Introduction
Skeletal stability after bimaxillary surgical correction of Class III malocclusion was investigated through a qualitative and quantitative analysis of the maxilla and the distal and proximal mandibular segments using a 3-dimensional voxel-based superimposition among virtual surgical predictions performed by the orthodontist in close communication with the maxillofacial surgeon and 12-18 months postoperative outcomes.
Methods
A comprehensive secondary data analysis was conducted on deidentified preoperative (1 month before surgery [T1]) and 12-18 months postoperative (midterm [T2]) cone-beam computed tomography scans, along with virtual surgical planning (VSP) data obtained by Dolphin Imaging software. The sample for the study consisted of 17 patients (mean age, 24.8 ± 3.5 years). Using 3D Slicer software, automated tools based on deep-learning approaches were used for cone-beam computed tomography orientation, registration, bone segmentation, and landmark identification. Colormaps were generated for qualitative analysis, whereas linear and angular differences between the planned (T1-VSP) and observed (T1-T2) outcomes were calculated for quantitative assessments. Statistical analysis was conducted with a significance level of α = 0.05.
Results
The midterm surgical outcomes revealed a slight but significantly less maxillary advancement compared with the planned position (mean difference, 1.84 ± 1.50 mm; P = 0.004). The repositioning of the mandibular distal segment was stable, with insignificant differences in linear (T1-VSP, 1.01 ± 3.66 mm; T1-T2, 0.32 ± 4.17 mm) and angular (T1-VSP, 1.53° ± 1.60°; T1-T2, 1.54° ± 1.50°) displacements ( P >0.05). The proximal segments exhibited lateral displacement within 1.5° for both the mandibular right and left ramus at T1-VSP and T1-T2 ( P >0.05).
Conclusions
The analysis of fully digital planned and surgically repositioned maxilla and mandible revealed excellent precision. In the midterm surgical outcomes of maxillary advancement, a minor deviation from the planned anterior movement was observed.
Highlights
- •
Three-dimensional analysis used automated protocol with convolutional neural networks.
- •
Excellent maxilla and mandible repositioning was observed.
- •
At 12-18 months, maxillary advancement is slightly less than planned.
- •
The collaborative synergy of surgeon and orthodontist maximizes outcomes.
The primary goal of orthognathic surgery is to correct severe dentofacial deformities, achieving both esthetic and functional improvements. Traditionally, changes resulting from orthognathic surgery have been analyzed by comparing presurgical and postsurgical 2-dimensional (2D) radiologic images, focusing on skeletal and soft-tissue changes. , However, advancements in computer-assisted imaging analysis have made low-dose cone-beam computed tomography (CBCT) available, allowing for 3-dimensional (3D) assessment of orthognathic patients. Compared with 2D images, CBCT provides more detailed information for surgeons, enabling accurate evaluations of both skeletal and soft tissue and reducing the risk of intraoperative errors. , , The advent of 3D virtual surgical planning (VSP) enhanced preoperative analysis and eliminated many of the laboratory steps involved in surgical splint preparation. , Accurate VSP represents a remarkable challenge for orthodontists and orthognathic surgeons, as the virtual plan correction requires collaborative interdisciplinary communication, anticipation of surgical difficulties and limitations, and visual evaluation of the simulated results on both skeletal and soft tissues. , The success of orthognathic surgery relies not only on surgical procedures but also on a precise diagnosis and a well-defined treatment plan, aiming for predetermined results, skeletal stability, and long-term soft-tissue harmony during follow-up.
For patients with Class III malocclusion, previous studies have observed changes in the mandibular condyles and rami 1 week postsurgery, as well as significant postoperative adaptations 1-year postsurgery. , Given that VSP represents a substantial improvement in orthognathic surgical management, it is crucial to analyze the reliability of this protocol by evaluating postoperative outcomes. Although several studies have reported the accuracy of VSP in the early postoperative months, there is a paucity of data regarding midterm postoperative displacement and remodeling of the jaws after virtually planned orthognathic surgery using an automated voxel-based workflow for 3D imaging analysis. By employing specific mathematical algorithms, a more recent method known as voxel-based registration ensures a high level of accuracy in automated image registration. , A comprehensive 3D evaluation of orthognathic patients using a fully digital virtual planning protocol can provide valuable insights into postsurgical displacements of the jaws, quantification of changes, and assessment of their direction. Recognizing the potential for postsurgical skeletal changes and the influence of postsurgical orthodontic movements on skeletal stability, active orthodontist involvement in the virtual planning process is crucial to ensure accurate and stable outcomes.
The objective of this study was to evaluate postsurgical skeletal stability after 12-18 months of follow-up in patients with severe dentoskeletal Class III malocclusions treated with bimaxillary orthognathic surgery after VSP performed by a trained orthodontist in close communication with the oral and maxillofacial surgeon. A 3D workflow based on an automated deep-learning approach was used to assess qualitative and quantitative morphometric changes in the position of the maxillary bone, as well as the distal and proximal segments of the mandible, by comparing preoperative and postoperative CBCT scans with the VSP.
Material and methods
A secondary analysis of preexisting data prospectively collected for clinical purposes was performed. The medical protocol and ethics followed the Declaration of Helsinki. The Institutional Review Board approved the study (HUM00224130 – reference for the University of Michigan, Ann Arbor, Mich). To use radiologic data for scientific analysis, a specific informed consent form was signed by all patients.
Deidentified CBCT scans (field of view of 17 × 20 cm; 400 µm; 110 kVp; 3.175 s; 59 mSV) from 17 nongrowing patients (9 females and 8 males), aged 18-32 years (mean age, 24.8 ± 3.5 years), with a Class III dentoskeletal malocclusion (A-point–Nasion-B-point angle <0°; unilateral or bilateral Angle Class III molar relationship), who underwent bimaxillary orthognathic surgery with traditional approach and preoperative VSP, were included. The VSP was conducted by an orthodontist in close communication with the surgeon using Dolphin Imaging software (Dolphin Imaging and Management Solutions, Chatsworth, Calif). After acquiring the presurgical CBCT scans, clinical records, and photographs, the orthodontist and maxillofacial surgeon discussed the treatment goals, including surgical, esthetic, and occlusal corrections. The orthodontist then conducted the VSP. This plan was presented to the surgeon for approval, with revisions made if necessary to align orthodontic and surgical objectives. Orthognathic surgery was performed using computer-aided design and manufacturing intraoperative splints for jaw repositioning. The CBCT scans were collected up to May 2023 and stored in the Dropbox folder at the laboratory-protected server at the University of Michigan, School of Dentistry. Patients with 1-jaw surgery, surgery-first approach, history of mandibular trauma, craniofacial syndromic malformations, systemic diseases, or osteometabolic disorders were excluded.
Preoperative (T1) CBCT scans, performed 1 month before surgery, and postoperative (T2) CBCT scans taken at the midterm period, 12-18 months after surgery, were analyzed and compared with the VSP. The virtual simulation was performed by a trained orthodontist according to the objectives discussed with the maxillofacial surgeon to determine the jaw movements in all spatial directions ( Fig 1 ). The resulting data were then exported as stereolithography files. Three-dimensional image analysis was conducted using the software 3D Slicer, using dedicated tools on the basis of recently validated deep-learning approaches. A standardized orientation of all T1 CBCTs was performed according to the Frankfurt plane and the midsagittal plane. , Automated voxel-based registration of postoperative CBCTs on oriented T1 scans was performed for each patient, selecting the cranial base as the reference area of superimposition because it is not modified by the surgery. Automated bone segmentation allowed us to obtain the virtual models of each skeletal segment (cranial base, maxilla, and mandible). Quality control of the segmentations was performed using the ITK-SNAP software (version 3.8.0; http://www.itksnap.org ). Automated landmarks identification was performed; landmark position was then checked by an experienced orthodontist ( Table I ).

Landmarks | Abbreviation | Definition | Abbreviation of bilateral landmarks |
---|---|---|---|
Maxilla | |||
Orbitalis | Or | The most inferior point on the lower portion of the orbit contour | Right side: ROr; left side: LOr |
Medial zygomatic point | MZyg | The deepest portion of the maxillary zygomatic process curvature | Right side: RMZyg; left side: LMZyg |
A-point | A | The most posterior point of the anterior concavity of the maxilla | – |
Mandible | |||
B-point | B | The most posterior point of the anterior concavity of the mental symphysis | – |
Pogonion | Pog | The most anterior point of the mentonian symphysis | – |
Menton | Me | The most inferior point of the mentonian symphysis | – |
Condylion | Co | The most superior point of the condyle contour | Right side: RCo; left side: LCo |
Medial condylar pole | MCo | The most medial and central point of the condyle | Right side: RMCo; left side: LMCo |
Lateral condylar pole | LCo | The most lateral and central point of the condyle | Right side: RLCo; left side: LLCo |
Gonion | Go | The most inferior and posterior point of the mandibular angle | Right side: RGo; left side: LGo |
For the qualitative analysis, automated surface registration was performed to superimpose the skeletal models of VSP and T2. Shape analyses were conducted by subtracting the point-based models of VSP and T2, allowing for better visualization of changes that occurred in the 3 spatial axes (x, y, and z). Surgical outcomes were highlighted using semitransparent overlays and colormaps generated automatically by the software. By adjusting the surface distance values on the color bar, the interpretation of the distance maps enhanced the understanding of the magnitude of positional changes between the models, with excess or deficit movements being correlated with positive and negative numbers, respectively.
For the quantitative analysis, the dedicated tool “automatic quantification of 3D components (AQ3DC)” was used to calculate linear measurements in millimeters along the 3 coordinates: anteroposterior (x-axis), upper-lower (y-axis), and right-left (z-axis). Root mean square was used to calculate the 3D displacement. In addition, angular measurements in degrees were obtained for the 3 components: yaw, pitch, and roll. The focus of the analysis was on comparing T1-VSP and T1-T2 data. To assess the accuracy of mandibular and maxillary movements relative to the cranial base region of reference, displacements within 2 mm for linear measurements and within 4° for angular measurements were considered clinically acceptable criteria.
Statistical analysis was performed using the R (version 4.3; R Core Team, Vienna, Austria). Intrarater reliability was preliminarily determined using the intraclass correlation coefficient for all measurements. A pilot study was conducted to determine the sample size needed for comparing 2 paired means. A total of 15 patients would be required, considering the following parameters: the difference in means (μ1 − μ2 = 1.47), the standard deviation (SD = 1.85), the significance level (α = 0.05), and the statistical power (β = 0.8). To evaluate the normality of each distribution, the Kolmogorov-Smirnov test was calculated. Descriptive statistics recorded absolute and relative frequencies for categorical data, mean and standard deviation for continuous quantitative variables with normal distribution and median and interquartile range for asymmetric distributions, and 95% confidence interval for a difference between 2 means. Bivariate analysis was performed using a 2-tailed Student t test for normal distributions and Wilcoxon and Mann-Whitney U tests for asymmetrical distributions. The level of significance was set at α = 0.05.
Results
Details of the study sample are presented in Table II . The mean duration of postsurgical follow-up was 15.40 ± 2.92 months. The intraclass correlation coefficient for all measurements exceeded 0.87, indicating high reliability. Figure 2 provides a qualitative analysis, displaying semitransparent overlays and color-coded maps of a representative patient to illustrate the findings. Tables III-V compare the results between T1-VSP (planned position) and T1-T2 (observed position), summarizing the linear and angular displacements, respectively. Table VI reports the cephalometric skeletal measurements at T1 (presurgical), T2 (postsurgical), and VSP (planned).
Demographic variables | Study sample |
---|---|
Patients | 17 |
Sex | |
Female | 9 (53%) |
Age (y) | 24.8 ± 3.5 |
Sagittal skeletal diagnosis | |
Class III malocclusion | 17 (100%) |
Skeletal asymmetry | 9 (53%) |
Mean follow-up (mo) | 15.40 ± 2.92 |

Linear displacement (mm) | Group | ||
---|---|---|---|
Landmarks | T1-VSP | T1-T2 | P value |
Maxilla | |||
A-point | |||
AP | 4.94 ± 1.88 | 3.10 ± 1.60 | 0.004 ∗ |
SI | 0.53 (−2.50 to 5.96) | 0.20 (−1.97 to 6.25) | 0.918 |
3D | 5.54 ± 1.85 | 4.01 ± 1.86 | 0.020 ∗ |
MidZyg | |||
RL | −0.20 ± 1.16 | −0.11 ± 1.10 | 0.816 |
AP | 0.00 (−3.00 to 4.95) | 0.44 (−1.08 to 2.29) | 0.581 |
SI | −0.44 (−2.50 to 2.11) | −0.07 (−2.50 to 1.69) | 0.215 |
3D | 1.60 (0.40-5.55) | 1.46 (0.31-2.85) | 0.326 |
MidOr | |||
RL | −0.19 ± 0.89 | −0.25 ± 0.83 | 0.842 |
AP | 0.04 ± 1.14 | 0.02 ± 0.71 | 0.975 |
SI | 0.13 ± 0.64 | −0.11 ± 0.47 | 0.219 |
3D | 1.43 ± 0.61 | 1.11 ± 0.43 | 0.084 |
Mandible | |||
B-point | |||
RL | 0.26 ± 2.58 | 0.91 ± 3.11 | 0.510 |
AP | 1.01 ± 3.66 | 0.32 ± 4.17 | 0.611 |
SI | 2.39 (−2.45 to 14.96) | 2.58 (−1.14 to 11.9) | 0.389 |
3D | 5.15 (2.52-15.27) | 6.65 (2.56-12.45) | 0.143 |
Pog | |||
RL | −0.01 ± 2.88 | 0.99 ± 3.10 | 0.335 |
AP | 1.98 ± 4.77 | 1.89 ± 4.82 | 0.955 |
SI | 1.82 (−1.89 to 10.85) | 1.65 (−0.59 to 10.26) | 0.679 |
3D | 6.52 ± 3.49 | 6.75 ± 3.10 | 0.843 |
Me | |||
RL | −0.15 ± 3.12 | 0.84 ± 3.31 | 0.376 |
AP | 1.84 ± 4.70 | 2.29 ± 5.02 | 0.789 |
SI | 1.38 (−1.21 to 12.21) | 1.4 (−0.91 to 12.36) | 0.973 |
3D | 6.35 (1.46-14.94) | 7.47 (1.64-14.38) | 0.370 |
MidCo | |||
RL | −0.20 ± 0.88 | −0.25 ± 0.58 | 0.845 |
AP | −0.39 (−3.35 to 0.84) | −0.10 (−3.35 to 1.08) | 0.153 |
SI | −0.19 (−5.07 to 2.00) | −0.10 (−5.07 to 0.58) | 0.679 |
3D | 1.58 (0.48-6.18) | 0.97 (0.12-6.18) | 0.006 ∗ |
MidLCo | |||
RL | −0.06 ± 0.74 | −0.05 ± 0.55 | 0.973 |
AP | −0.48 (−4.25 to 0.71) | 0.28 (−4.25 to 1.51) | 0.020 ∗ |
SI | 0.04 (−2.07 to 3.92) | 0.01 (−2.07 to 1.19) | 0.705 |
3D | 1.57 (0.38-4.81) | 0.99 (0.28-4.81) | 0.174 |
MidMCo | |||
RL | 0.23 (−1.57 to 1.07) | −0.02 (−1.05 to 0.33) | 0.270 |
AP | 0.14 (−1.59 to 3.77) | −0.38 (−1.59 to 1.22) | 0.153 |
SI | −0.52 ± 1.65 | −0.76 ± 1.27 | 0.643 |
3D | 1.59 (0.33-4.50) | 0.91 (0.21-4.50) | 0.079 |
MidGo | |||
RL | 0.19 ± 1.19 | 0.43 ± 1.68 | 0.636 |
AP | 0.39 ± 1.75 | 0.55 ± 2.01 | 0.808 |
SI | −0.57 ± 1.33 | 0.45 ± 1.69 | 0.060 |
3D | 2.39 ± 0.87 | 3.04 ± 0.77 | 0.026 ∗ |
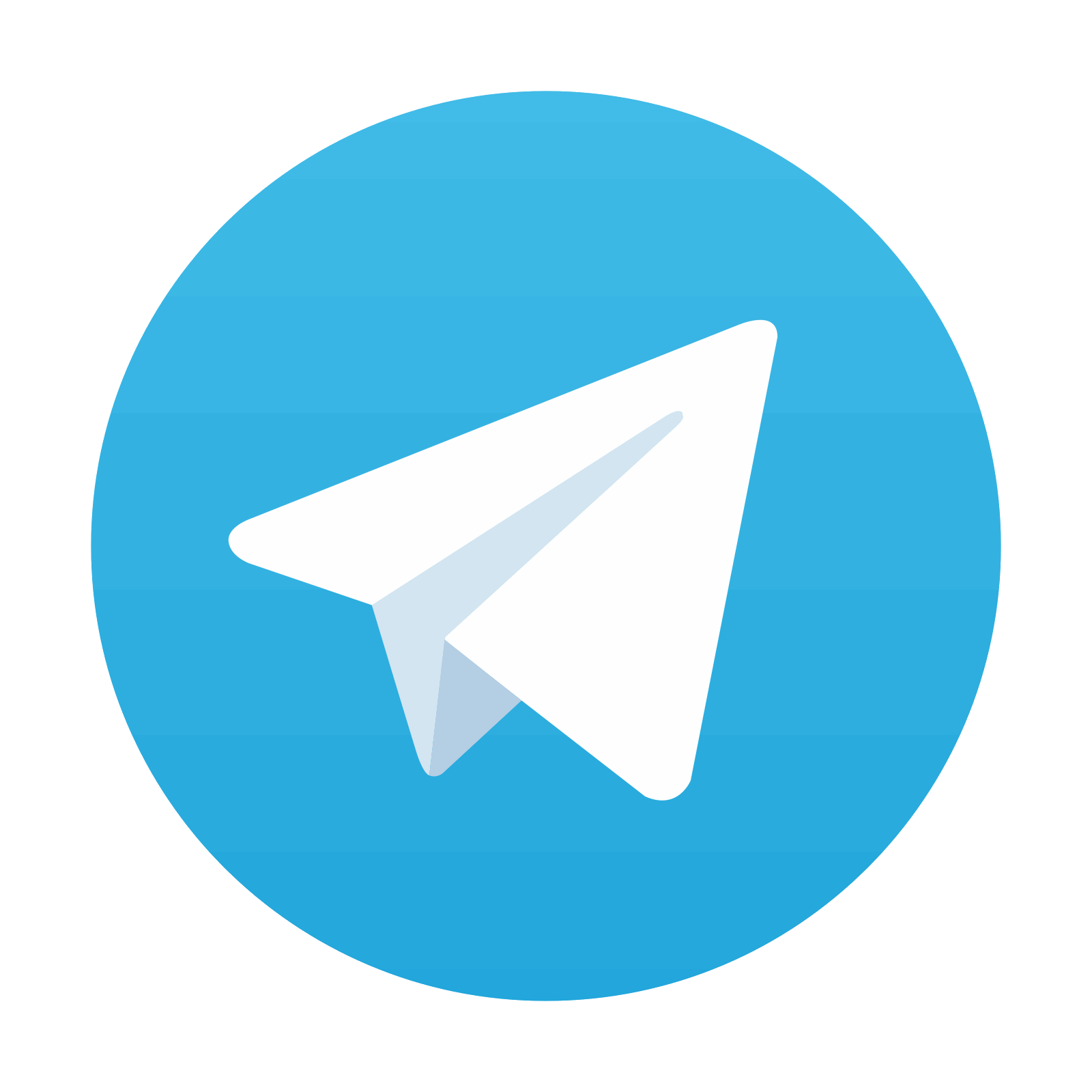
Stay updated, free articles. Join our Telegram channel

Full access? Get Clinical Tree
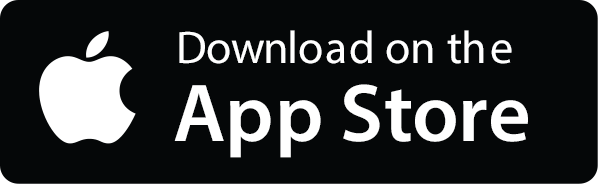
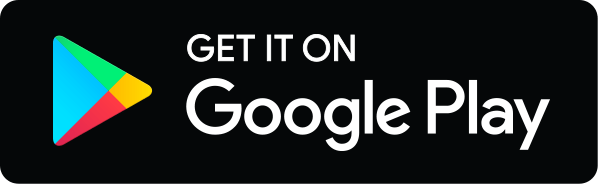
