals-lww-com/plasreconsurg/Abstract/2009/11000/The_Perforasome_Theory__Vascular_Anatomy_and.20.aspx.)

Perforator flaps have become a mainstay of reconstruction. The DIEP flap is now the preferred technique in breast reconstruction as it offers excellent aesthetic outcomes and decreased donor site morbidity compared to previous techniques such as the transverse rectus abdominis myocutaneous (TRAM) flaps.20 Other techniques such as profunda artery perforator (PAP), thoracodorsal artery perforator (TDAP), and superior gluteal artery perforator (SGAP) flaps have been described and are now commonly used for a variety of reconstructive purposes, including but not limited to head and neck reconstruction, abdominal wall reconstruction, and extremity reconstruction.21,22
The perforator flap offers many advantages over the conventional myocutaneous flap. The wealth of perforators provides an abundance of donor sites. Perforator flaps do not require elevation of the underlying muscle; therefore, the muscle can be spared and donor site function is preserved, as opposed to myocutaneous or myofascial flaps. Unlike conventional flaps, perforator flaps can be oriented in any direction on the perforator, which allows for optimal defect coverage. Perforator flaps can be much more versatile than conventional flaps because they can be readily thinned and shaped to conform to the defect, they can be oriented freely based on the pedicle, and they have a longer pedicle.23 These properties may stem from the observed phenomenon that pedicled flaps are hyperperfused compared to normal circulation.24 However, these benefits do not come without a cost. Perforator flaps often require tedious, time-consuming dissection, which can prolong operative time. In addition, the distribution of perforator vessels is less consistent than larger vessels, necessitating more preoperative studies and intraoperative effort.25
Perforator flaps are a powerful tool in the reconstructive surgeon’s armamentarium. The increase in popularity of these flaps has been supported by improved understanding, which has enabled perforator flaps to supplant many conventional flaps. The mantra of “flap of choice” is slowly fading away as the versatility of perforator flaps continues to evolve and expand.26 Continued study is needed to better characterize and define the vascular distribution, and future investigation will refine the use of these flaps as well as potentially discover their new applications.
Since its inception in 1991, the propeller flap has undergone a series of transformations to maximize tissue coverage and improve its use in soft-tissue defect reconstruction throughout the body. The original flap described by Hyakusoku et al. was adipocutaneous in nature and consisted of a skin island with two “propeller blades” at each end of a vascular pedicle.27 Much like a propeller, the flap was then rotated 90° around its pedicle for the release of scar contractures at the axilla and elbow. A modified version of the original propeller flap was later described by Hallock and included two major changes: as skeletonized perforating vessel and a flap rotation angle of 180°.28
The different types of propeller flaps commonly used in current practice are described in a consensus by the Advisory Panel of the First Tokyo Meeting on Perforator and Propeller Flaps.29 The “Tokyo” consensus, analogous to the “Gent” consensus on perforator flaps, describes propeller flaps as an “island flap that reaches the recipient site through an axial rotation.”30 This definition excludes flaps whose principal movement is advancement and flaps that are not designed as a complete island.
The “Tokyo” consensus also sets forth how to classify propeller flaps.29 Proper terminology necessitates identifying the type of nourishing pedicle, the degree of skin island rotation, and the artery of origin of the perforator vessel, if known. The three following types of propeller flaps are distinguished based on the type of nourishing pedicle: (1) The subcutaneous pedicled propeller flap is analogous to the original propeller flap described in 1991, where a subcutaneous pedicle of unknown vascular origin is rotated 90° without the need to visualize or isolate perforator vessels, followed by direct closure of the donor site. (2) The perforator pedicled propeller flap incorporates the perforator flap concept, leading to a propeller flap supplied by a perforator vessel that determines the borders of the skin island overlying it. This perforator vessel is visualized, dissected, and skeletonized, allowing for a rotation up to 180° as opposed to the original subcutaneous propeller flap. Freeing the vessel from fascial adhesions minimizes the risk of vessel buckling during flap rotation. The greater range of rotation inherent in the pedicled propeller flap design makes it the most commonly used type of propeller flap. (3) The supercharged propeller flap is a modified version of the pedicled propeller flap that allows for greater tissue coverage. Its design involves the dissection and anastomosis of an extra vascular pedicle to enhance arterial inflow, venous outflow, or both. As such, it is used if extra flap length is needed that would not be sufficiently supplied by a single perforator vessel, or for salvage procedures (FIGURE 9-12 and TABLE 9-5).
FIGURE 9-12
Illustration of the three types of propeller flaps Subcutaneous pedicled propeller (top), perforator pedicled propeller (middle), supercharged propeller (bottom). (Used with permission from Pignatti M, Ogawa R, Hallock GG, et al. The “Tokyo” consensus on propeller flaps. Plast Reconstr Surg. 2011;127(2):716–722. https://journals-lww-com/plasreconsurg/Abstract/2011/02000/The__Tokyo__Consensus_on_Propeller_Flaps.27.aspx.)

Flap Type | Characteristics | Technique |
Subcutaneous pedicled propeller | Random subcutaneous pedicle of uncertain origin, up to 90° rotation | Macroscopic (vessel not visualized) |
Perforator pedicled propeller | Skeletonized perforating vessel, origin known from anatomy or directly visualized, up to 180° rotation | Magnification needed (vessel visualized and dissected) |
Supercharged propeller | Skeletonized perforating vessel plus extra vein, artery or both, origin known from anatomy or directly visualized, up to 180° rotation | Microsurgical (vessel visualized and dissected, plus microsurgical anastomosis) |
An updated “Tokyo” consensus was proposed by Toia et al. in 2017, whereby a new type of propeller flap is described that does not fit into the established classification. The newly proposed axial pedicled propeller flap is by definition a propeller flap since it involves an island of tissue that reaches its recipient site through axial rotation; however, its pedicle is neither subcutaneous nor perforator, but rather comes out of the bone to enter the flap perpendicularly.31 Two such types of flaps have been reported in the literature: the supratrochlear artery axial propeller (STAAP) flap and the deep lingual artery axial propeller (DLAAP) flap.31
Propeller flaps are an attractive reconstructive option due to their versatility. Reconstruction can be performed in the upper and lower extremities, the head and neck, the trunk, abdomen, and buttocks. In order to be successful, the defect should be in a well-vascularized territory, be small to medium sized, and be surrounded by healthy tissue.32
There are many advantages to using propeller flaps, which set it apart from other techniques. The most obvious benefit is the great mobility, which can reach 180° of rotation, allowing for maximum utilization of the flap. The design of the flap allows for partial coverage of the secondary defect (often by primary closure) and scar manipulation, which is especially beneficial in cosmetically sensitive areas. Another advantage of propeller flaps is their ability to exceed the traditional 3:1 length to width ratio; in fact, successful flaps with a ratio of greater than 6:1 have been reported.33 This may be due in part to the ability of perforator vessels to adequately perfuse beyond their angiosome/perforasome territory into those of their neighbors.34 In all, these flaps are able to offer robust coverage with skin that offers similar quality and type, which can be used in a variety of applications.35
No flap is perfect, and propeller flaps do have disadvantages that must be carefully considered when they are used. The complication rate can be as high as 31% in lower extremity reconstruction due to the lack of well-vascularized recipient tissue. Certain demographics such as infants and the elderly have higher rates of complications as well.32 As stated, these flaps cannot cover as great of an area as can others, due to being elevated on a single pedicle. Rotation of the flap can cause undue tension on the pedicle and the donor site, which must be carefully monitored. Finally, as with other flap modalities, the dissection can be arduous, as perforator anatomy can be varied.32
Propeller flaps, when properly utilized, can be an excellent reconstructive option that may become more prevalent as they are continually studied. Although propeller flaps have lower complications than conventional pedicled flaps, there are currently few studies analyzing risk variables and propeller flap complications such as venous congestion, superficial necrosis, and flap failure.36,37 Consistent risk variable documentation by providers and future multicenter studies will help identify what patient factors and flap characteristics are more likely to result in success or complications.
In 1987, Taylor and Palmer defined the concept of an angiosome. They described the skin perfusion as a 3D jigsaw puzzle where each artery originating from a source vessel perfuses a skin territory with its underlying structure.38 For example, the deep inferior epigastric system vascularizes a skin territory labeled the deep inferior epigastric angiosome. Each defined artery supplying the skin has its own angiosome.
Angiosomes are not isolated entities and are related to each other by connecting vessels called choke vessels. These vessels are at the margin of adjacent angiosomes and can be more or less open to allow perfusion from one territory to another. This concept will be later explained in the delay phenomenon section.
The venous system is composed of a deep and a superficial system, connected by perforator veins. An artery may have one or two veins with a similar anatomical pathway. Such a vein is labeled vena comitans or venae comitantes in the plural form. (Comitans comes from Latin and means to accompany.) For example, a radial forearm flap may have the cephalic vein as a superficial venous drainage system as well as two venae comitantes along the radial artery. These two systems ultimately join near the elbow via a communicating vein. Of note, some arteries do not have vena comitans. For example, the carotid artery does not have any accompanying vessels per se.
Using the same concept as angiosomes, Taylor et al. described a comparable venosome concept. A close relationship was found between the arterial and venous territories of each muscle.38 Instead of having choke vessels on the periphery of their territories, venosomes have oscillating veins that have the unique characteristic of no valves. In contrast, venae comitantes have valves to direct blood flow toward the source artery.39
The elevation of a flap has a major impact on local blood flow. The angiosome concept explains the interconnection between different vascular territories. During a flap harvest, blood inflow and outflow are often restricted to a single pedicle; the numerous small connections between the flap and its surrounding tissues are severed. Microcirculation undergoes a reorganization to allow adequate blood inflow and outflow; this process lasts up to 4 weeks. The sequence for pedicle flaps can be described as follows40:
0–24 hours: The immediate response of the microcirculation is vasospasm attributed to a local axon reflex phenomenon and hyperemia caused by hypoxia and loss of sympathetic control. The overall effect is a decrease in blood flow. There is a decrease in circulation during the initial 6 hours, reaching a plateau at 6–12 hours postharvest, followed by a progressive increase at 12 hours postharvest. Congestion and edema are frequently observed during this post-surgical period.
1–3 days: An improvement in pulse amplitude is noted when spontaneous vascular tone returns around the third day. There is an increase in the number and caliber of longitudinal anastomoses as well as the number of small vessels in the pedicle. It is imperative for flap survival to avoid shear and congestion during this period.
3–7 days: Circulation increases progressively with a plateau on day 7. Anastomoses noted earlier become significantly functional at 5–7 days, and there is an increase in both vessel size and number.
1 week: Blood circulation is well established between pedicle and recipient bed. Pulse amplitude approaches preoperative levels.
7–14 days: No further significant increase in vascularization occurs and maximum inflow is achieved. Circulation efficiency is superior to preoperative levels during the period of 10–21 days.
2 weeks: There is a progressive regression of the vascular system. Maturation of the new anastomoses between flap and recipient bed takes place.
3 weeks: The vascular pattern is similar to preoperative levels. Vascular connections between the pedicle flap and the recipient bed are fully developed.
4 weeks: There is a decrease in diameter of all vessels, including preexisting vessels. Circulation efficiency is normal and skin color becomes more normal without the increased vascularity.
The sequence that follows a free flap harvest and anastomosis is similar but retains a major distinction. When blood vessels are sectioned, they lose the direct nervous regulation by sympathetic and parasympathetic fibers. However, the re-establishment of circulation between the flap and its recipient bed shares similitudes.
Local blood flow in the microcirculation is regulated by multiple factors, each one ultimately causing vasoconstriction or vasodilation. The summation of these opposing forces eventually determines the final local blood flow. In the case of a flap in which microvascular rearrangements are multiple secondary to flap dissection, these factors are of paramount importance. They must be carefully evaluated and controlled to allow flap survival. These factors can be classified as being regulated systemically or locally.
Systemic regulation has two components: neural regulation and humoral regulation. Neural regulation is the dominant effect of systemic regulation and is mostly via sympathetic adrenergic fibers. Vasoconstriction is induced by α-adrenergic receptors while vasodilation is the result of β-adrenergic receptors. Other fibers have also been implicated in the overall vascular tone. Serotoninergic fibers induce vasoconstriction while parasympathetic cholinergic fibers, via muscarinic receptors, cause vasodilation. The combination of all these forces ultimately determines the neural basal tone of vascular smooth muscle fibers in blood vessel.41
Humoral regulation is controlled by circulating hormones. The most important are epinephrine and norepinephrine acting on α-adrenergic receptors, thereby causing vasoconstriction. Serotonin, thromboxane A2, and prostaglandin F2α also cause vasoconstriction. Substances causing vasodilation are prostaglandin E1, prostaglandin I2, histamine, bradykinin, and leukotrienes C4 and D4.41
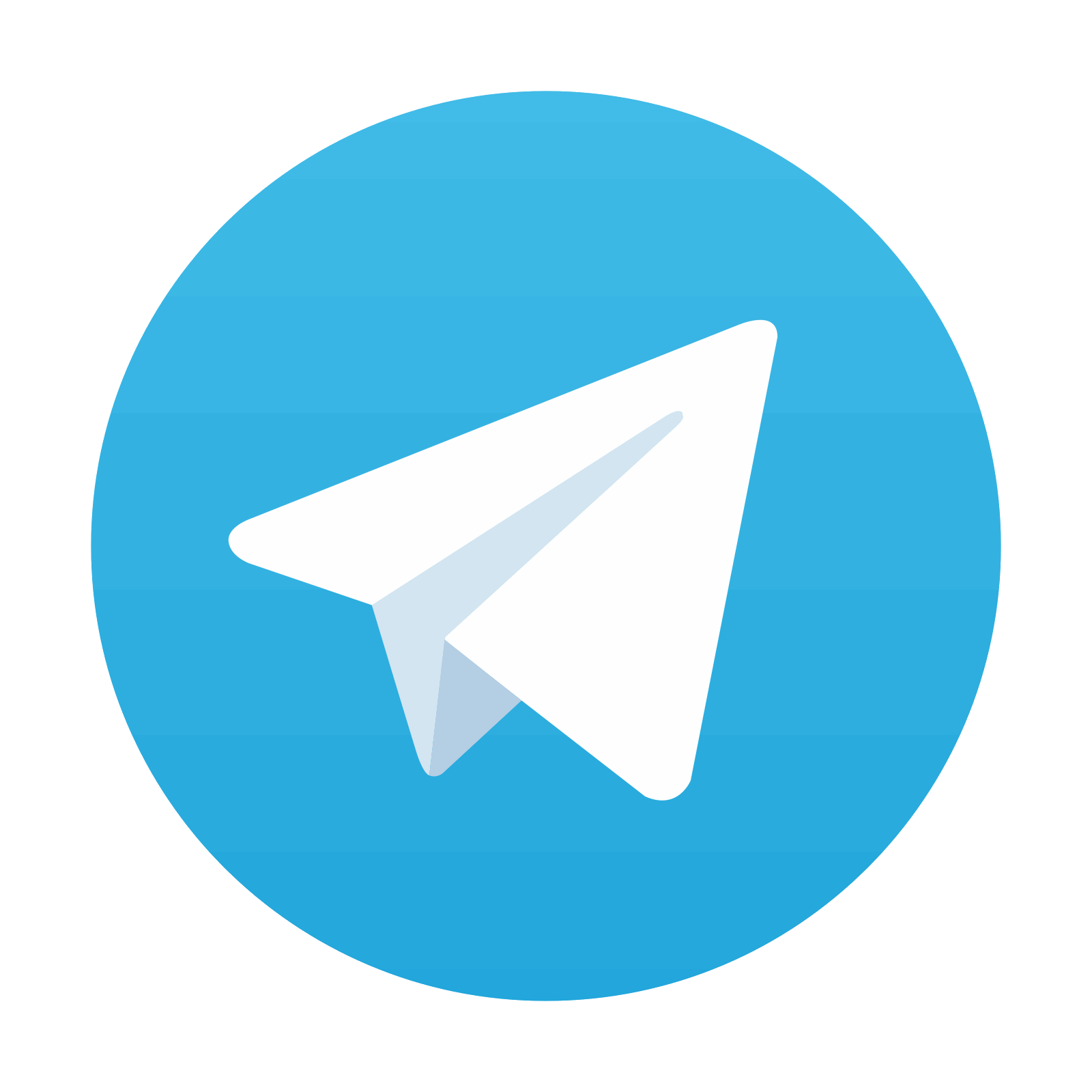
Stay updated, free articles. Join our Telegram channel

Full access? Get Clinical Tree
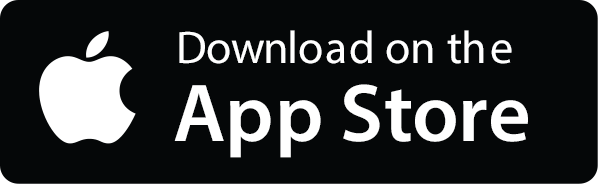
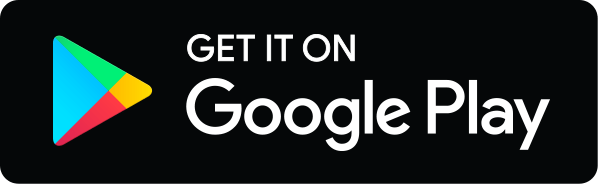
