units (MUs) that are firing. CMAPs are usually recorded in mV. For sensory nerves, the response is called an SNAP and represents the summation of individual sensory nerve fibers. SNAPs are very small-amplitude potentials that are usually recorded in μV. Late responses (evoked potentials that record over a very long pathway) include F waves and H-reflexes. Orthodromic refers to conduction in the same direction as occurs physiologically (i.e., a sensory fiber conducts from the extremity toward the spine). Antidromic refers to conduction in the opposite direction to the physiological direction.
of some of the supporting connective tissue stroma (Schwann cells and endoneurial tubes). Regeneration of axons (through collateral sprouting or axonal growth) can occur with good functional recovery, depending on the amount of axonal loss.
Uniform demyelination – slowing of CV along the entire nerve (e.g., Charcot-Marie-Tooth disease).
Segmental demyelination – uneven degree of demyelination in different areas along the course of the nerve; may have variable slowing (temporal dispersion).
Focal nerve slowing – localized area of demyelination causing nerve slowing; decreased CV is noted across the lesion.
Conduction block – severe focal demyelination that prevents propagation of the AP through the area. There will be more than 20% amplitude decrement when the nerve is stimulated proximal to the lesion. The distal CMAP amplitude remains intact. Clinically, conduction block presents as weakness.
criterion for abnormality. An abnormal latency greater than 0.5 to 1.0 ms (as compared with the other side) or H-reflex absence in patients under 60 years may suggest a lesion along the H-reflex pathway (afferent and/or efferent fibers). This may be due to an S1 radiculopathy. The standard formula for calculating the H-reflex is 9.14 + 0.46 (leg length in cm from the medial malleolus to the popliteal fossa) + 0.1 (age). For a patient older than 60 years, 1.8 ms will be added to the total calculated value.
TABLE 17-1 Basic Abnormal Patternsa | ||||||||||||||||||||||||
---|---|---|---|---|---|---|---|---|---|---|---|---|---|---|---|---|---|---|---|---|---|---|---|---|
|
wire in the center of the shaft that is the recording electrode. The concentric needle can register the voltage changes between the wire and the shaft. The pointed tip of the needle has an oval (beveled) shape. Since the exposed active recording electrode is on the beveled portion of the cannula, the concentric needle picks up from a 180° field. Therefore, it registers smaller amplitude (since it has a smaller recording area). A separate surface electrode serves as the ground.
Normal insertional activity only lasts a few hundred milliseconds and is due to muscle depolarization.
Increased insertional activity occurs due to denervation or cell membrane irritability and lasts >300 ms. There may be evidence of initially positive deflection waveforms that do not persist. If these positive waveforms are sustained and fire regularly, they are considered abnormal spontaneous potentials (see below).
Decreased insertional activity occurs when the needle is placed into atrophied muscle, fat, or edema and lasts <300 m/s.
electrically discrete areas of muscle) for insertional activity and activity at rest. The needle can be withdrawn almost to the skin and then redirected in a different direction, again stopping at three or four different depths. This can be repeated so that the needle examines about 12 to 16 discrete areas of the muscle (depending on the patient’s tolerance).
After insertion of the needle into normal muscle at rest, there should be electrical silence (Fig. 17-2A).
Normal muscle may also display end plate activity. This occurs after a needle is placed in the region of the NMJ or end plate. The needle should be moved out of the end plate, as the clinician cannot get reliable information about the muscle. Either of two waveforms may occur: miniature end plate potentials (MEPPs) or end plate potentials (EPPs). The patient may complain of increased pain. It is important to recognize these potentials so that they are not misinterpreted as abnormal spontaneous potentials.
MEPPs – Represent spontaneous release of single quantum of acetylcholine (ACh) at the presynaptic terminal that manifests as end plate noise (Fig. 17-2B).
EPPs or “end plate spikes” – Represent single muscle fiber depolarizations at the presynaptic terminal with resultant release of large amounts of ACh (Fig. 17-2C).
MEPPs and EPPs may or may not be present together (Fig. 17-2D).
Usually represents pathology (injury or denervation) that stems from a muscle or nerve. These spontaneous depolarizations have an abnormal morphology and firing pattern.
Examples of muscle fiber-generated spontaneous potentials: Fibs triphasic with initial positive (downward) deflection, PSWs biphasic with positive deflection, myotonic discharges, and complex repetitive discharges (CRDs).
Examples of neural-generated spontaneous potentials: Myokymic discharges, cramps, neuromyotonic discharges, tremors, fasciculations, and multiple MU potentials.
Fibs and PSWs appear 3 weeks or more after injury.
![]() Figure 17-3 Fibs and PSWs. Adapted from DeLisa JA, ed. Manual of Nerve Conduction Velocity and Clinical Neurophysiology. 3rd ed. Baltimore, MD: Raven Press; 1994. |
![]() Figure 17-4 Fasciculation potentials. Adapted from DeLisa JA, ed. Manual of Nerve Conduction Velocity and Clinical Neurophysiology. 3rd ed. Baltimore, MD: Raven Press; 1994. |
![]() Figure 17-5 CRDs. Adapted from DeLisa JA, ed. Manual of Nerve Conduction Velocity and Clinical Neurophysiology. 3rd ed. Baltimore, MD: Raven Press; 1994. |
![]() Figure 17-6 Myotonic discharges. Adapted from Goodgold J. Electrodiagnosis of Neuromuscular Diseases. 2nd ed. Baltimore, MD: Williams & Wilkins; 1977. |
TABLE 17-2 Abnormal Spontaneous Potentials | ||||||||||||||||||||||||||||||||||||||||||||||||||||||||||||||||||||||||||||||||
---|---|---|---|---|---|---|---|---|---|---|---|---|---|---|---|---|---|---|---|---|---|---|---|---|---|---|---|---|---|---|---|---|---|---|---|---|---|---|---|---|---|---|---|---|---|---|---|---|---|---|---|---|---|---|---|---|---|---|---|---|---|---|---|---|---|---|---|---|---|---|---|---|---|---|---|---|---|---|---|---|
|
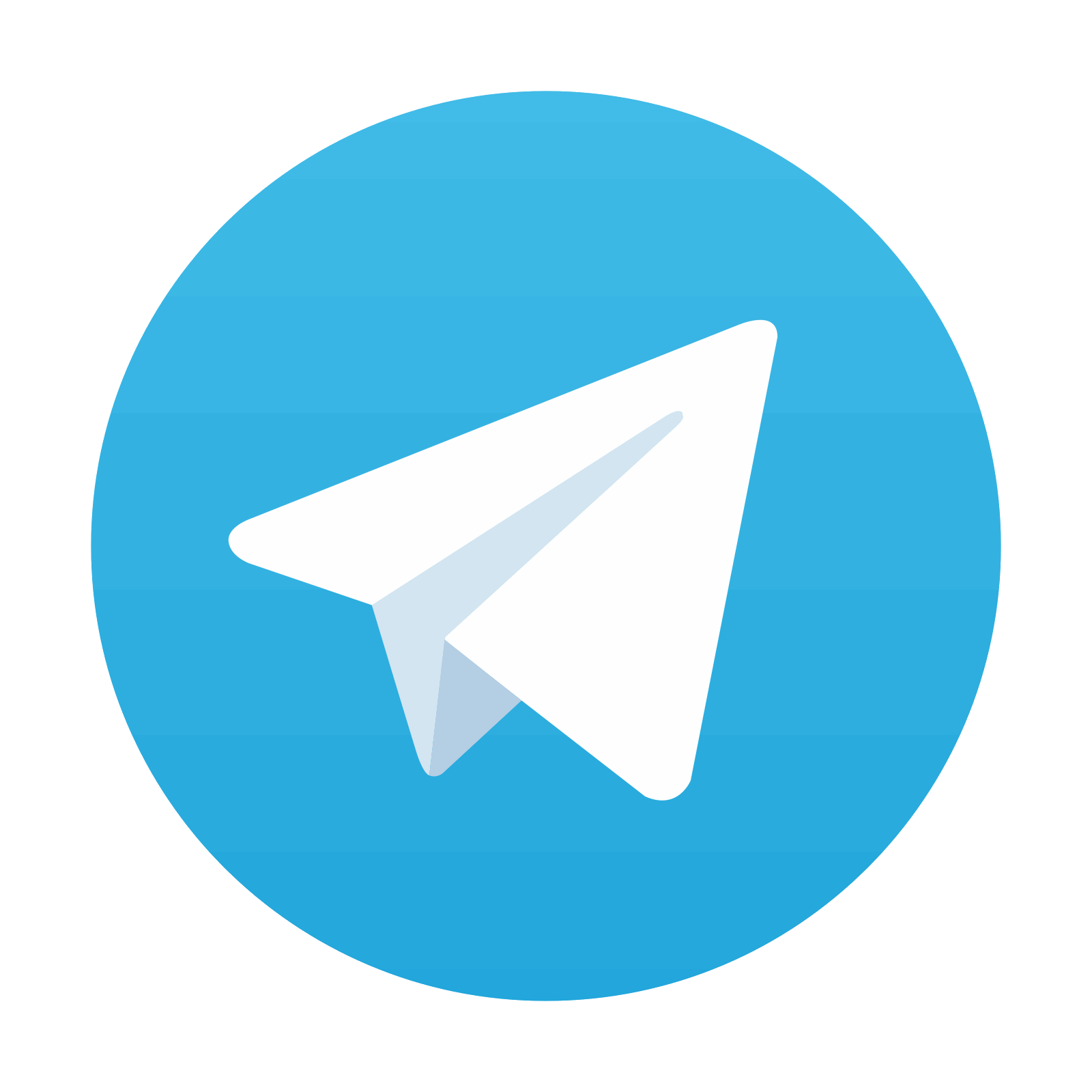
Stay updated, free articles. Join our Telegram channel

Full access? Get Clinical Tree
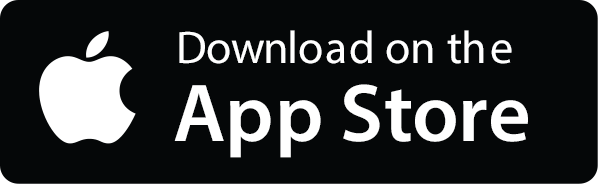
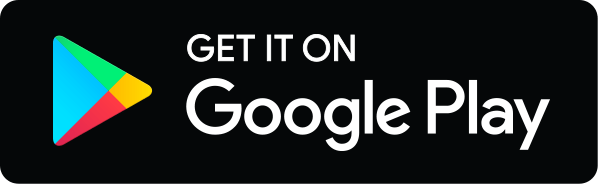