Evaluation of the Patient with Suspected Neuromuscular Junction Disorder
William J. Litchy
Introduction
The evaluation of the patient with a neuromuscular junction (NMJ) disorder has evolved over recent years. Increased understanding of the structure and function of the NMJ; the advent of new and improved electrophysiologic techniques, including patch clamp and endplate noise analysis; the increased understanding of the immunologic characteristics of the NMJ; and the genetic studies that are identifying increasing numbers of inherited NMJ disorders have changed our perspective on this group of disorders. At the same time that more NMJ disorders have been identified and characterized, treatment options have increased, and the complexity of the evaluation of the person suspected of having a NMJ disorder is greater.
Since Jolly, in 1895, described the physiologic evaluation of the patient with myasthenia gravis, there have been advances in the armamentarium for the electrodiagnostic medicine physician (1). Improved knowledge of the clinical syndromes associated with neuromuscular disorders has been helpful. Although weakness, particularly fluctuating weakness, is the hallmark of NMJ disorders, further delineation of the symptoms and signs of these disorders has been important. Increasing knowledge of the structure and function of the NMJ has been paramount for furthering the understanding of NMJ disorders; in this case the receptors and function have been important. Advances in immunology and in the knowledge of the receptors in the NMJ have revolutionized both the understanding of the pathologic mechanisms of the disease and the diagnostic evaluation of these disorders. The ability to evaluate the genetic aspects of the NMJ diseases has also led to many advances. Not only do we now recognize a large variety of congenital myasthenic syndromes, but we can also describe the ion channels or membrane receptor impairments and locate the genetic defect(s) causing the disorder (2,3,4).
The electrodiagnostic medicine physician’s tools have also improved and, when combined with the clinical, immunologic, and genetic information, it is possible to interpret the electrophysiologic findings in a more clinically meaningful manner. The basic tools of the electrodiagnostic physician are nerve conduction studies and needle electromyography. The most sensitive nerve conduction test for the evaluation of a weak patient with suspected NMJ disease is repetitive nerve stimulation. In myasthenia gravis, the most common disorder of the NMJ, abnormalities of repetitive nerve stimulation (RNS) studies may be seen in up to 90% of people. The specific pattern of abnormal, decremental serial responses in these patients is often characteristic of the disease. Needle electromyography (EMG), particularly single-fiber EMG, is also very useful in the evaluation of the patient. It is a very sensitive test but not specific for disorders of the NMJ (5,6). Abnormalities can be observed in disorders of the motor neuron and
peripheral nerve as well as the muscle fiber that may be indistinguishable from the observations in myography (7,8).
peripheral nerve as well as the muscle fiber that may be indistinguishable from the observations in myography (7,8).
The scope of this chapter is to review the approaches to the evaluation of the patient with a suspected NMJ disorder in the clinical neurophysiology laboratory. The emphasis will be on the tools used in the electrodiagnostic laboratory. To understand the value of these tools, a brief discussion of the structure and function of the NMJ is included. This will be followed by a discussion of techniques and approaches to their use. Finally, observations on some of more commonly encountered disorders of neuromuscular transmission (NMT) will be presented.
Neuromuscular Junction
Structure
The NMJ is a specialized synapse anatomically and functionally designed to activate muscle fibers in a controlled manner located at a specialized area of the muscle membrane called the endplate. The NMJ is composed of presynaptic and postsynaptic membranes separated by the synaptic cleft. The presynaptic region is the termination of the motor axon at the endplate, while the postsynaptic region is the specialized region of the muscle fiber membrane with multiple junctional folds. Structural and functional abnormalities of the NMJ result in numerous clinical disorders, the hallmark of which are fluctuating weakness and fatigability (9) (Fig. 15-1).
Presynaptic Terminal
The presynaptic terminal has all of the machinery required to release the neurotransmitter acetylcholine (ACh): mitochondria; synaptic vesicles, each containing 5,000 to 10,000 ACh molecules; and a presynaptic membrane that includes active zones and sodium, potassium, and calcium ion channels involved in the local depolarization and subsequent release of synaptic vesicles into the synaptic cleft (10,11,12,13). The synaptic vesicles cluster at the active zones in preparation for release into the synaptic cleft. During the depolarization phase of the presynaptic region, ionic calcium enters the presynaptic terminal. The half-life for internal Ca2+ concentration is approximately 500 ms. The rise in intracellular calcium results in the vesicular fusion with the presynaptic membrane and release of ACh into the synaptic cleft (14).
Synaptic Cleft
The synaptic cleft, a space between the pre- and post-synaptic membranes, is the site where endplate-specific acetylcholinesterase (AChE) is located on the synaptic basal lamina. The role of AChE is to inactivate ACh and maintain a steady-state function of the NMJ.
Postsynaptic Cleft
The postsynaptic membrane is composed of the specialized region of the muscle membrane formed into junctional folds with ACh receptors (AChR) clustered at the tops of the folds at a density of 1,000/um+. At the base of the junctional folds are voltage-gated Nav1.4 channels. The distribution of the AChRs and the Nav1.4 into separate areas of the junctional fold is ideal for the normal function of the NMJ. Activation of these AChRs by acetylcholine, usually two molecules per receptor, results in a membrane depolarization and activation of Nav1.4 channels. When this depolarization is sufficiently large, it produces an action potential and a contraction of the muscle fibers (15).
Function
Under the resting state there is a steady random release of ACh-filled synaptic vesicles. The ACh molecules attach to the AChR and generate miniature endplate potentials (MEPPs) (16,17,18). The MEPP is the result of the action of one or a few synaptic vesicles. A nerve action potential depolarizing the nerve terminal results in an influx of calcium ions into the presynaptic terminal through voltage-gated calcium channels, causing a fusion of the synaptic vesicles with the presynaptic membrane and release of a large amount of ACh into the synaptic cleft. The influx of calcium ions results in an increased probability of additional release of ACh for a short time (18).
The depolarization the presynaptic membrane by an action potential results in the release of
many synaptic vesicles and produces an endplate potential (16,18). When the endplate potentials exceed the threshold for activating the Nav1.4 channels, a muscle fiber action potential occurs. The high concentrations of the AChRs at the top of the functional fold and the Nav1.4 channels located at the base of the junctional fold are essential for normal propagation of the action potential and contraction of the muscle fiber. The difference between the depolarization caused by the AChR activation and the depolarization required to activate the Nav1.4 is the safety factor. When the activation of the AChRs is below the safety factor, the depolarization required to activate the muscles will not occur. All clinical disorders of NMT, whether acquired or congenital, are the result of the endplate potential being inadequate to activate the Nav1.4 or else the Nav1.4 channels not responding to normal endplate potentials (19).
many synaptic vesicles and produces an endplate potential (16,18). When the endplate potentials exceed the threshold for activating the Nav1.4 channels, a muscle fiber action potential occurs. The high concentrations of the AChRs at the top of the functional fold and the Nav1.4 channels located at the base of the junctional fold are essential for normal propagation of the action potential and contraction of the muscle fiber. The difference between the depolarization caused by the AChR activation and the depolarization required to activate the Nav1.4 is the safety factor. When the activation of the AChRs is below the safety factor, the depolarization required to activate the muscles will not occur. All clinical disorders of NMT, whether acquired or congenital, are the result of the endplate potential being inadequate to activate the Nav1.4 or else the Nav1.4 channels not responding to normal endplate potentials (19).
Electrophysiologic Studies
Electrophysiologic techniques are used to confirm the clinical suspicion of the disorder of NMJ and to exclude other disorders in patients with similar symptoms. The electrophysiologic studies include routine nerve conduction studies, needle EMG, RNS, and single-fiber EMG. Although electrodiagnostic studies can be very useful in the diagnosis of a NMJ disorder, and in some cases following the course of treatment intervention, the absence of
electrodiagnostic abnormalities does not preclude a diagnosis of a NMJ disorder. In autoimmune myasthenia gravis, for example, 10% of the patients may not have abnormal results on electrophysiologic studies. This percentage is even greater for patients in early stages of the disorder or those with primarily ocular myasthenia gravis.
electrodiagnostic abnormalities does not preclude a diagnosis of a NMJ disorder. In autoimmune myasthenia gravis, for example, 10% of the patients may not have abnormal results on electrophysiologic studies. This percentage is even greater for patients in early stages of the disorder or those with primarily ocular myasthenia gravis.
The most frequently used tests in the clinical neurophysiology laboratory are repetitive stimulation of a motor nerve with recording from its muscle (RNS) and single-fiber EMG. These tests measure the function of the NMJ indirectly. The most direct method to study the NMJ is with endplate analysis techniques. Although in vitro endplate analysis is clearly the most sensitive and reliable approach to evaluating the patient with a suspected NMJ disorder, it requires a muscle biopsy and a sophisticated analysis. RNS and single-fiber EMG are more practical, and when combined with the available immunologic studies, most patients with NMJ disorders can be evaluated adequately (20).
Repetitive Nerve Stimulation
RNS is the most frequently used test in the clinical neurophysiology laboratory to evaluate patients with a suspected disorder of the NMJ. Although not as sensitive as single-fiber EMG, it is specific when an abnormality is found. RNS is a straightforward test, but when used incorrectly it can lead to misdiagnosis or to no diagnosis when a disease is present. The physiologic characteristics of the NMJ and the potential for technical errors must always be considered when evaluating a patient with suspected NMJ disorders in the clinical neurophysiology laboratory.
The RNS evaluation of the patient with a suspected NMJ disorder requires preparation of the patient, use of the appropriate equipment, and studying the correct muscles with the appropriate stimulation protocols. Although the technique of RNS stimulation is straightforward, there are numerous physiologic and technical factors that affect test results.
Patient Preparation
For the successful evaluation with RNS, the patient should be properly prepared for the study. This preparation includes instructing the patient in what is expected. The limb must be warm to avoid unreliable and incorrect results. Also, the patient should not be taking any medication interfering with the test; specifically, the patient should not take any anticholinesterase medications prior to the study, such as pyridostigmine.
Muscles Studied
It is important to study the correct muscles when doing RNS studies. The criteria for selecting the nerve and muscle combination are no different than choosing a nerve or muscle for any other electrodiagnostic test. The muscle studies should be relevant. There should be a suspicion that it is clinically involved or is useful in excluding a disorder of the NMJ or other disease. The muscle should be reliable in that the information one obtains from the study reflects the function of the NMJ. The results obtained should be reproducible. If the results are not similar each time the study is performed, it is difficult to interpret the results. The test should be relatively comfortable. RNS is usually uncomfortable, so it is helpful to choose a muscle that you are used to testing and that may be less painful. The muscles most commonly studied are listed in Table 15-1, along with the nerve stimulated and the advantages and disadvantages of studying the muscle (21,22).
Stimulation Protocols
Before performing repetitive stimulation on a nerve, it is important to perform routine motor and sensory nerve conduction studies to exclude other disorders. A nerve or muscle affected by another pathophysiologic process is unreliable, and abnormalities observed on RNS are difficult to interpret. RNS is used to demonstrate abnormal function of the NMJ. Based on the knowledge of the physiology and the results observed, there are reasonable approaches to stimulating the nerve innervations of the muscle.
Most frequently, repetitive stimulation is performed by stimulating at 2 Hz in a train of four stimuli. Longer trains of stimuli, up to 10, and a higher frequency, 3 Hz, are used in some clinical neurophysiology laboratories. However, abnormalities, when present, are observed with a train of four stimuli and a lower stimulation frequency under most circumstances. Shorter stimulus trains help to reduce the artifact associated with movement.
Three sets of four stimuli are sometimes performed to ensure that the recordings are reliable and reproducible. These baseline recordings are used to compare with subsequent studies.
Three sets of four stimuli are sometimes performed to ensure that the recordings are reliable and reproducible. These baseline recordings are used to compare with subsequent studies.
Table 15-1 Nerves and Muscles Commonly Used in the Evaluation of Patients with Suspected Neuromuscular Junction Disorders | ||||||||||||||||||||||||||||||||||||||||||||||||||||||||||||||||||
---|---|---|---|---|---|---|---|---|---|---|---|---|---|---|---|---|---|---|---|---|---|---|---|---|---|---|---|---|---|---|---|---|---|---|---|---|---|---|---|---|---|---|---|---|---|---|---|---|---|---|---|---|---|---|---|---|---|---|---|---|---|---|---|---|---|---|
|
Exercising the muscle studied is a valuable way to accentuate an abnormality or to observe one that is not present in the baseline recordings. If there is an abnormality, brief exercise may repair the decrement, and this is another characteristic feature of a disorder of the NMJ. In a presynaptic NMJ disorder such as Lambert-Eaton myasthenic syndrome, brief exercise may produce facilitation of the response, which, if large enough, may be diagnostic of this disorder (see later section in this chapter).
Abnormalities on Repetitive Nerve Stimulation
The typical abnormalities observed in NMJ disorders are decrement, repair of the decrement, postactivation exhaustion, and facilitation. A decrement of up to 8% has been reported in patients without NMJ disorders. In autoimmune myasthenia gravis, a minimal decrement of 10% should be present in more then one muscle, and that decrement should repair immediately following exercise (20). The size of the decrement varies in the muscles studied in the same patient, reflecting the variation in the NMJ pathology (Fig. 15-2).
Decrement
Decrement is the change in the amplitude (or area) of the fourth compound muscle action potential (CMAP) compared to the first CMAP in the train. Decrement is expressed as a percent reduction from the first response in the stimulus train (Fig. 15-3). Although there can be a small decrement in normal people, a reproducible decrement of more then 10% is considered abnormal. Besides the amount of decrement, the pattern of the decrement is important in identifying the specific cause of the NMJ disorder. In autoimmune myasthenia gravis, the largest decrement is between the first and second response (see Fig. 15-3). In other disorders of the NMJ, this may not be the case. Although this pattern is seen in other diseases, a different pattern is due to either technical problems or other disorders. The amount of the decrement is also affected by exercise, the time after exercise when a train of stimuli is given, and the particular muscle examined (20).
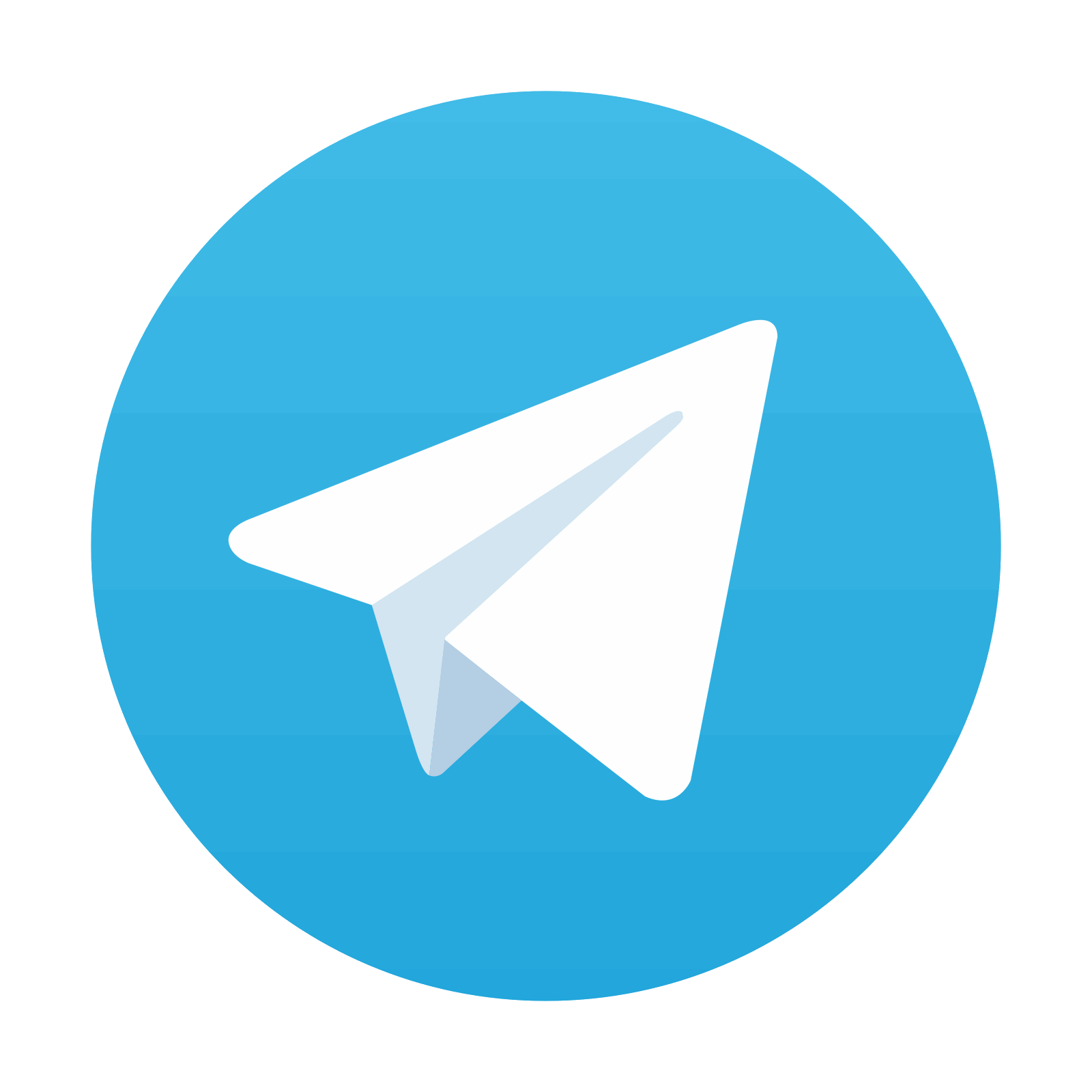
Stay updated, free articles. Join our Telegram channel

Full access? Get Clinical Tree
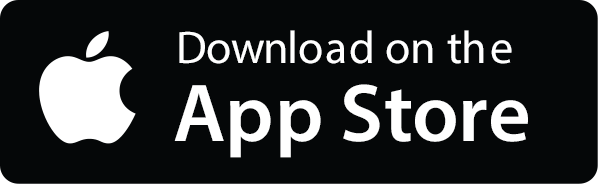
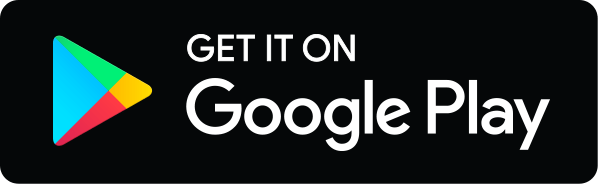
