Electrodiagnostic medicine and electrophysiologic testing are an extension of the history and physical examination tailored to the clinical scenario. A wide range of tests are available and it is incumbent on the electrodiagnostician to select those relevant to the clinical circumstances. The testing procedure may change as data are acquired and new findings interpreted. High-quality consultations are rendered by physicians with experience and technical competence coupled with an understanding of the peripheral nervous system. This chapter provides an overview of electrodiagnostic tests, the extent of such testing for different clinical scenarios based on guidelines, and the types of abnormalities one can expect to find with different neuromuscular disorders.
Clinical Assessment: History and Physical Examination
History
A directed history enables the electrodiagnostician to develop a conceptual framework for subsequent testing. The history should include the referral reason, information as to whether symptoms are confined to a single limb or more than one limb, and the characteristic, nature of, the duration and time of onset, of symptoms. Pain and numbness indicate that the sensory system is involved. Motor weakness without pain and numbness indicates primarily involvement of the motor system, motor axons, neuromuscular junction, or muscles. For patients with more generalized symptoms—that is, symptoms in more than one limb—the electrodiagnostician should inquire about other neurologic complaints, such as dyspnea, bladder urgency, dysphagia, dysphonia, and visual changes. Previous cervical or lumbar spine conditions, such as spinal stenosis or surgical interventions, should be noted. Activities that aggravate or relieve a patient’s symptoms can often help differentiate musculoskeletal problems from entrapments, plexopathies, and radiculopathies. Hand numbness at night is associated with carpal tunnel syndrome.
Diabetes and alcohol are common causes of polyneuropathy in the United States, and an appropriate history should include questioning about such issues. Any family history of neurologic disorders is important to elicit for persons with generalized or unexplained symptoms. A brief recording of the person’s work activities is often helpful to identify persons at risk for overuse syndromes.
Important past medical history includes any history of malignancy, chemotherapy, or radiation, because these conditions and treatments can have peripheral neurologic implications. Previous surgeries for entrapment neuropathies, such as ulnar transposition or carpal tunnel release, should be ascertained. For instance, a transposed ulnar nerve complicates nerve conduction calculations for the segment across the elbow because measurement is less precise, and stimulus sites above and below the elbow can be more difficult to find. Whether a person is thrombocytopenic, has bleeding disorders, or is receiving anticoagulants should also be noted.
Physical Examination
Strength, sensation, and reflex testing are the most important aspects of the physical examination. Motor weakness is often seen in neuromuscular disorders. Subtle motor weakness can be elucidated by comparing sides and examining smaller muscles, such as the extensor hallucis longus or the hand intrinsic muscles. Reduced reflexes indicate peripheral nervous system problems, whereas increased reflexes suggest a central nervous system disorder, particularly when seen in combination with a Babinski sign, spasticity, and increased tone. An important caveat is that in acute spinal cord injury, there can be an initial reduction in reflexes because of spinal shock. Cranial nerve examination should be performed if stroke or another generalized condition is suspected.
An algorithmic approach to using physical examination and symptom information to tailor the electrodiagnostic evaluation is shown in Figure 8-1 . In this approach, the patient’s sensory complaints and physical examination signs of sensory loss and weakness create a conceptual framework for approaching these sometimes daunting problems. For the purposes of this discussion, generalized findings are defined as being present in two or more limbs. Although helpful, there are many exceptions to this taxonomy. The electrodiagnostician must refocus the diagnostic effort as data are acquired. Sensory loss is a key finding on examination for someone presenting with generalized symptoms, and provides a branch point in the algorithmic approach to tailoring the electrodiagnostic study (see Figure 8-1 ).

Myopathies, neuromuscular junction disorders, motor neuron disease, and multifocal motor neuropathy are all characterized by preservation of the sensory system. In contrast, polyneuropathies, bilateral radiculopathies, myelopathies, and central nervous system disorders frequently result in reduced sensation. In persons with no sensory loss or weakness on examination and preserved reflexes, the electrodiagnostician should maintain a heightened suspicion for myofascial pain syndrome or fibromyalgia, polymyalgia rheumatica, or other musculoskeletal disorders.
Purpose of Electrodiagnostic Testing
See Appendix 8A .
Electrodiagnostic testing excludes conditions in the differential diagnosis and alters the referring diagnostic impression 42% of the time. Electrodiagnostic testing can also suggest severity or extent of the disorder beyond the clinical symptoms. Finally, there is utility in solidifying a diagnosis. For example, an unequivocal radiculopathy on electromyography (EMG) provides greater diagnostic certainty and identifies avenues of management.
The value of any test depends on the a priori certainty of the diagnosis in question. For a condition or diagnosis for which there is great certainty before additional testing, the results of the subsequent tests are of limited value. For instance, in a patient with acute-onset sciatica while lifting, L5 muscle weakness, a positive straight leg raise, and magnetic resonance imaging (MRI) showing a large extruded L4 to L5 nucleus pulposus, an EMG test will be of limited value in confirming the diagnosis of radiculopathy because the clinical picture is so convincing with or without EMG findings. In contrast, an elderly diabetic patient with sciatica, having limited physical examination findings and equivocal or age-related MRI changes, presents an unclear picture. In this case, electrodiagnostic testing may be of greater value, putting in perspective the imaging findings and identifying diabetic polyneuropathy if present.
Nerve Injury Classification
Peripheral nerve injury is one of the most common types of pathology likely to be encountered during an electrodiagnostic medicine evaluation. It is necessary to be familiar with the various classification systems available to categorize an insult to neural tissue.
Seddon Classification
The degree to which a nerve is damaged has obvious implications with regard to its present function and potential for recovery. There are essentially two general classification systems. In Seddon classification, there are three degrees or stages of injury to consider: neurapraxia, axonotmesis, and neurotmesis ( Table 8-1 ).
Type | Function | Pathologic Basis | Prognosis |
---|---|---|---|
Lundborg | |||
Physiologic Conduction Block | |||
Type a | Focal conduction block | Intraneural ischemia; metabolic (ionic) block; no nerve fiber changes | Excellent; immediately reversible |
Type b | Focal conduction block | Intraneural edema; increased endoneurial fluid pressure; metabolic block; little or no fiber changes | Recovery in days or weeks |
Seddon-Sunderland | |||
Neurapraxia | |||
Type 1 | Focal conduction block; primarily motor function and proprioception affected; some sensation and sympathetic function may be present | Local myelin injury, primarily larger fibers; axonal continuity; no Wallerian degeneration | Recovery in weeks to months |
Axonotmesis | |||
Type 2 | Loss of nerve conduction at injury site and distally | Disruption of axonal continuity with Wallerian degeneration; endoneurial tubes, perineurium, and epineurium intact | Axonal regeneration required for recovery; good prognosis, because original end organs reached |
Type 3 | Loss of nerve conduction at injury site and distally | Loss of axonal continuity and endoneurial tubes; perineurium and epineurium preserved | Disruption of endoneurial tubes, hemorrhage, and edema produce scarring; axonal misdirection; poor prognosis; surgery may be required |
Type 4 | Loss of nerve conduction at injury site and distally | Loss of axonal continuity, endoneurial tubes, and perineurium; epineurium intact | Total disruption of guiding elements; intraneural scarring and axonal misdirection; poor prognosis; surgery necessary |
Neurotmesis | |||
Type 5 | Loss of nerve conduction at injury site and distally | Severance of entire nerve | Surgical modification of nerve ends required; prognosis guarded and dependent on nature of injury and local factors |
Neurapraxia
The term neurapraxia is used to designate a mild degree of neural insult that results in blockage of impulse conduction across the affected segment. It is also acceptable to simply designate this type of neural insult as conduction block. The most important aspect of conduction block is its reversibility. Muscle wasting usually does not occur in conduction block because muscle innervation is maintained, and recovery is typically rapid enough to avoid disuse atrophy. Fibrillation potentials should not be observed in conduction block because the axon is not disrupted. Many nerve injuries are mixed lesions in which some fibers have conduction block, some have axonal loss, and others are demyelinated. In this case, it is certainly possible to observe fibrillation potentials.
Axonotmesis
The second degree of neural insult in Seddon classification is axonotmesis, which is a specific type of nerve injury where only the axon is physically disrupted, with preservation of the enveloping endoneurial and other supporting connective tissue structures (perineurium and epineurium). Compression of a profound nature and traction on the nerve are typical lesion etiologies. Once the axon has been disrupted, the characteristic changes of Wallerian degeneration occur. The fact that the endoneurium remains intact is a very important aspect of this type of injury. A preserved endoneurium implies that once the remnants of the degenerated nerve have been removed by phagocytosis, the regenerating axon simply has to follow its original course directly back to the appropriate end organ. A good prognosis can be expected when neural damage results only in axonotmesis.
Neurotmesis
The greatest degree of nerve disruption is designated in Seddon classification as neurotmesis. This is complete disruption of the axon and all supporting connective tissue structures, whereby the endoneurium, perineurium, and epineurium are no longer in continuity. This lesion has a poor prognosis for complete functional recovery. Surgical reapproximation of the nerve ends, if possible, will probably be required. Surgery does not guarantee proper endoneurial tube alignment, but it improves the chances that axonal growth will occur across the injury site.
Sunderland Classification
A second popular and more detailed classification is that proposed and modified by Sunderland. Sunderland classification is divided into five types of injury, based exclusively on which connective tissue components are disrupted (see Table 8-1 ). Type 1 injury corresponds to Seddon designation of neurapraxia. Seddon axonotmesis is subdivided by Sunderland into three gradations of neural insult (types 2 to 4). Sunderland type 5 injury corresponds to Seddon neurotmesis (complete neural disruption).
Clinical Testing of Motor and Sensory Nerves
Electrodiagnostic testing consists of EMG and nerve conductions. Standard nerve conduction studies (NCSs) that are used in the evaluation of patients include motor nerve conductions, sensory nerve conductions, F-waves, and H-reflexes. It is important to properly perform these tests and compare the data with well-derived normative values. Enough testing should be performed to properly delineate the conditions being sought and eliminate other conditions in the differential diagnosis.
Although it is important to be aware of individuals who have implanted cardiac devices, in a study involving patients with dual-chamber pacemakers and implanted cardiac defibrillators, NCSs including median motor testing with an Erb point stimulation on the left side of the body resulted in no change in the function of these devices, nor did any stimulation show up on any of the electrocardiogram tracings. These investigators concluded that conventional NCSs have no effects on these implanted devices, and their findings should diminish the anxiety of electrodiagnosticians when working with such patients. These findings further support the safety of performing such testing in persons with implanted cardiac devices and dispel some of the concern expressed in previous recommendations.
Sensory nerve conductions should nearly always be performed when assessing a patient. In the upper limb, multiple sensory nerves are easily accessible and allow assessment for both entrapments and polyneuropathies. In the lower limbs, the most readily accessible sensory nerve is the sural nerve, which has been recommended as an excellent nerve for screening for distal symmetrical polyneuropathy.
Motor NCSs should be performed in almost all situations. Examiners should assess the morphology of the waveform, its latency, amplitude, and conduction velocity. Conduction should be performed across any suspected sites of entrapment or injury.
Despite efforts to minimize variability and enhance quality in the practice of electrodiagnostic medicine, it is well recognized that universal standards for NCSs are not used in the United States. The majority of electrodiagnostic physicians and laboratories, rather than develop their own normative values, have instead relied on the peer-reviewed literature. However, many published studies, compiled in various reference texts, do not meet contemporary standards for statistical and methodological rigor.
What is concerning about the lack of standardization of nerve conduction testing is the poor interrater reliability for identifying abnormal results that lead to differing diagnostic conclusions. A recent study delineated the problems in identifying polyneuropathy that arise when different experts and technologists use their own laboratory standards when evaluating patients with and without diabetic sensorimotor polyneuropathy. In this well-done prospective study they found that “interobserver variability” was attributed to differences in performance of nerve conduction testing.
In a companion study, Litchy et al. instituted standard instructions, standard techniques and procedures, and significantly reduced the interobserver differences in nerve conduction. Together, these two investigations underscore the need for standard well-derived reference values for practice across the United States.
H-Reflexes
The commonly used H-reflex is an electrophysiologically recorded Achilles muscle stretch reflex without the use of the muscle spindle. Clinical H-reflexes are generated by recording over the gastrocnemius and soleus muscles and stimulating the tibial nerve in the popliteal fossa. Care must be taken to perform this test correctly. It is a submaximal elicited reflex response. The stimulus duration is 1 ms, and the stimulus should be slowly increased by 3- to 5-mA increments. The patient should be relaxed, and the stimulus frequency should be less than once per second to avoid habituation of the response. The H-reflex is consistent in latency and morphology, and occurs when the motor response over the gastrocnemius and soleus is submaximal. As the stimulus current is gradually increased, the H-reflex reaches its maximum amplitude, and then extinguishes as the motor response becomes maximal. Many researchers have evaluated its sensitivity and specificity with regard to lumbosacral radiculopathies, and generally found a range of sensitivities from 32% to 88%. The specificity, however, has been reported at 91% for H-reflexes in lumbosacral S1 radiculopathy. The H-reflex can also help separate S1 radiculopathy from L5 radiculopathy, the latter being more likely to have a normal reflex.
H-reflexes are useful for assessing for demyelinating polyneuropathy, cauda equina syndrome, and for confirming a sciatic neuropathy. Remember that delay or decreased amplitude in the H-reflex can occur with lesions anywhere along its course, including the sciatic nerve, the lumbosacral plexus, or the S1 root. H-Reflex studies of other nerves are more difficult to elicit and they are not commonly used in clinical practice.
F-Waves
F-waves are late responses involving the motor axons and axonal pool at the spinal cord level. They can be elicited in most upper and lower limb muscles, and are typically tested by stimulating the median, ulnar, peroneal, or tibial nerves when recording from muscles they innervate. In contrast to H-reflexes, they are elicited by maximal stimulation of the nerve. They vary in morphology and latency, although when recording multiple F-waves, they should fall roughly within the same latency period. When responses that look like F-waves are seen across the screen at widely varying latencies, the examiner should turn up the machine volume, discharge the stimulator while it is off the skin, and determine whether background motor unit firing is the cause of these widely varying responses. F-waves demonstrate low sensitivities for radiculopathy and thus have a limited role in such evaluations ; however, they are useful in the assessment of suspected polyneuropathy.
Needle Electromyography
One of the most important means of evaluating for neuromuscular diseases is EMG using a monopolar pin or coaxial needle (“needle” is used to mean either pin or needle). Surface EMG has no clinical use over standard needle EMG. EMG testing, a vital part of electrodiagnostic evaluations, provides useful information and, although somewhat uncomfortable, poses minimal risks to the patient. The American Association of Neuromuscular and Electrodiagnostic Medicine (AANEM) supports the Occupational Safety and Health Administration rule that mandates the use of gloves and universal precautions during testing. Disposable needles are now readily available and are sufficiently inexpensive that they should be used. Reusable surface electrodes should be cleaned with a 1 : 10 dilution of household bleach or 70% isopropyl alcohol solution between patients. Disposable surface electrodes are inexpensive, widely available, and easy to use.
Needlestick injuries are reported by the majority of electrodiagnosticians to have occurred at least once during their career. The most common preventable reason for injury was a perceived lack of time. Using a device to clamp the plastic sleeve that holds the needle and replacing the needle with a one-handed technique are recommended to help prevent needle sticks.
In most cases, it makes no difference whether the skin is antiseptically prepared before needle insertion. Some examiners use alcohol as an antiseptic, and certainly if the skin is not clean then alcohol represents a useful skin preparation. With regard to lymphedema one should use caution and sterilize the skin, as well as avoid traversing an infected space or an area of clearly taut skin that could weep serous fluid after the needle insertion. Care should also be used to avoid cellulitis. The needle should not penetrate infected skin or open ulcerations or wounds. Needle EMG is not listed by the American Heart Association as a procedure requiring prophylactic antibiotic treatment to prevent endocarditis.
Hemorrhagic complications from needle EMG are rare and are asymptomatic when they occur. In one case series, minor paraspinal muscle hematomas were noted on MRI performed shortly after needle EMG examination in 4 of 17 patients who were not receiving anticoagulants. These hematomas were small and of no clinical significance. In this report, the authors state that there has never been a case report of paraspinal hematoma compressing the spinal roots or the epidural space. Ultrasound evaluation of over 700 muscles showed only two small subclinical hematomas in patients who were anticoagulated. However, since then, there has been at least one case of threatened compartment syndrome in the anterior tibialis of a patient who was subtherapeutically anticoagulated. Although clinicians should always weigh carefully the risk-to-benefit ratio of testing, a person with appropriate levels of anticoagulation for venous thromboembolism can be safely studied with little risk of neurologic complications. Appropriate pressure should be applied to the area after testing to prevent intramuscular hematoma. The paraspinal muscles are such an important region to study, not only for persons with suspected radiculopathy but also in neuromuscular evaluations that electrodiagnosticians should examine them whenever reasonably possible.
As noted, the electrodes for standard EMG generally fall into two categories. Monopolar needles are those in which the needle is coated with Teflon except for the tip, and the differences in potential from the tip of the electrode to a nearby surface electrode are recorded. Concentric needles are those with a fine wire running through the center of an insulated shaft that is electrically referenced to an outer metal shaft. Concentric needles are useful for quantitative motor unit analysis and are commercially available in disposable form. Background interference is minimized with concentric needles, and the ground electrode can remain in one place for a given limb.
The needle examination consists of assessing four separate aspects of needle EMG. These aspects are (1) insertion activity, (2) spontaneous activity, (3) the morphology and size of motor unit action potentials (MUAPs), and (4) motor unit recruitment.
Needle Insertional Activity
Placing a needle (monopolar or standard concentric) recording electrode into healthy muscle tissue and advancing it in quick but short intervals results in brief bursts of electrical potentials referred to as insertional activity ( Figure 8-2, A ).

The observed electrical activity is believed to result from the needle electrode mechanically depolarizing the muscle fibers surrounding its leading edge as it pierces and deforms the tissue. Insertional activity and spontaneous activity are usually examined with three or four insertions in each of four muscle vectors or quadrants. Smaller muscles, such as the abductor pollicis brevis, can be adequately assessed with fewer needle movements. After brief small movement of the needle, insertional activity usually persists for no more than a few hundred milliseconds. Minimal and localized muscle tissue damage may occur from direct needle trauma and is the basis for the synonymous term of injury potentials. The purpose of including insertional activity analysis as part of the electromyographic examination is that the probing needle might provoke transient and/or sustained abnormal potentials associated with membrane instability before this abnormal activity with the muscle at rest.
Decreased Insertional Activity
Atrophied muscle that has been replaced by fibrous or fatty tissue, or that is otherwise electrically inexcitable, can no longer generate electrical activity. Consequently, the needle electrode is incapable of mechanically depolarizing this tissue. The result is that few, if any, electrical waveforms will be detected after needle movement and usually lasts less than 100 ms. This is usually considered abnormal. Care must be taken to insure that the lack of electrical activity is not caused by placement of the needle into subcutaneous tissue instead of muscle (see Figure 8-2, B ).
Increased Insertional Activity
Practitioners have noted that insertional activity can appear to persist after needle movement cessation. This finding has led to the term increased insertional activity. In disease states in which the muscle is no longer connected to its nerve or the muscle membrane is inherently unstable from primary muscle pathology or abnormal ion channels, the increased insertional activity completes a temporal continuum from the previously normal insertional activity to the development of sustained membrane instability or positive sharp wave (PSW) fibrillation potentials (see Figure 8-2, C ). Practitioners differ as to the significant differences if any between increased insertional activity and PSWs. There is no clear conventional cut-off time between the time that persistent increased insertional activity becomes a run of fibrillation potentials. Many practitioners consider this cut-off time between 1 and 3 seconds after the needle has stopped moving. Very few authors specifically address this detail. The use of the longer time of 2 or 3 seconds improves the specificity and limits false-positive results but slightly decreases the sensitivity of the needle EMG. Diffuse abnormal insertional activity with prolonged trains of PSWs in essentially every muscle, yet without any symptoms or disability, has been described as “EMG disease.” This is a rare condition that is infrequently encountered in clinical practice and is of unclear clinical significance.
End-Plate Potentials
An active needle electrode located in the end-plate region can record two distinct waveforms: either miniature end-plate potentials (MEPPs) or end-plate spikes.
Miniature End-Plate Potentials
One of the waveforms is a short-duration (0.5 to 2 ms), small (10 to 50 µV), irregularly occurring (once every 5 seconds per axon terminal), monophasic negative waveform. These potentials represent the random release of acetylcholine vesicles. Volume conduction theory would suggest that for a potential to be monophasic and negative, the current sink would have to start and finish within the very small recording region of the active electrode ( Table 8-2 ). Clinically, multiple MEPPs are the same as end-plate noise, and the sound is referred to as resembling a “seashell murmur,” which sounds like noise generated by a seashell held close to the ear ( Figure 8-3 ).
Characteristic | Details |
---|---|
Appearance | Monophasic negative waves |
Rhythm | Irregular |
Frequency | Usually so frequent and can be superimposed upon each other so that they cannot be individually seen |
Amplitude | 10 µV or less |
Stability | Persist until the needle is moved |
Observed in | Normal finding recorded from the end-plate zone |

End-Plate Spikes
A second waveform (end-plate spikes) can be detected with an active needle electrode placed in the end-plate region. These potentials are generated from the actual muscle fiber, similar to fibrillation potentials, and they are relatively short in duration (3 to 4 ms), of moderate amplitude (100 to 200 µV), irregularly firing, and biphasic with an initial negative deflection ( Table 8-3 ). End-plate spikes and MEPPs are frequently observed together, because they arise from the same region ( Figure 8-4 ). They can be mistaken for fibrillation potentials, but they fire irregularly, a distinction usually made visually on the display screen as well as by listening carefully. The needle should be moved to a new location after it enters an end-plate region, to reduce pain and enter muscle more conducive to assessment for fibrillation potentials.
Characteristic | Details |
---|---|
Appearance | Biphasic or triphasic spikes or positive waves |
Rhythm | Irregular |
Frequency | Intervals frequently less than 50 ms |
Amplitude | 50 to 300 µV |
Stability | Persists until the needle is moved |
Observed in | Is a normal finding |

Single Muscle Fiber
The single muscle fiber generates three waveforms that are at times difficult to separate: end-plate spikes and the two types of fibrillations (PSWs and spike fibrillation potentials). Distant motor units can mimic the shape of the PSW but fire in a semirhythmic manner and by moving the needle closer the positive waveform becomes an MUAP. In contrast, moving the needle electrode 1 to 5 mm will cause fibrillations to disappear. End-plate spikes can have the identical morphology of PSW and fibrillation potentials. The major key to separating them is that PSW and fibrillation potentials have a regular firing rate and can slowly trail off before they disappear. End-plate spikes usually fire irregularly. The extracellular waveform configuration of a single muscle fiber, similar to nerve tissue, depends on the characteristics of the intracellular action potential of a muscle. The action potential of a muscle is approximately 4 to 20 times longer than that of a nerve, because of the prolonged repolarization process. A triphasic waveform with a small terminal phase should be recorded from an extracellular active electrode placed adjacent to a propagating single muscle fiber action potential at some distance from the end-plate region ( Figure 8-5 ).

Fibrillation Potentials: Spike Form and Positive Sharp Waves
There is debate as to whether fibrillation potentials are distinct from PSWs, and if so, whether or not this has any clinical significance. Fibrillation potentials have been characterized as the spike form or biphasic waveform. Characteristics are shown in Table 8-4 . There is strong evidence that the PSW is a fibrillation that has a different shape primarily because the needle electrode is touching the fibrillating muscle cell, altering the muscle membrane, and consequently the shape of the waveform. It is unlikely that there are any clinically significant differences between the two.
Characteristic | Details |
---|---|
Appearance | Brief spike or positive sharp wave |
Rhythm | Regular, rarely irregular |
Frequency | 0.5 to 15 Hz |
Amplitude | 20 to 300 µV |
Stability | Gradually taper off |
Observed in | Nearly all lower motor neuron disorders, severe myopathies, and occasionally severe neuromuscular junction disorders. Chronic spinal cord injuries and stroke. |
Fibrillation potentials are simply spontaneous depolarizations of a single muscle fiber and demonstrate waveform configurations similar to those of single muscle fibers that are voluntarily activated ( Figure 8-6 ). Fibrillation potentials are typically short in duration (less than 5 ms), less than 1 mV in amplitude, and fire at rates between 1 and 15 Hz. They have a typical sound, likened to a high-pitched tick similar to “rain on a tin roof,” when amplified through a loudspeaker. When the recording electrode is located in the previous end-plate zone of a denervated muscle, fibrillation potentials can be biphasic with an initial negative deflection. A recording electrode outside the end-plate zone, but far from the tendinous region, will detect fibrillation potentials that are triphasic (positive-negative-positive).

It is possible to generate waveforms with similar configurations to fibrillation potentials while examining healthy muscle tissue. Specifically, inadvertently irritating the terminal axon or end-plate zone with the shaft of an electrode, and evoking end-plate potentials while simultaneously recording at some distance from the end-plate zone, will generate triphasic end-plate spikes that are identical in appearance to fibrillation potentials ( Figure 8-7 ). This is understandable because both waveforms are single muscle fiber discharges. The key to identification is the rapid and irregular discharge of end-plate spikes, whereas fibrillation potentials usually fire slower and very regularly. Irregularly firing fibrillation potentials occur at times, and are less well understood but thought to arise from spontaneous depolarizations within the transverse tubule system.

Positive Sharp Waves
PSWs are fibrillation potential waveforms that can be recorded from a single muscle fiber having an unstable resting membrane potential secondary to denervation or intrinsic muscle disease. Typically, they have a large primary sharp positive deflection followed by a small negative potential. These potentials are called PSWs (see Figure 8-6 ). This waveform is thought to have the same clinical significance as a fibrillation potential, in that it is a single muscle fiber discharge. Amplified through a loudspeaker, PSWs have a regular firing rate (1 to 15 Hz) and a dull thud sound. Their durations range from several milliseconds to 100 ms or longer. Although commonly observed to fire spontaneously, PSWs can be provoked by electrode movement, as can the spike form of fibrillation potentials.
A number of other waveforms can be observed that have the configurations resembling a PSW. An MUAP recorded from the tendinous region can also have an initial positive deflection followed by a negative potential, because the current sink cannot pass beyond the recording electrode. It is also possible for the recording electrode to damage a number of muscle fibers in close proximity to the recording surface, again preventing an action potential from passing its recording surface, resulting in a primarily positive potential. These two potentials can be distinguished from a PSW in that they are MUAPs and subject to voluntary control, whereas a PSW is not. Asking the individual to contract and relax the muscle under investigation should demonstrate that the waveform has a variable firing rate. A PSW typically fires at a regular rate and is not under voluntary control. If any doubt remains, the electrode should be repositioned until successful recordings are obtained. Similar to end-plate noise and end-plate spikes, PSWs and fibrillations will usually disappear when the needle electrode is moved a small distance such as 1 to 5 mm, whereas a motor unit will usually remain on the screen and change shape. This is because the fibrillation potential is generated by a very small electrical charge from only one muscle fiber, whereas an MUAP is generated by hundreds of muscle fibers covering an area of 1 to 3 cm 2 .
Transient runs of “PSW-appearing potentials” can be seen in healthy skeletal muscle, particularly in the paraspinal and hand or foot intrinsic muscles. The “nonpathologic” PSWs are thought to arise because the needle electrode is oriented in such a manner as to irritate the terminal axon of an end-plate, but extend along the muscle fiber while compressing the tissue and preventing action potential conduction. The induced end-plate spike appears similar to a PSW, but it displays a relatively rapid and irregularly firing rate characteristic of end-plate spikes (see Figure 8-7 ). Sustained PSWs are more significant than unsustained PSWs.
Diffuse abnormal insertional activity with prolonged trains of PSWs in essentially every muscle, yet without any symptoms or disability, has been described as “EMG disease.” This is a rare condition that is infrequently encountered in clinical practice.
Fibrillations are seen in any condition causing denervation, including nerve disease, inflammatory myopathies, and direct muscle trauma. In persons with complete spinal cord injury, muscles innervated by roots below the level of the lesion also demonstrate fibrillation potentials. Such prevalence of these findings in the legs of patients with spinal cord injuries can make electrodiagnostic testing for such patients ineffective at identifying radiculopathies or entrapment neuropathies. Similar fibrillations and positive waves can also be seen in the hemiparetic limbs of patients with strokes, and such findings should be interpreted with caution.
Fibrillation potentials as well as PSWs are commonly graded with a 0- to 4-grading scheme. This grading scale is described as follows:
1+: Transient but reproducible trains of discharges (fibrillations or PSWs) after moving the needle in more than one site or quadrant.
2+: Occasional spontaneous potentials in more than two different quadrants.
3+: Spontaneous potentials present in all quadrants.
4+: Abundant spontaneous potentials nearly filling the screen in all four quadrants.
Complex Repetitive Discharge
A complex repetitive discharge (CRD) is a spontaneously firing group of action potentials (formerly called bizarre high-frequency discharges or pseudomyotonic discharges) and require a needle recording electrode for detection. The configuration of these waveforms is that they are continuous runs of simple or complex spike patterns (fibrillation potentials or PSWs) that regularly repeat at 0.3 to 150 Hz. The repetitive pattern of spike potentials has the same appearance with each firing and bears the same relationship with its neighboring spikes ( Table 8-5 ).
Characteristic | Details |
---|---|
Appearance | May take any form, but this form is constant from one potential complex to the next |
Rhythm | Regular |
Frequency | 10 to 100 Hz |
Amplitude | 50 to 1000 µV |
Stability | Abrupt onset and cessation |
Observed in | Myopathies: polymyositis, limb-girdle dystrophy, myxedema, Schwartz-Jampel syndrome. Neuropathies: poliomyelitis, spinal muscular atrophy, amyotrophic lateral sclerosis, hereditary neuropathies, chronic neuropathies, carpal tunnel syndrome. “Normal”: iliopsoas, biceps brachii. |
A distinct sound, likened to that of heavy machinery or an idling motorcycle, is produced by the firing of CRDs. In addition to the sound and repetitive pattern, a hallmark of these waveforms is that they start and stop abruptly. CRDs might begin spontaneously or be induced by needle movement, muscle percussion, or muscle contraction. Nerve block and curare do not abolish CRDs, suggesting that the origin of these potentials is within the muscle tissue. Single-fiber and standard electromyographic studies suggest that CRDs are generated by an ephaptic activation of adjacent muscle cells. Detection of these waveforms usually suggests that a chronic process has resulted in a group of muscle fibers becoming separated from their neuromuscular junctions. They may be associated with a currently active disease or be the residua of a past disorder.
Myotonic Discharges
The phenomenon of delayed muscle relaxation after muscle contraction is referred to as myotonia or action myotonia. The finding of delayed muscle relaxation after reflex activation, or induced by striking the muscle belly with a reflex hammer, is called percussion myotonia. Clinical myotonia is usually accentuated by energetic muscle activity after a rest period. Continued muscle contraction lessens the myotonia and is known as the “warmup.” It is thought that cooling the muscle accentuates myotonia, but this finding has only been objectively documented in paramyotonia congenita.
Myotonic discharges can present in one of two waveform types ( Table 8-6 ).
Characteristic | Details |
---|---|
Appearance | Brief spikes, positive waveform |
Rhythm | Wax and wane |
Frequency | 20 to 100 Hz |
Amplitude | Variable (20 to 300 µV) |
Stability | Firing rate alterations |
Observed in | Myopathies: myotonic dystrophy, myotonia congenita, paramyotonia, polymyositis, acid maltase deficiency, hyperkalemic periodic paralysis. Other: chronic radiculopathy, chronic peripheral neuropathy. |
The myotonic potential induced by needle electrode insertion usually assumes a morphology similar to that of a PSW or a fibrillation potential. It is thought that the needle movement induces a repetitive firing of the unstable membranes of multiple single muscle fibers. This is because the recording needle is thought to have damaged that portion of the muscle fiber with which it is in contact. Regardless of the waveform type, the hallmark of myotonia is the waxing and waning in both frequency and amplitude. The myotonic discharge has a characteristic sound, likened to that of a dive bomber. Trains of PSW that trail off and stop but do not restart (wane, but do not wax) can be confused with myotonia. Amplitudes range from 20 to 300 µV, and firing rates from 20 to 100 Hz.
Myotonic discharges can occur with or without clinical myotonia. The observation of these potentials requires needle movement or muscle contraction. These waveforms persist after nerve block, neuromuscular block, or frank denervation. This suggests that their site of origin is the muscle membrane itself, and they appear to be attributable to a channelopathy. Although the exact mechanism of myotonic discharge production remains unclear, myotonia is found in channelopathies of both the sodium and potassium channels. Myotonic discharges are not specific for any one disorder. In addition to the myotonic disorders of muscle, myotonic discharges can also be detected occasionally in acid maltase deficiency, polymyositis, drug-induced myopathies, severe axonal neuropathies, and at times in any nerve or muscle disorder.
Fasciculation Potentials
The visible spontaneous contraction of a portion of muscle is referred to as a fasciculation. When these contractions are observed with an intramuscular needle recording electrode, they are called fasciculation potentials. A fasciculation potential is the electrically summated voltage of depolarizing muscle fibers belonging to all or part of one motor unit. Occasionally, fasciculation potentials can be documented only with needle EMG because they lie too deep in muscle to be seen from the skin. Recently, ultrasound has been used to visualize fasciculation potentials in skeletal muscle.
Fasciculation waveforms can be characterized with regard to phasicity, amplitude, and duration ( Table 8-7 ). Their discharge rates (absent or less than 1 per minute to 1 to 2 Hz) are irregular and occur randomly. They are not under voluntary control, nor are they influenced by mild contraction of the agonist or antagonist muscles. The site of origin of fasciculation potentials remains unclear, although it appears that there are three possible sites: the spontaneous discharge might arise from the anterior horn cell, or along the entire peripheral nerve (particularly the terminal portion), or at times within the muscle itself.
Characteristic | Details |
---|---|
Appearance | Single motor unit |
Rhythm | Irregular and random |
Frequency | Variable, absent to 1 to 2 Hz |
Amplitude | Variable, similar to motor units, 20 µV to 20 mV depending on needle location and motor unit characteristics. |
Stability | Each fasciculation is an individual motor unit and is looks different. If the same unit is fasciculating frequently, it is usually stable unless that motor unit happens to be unstable and thus likely to be pathologic. |
Observed in | Nearly all normal people. Is more frequent in nearly any lower motor neuron disorders. If they are accompanied by fibrillations they are much more likely to be abnormal. |
Fasciculation potentials occur in almost all normal persons in the foot intrinsic or gastrocsoleus muscles, and in patients with a variety of diseases. Typical diseases in which fasciculation potentials can be found include motor neuron disorders, radiculopathies, entrapment neuropathies, and cervical spondylotic myelopathy. Fasciculation potentials have also been described in metabolic disturbances, including tetany, thyrotoxicosis, and anticholinesterase overdoses. Studies have unsuccessfully attempted to distinguish between benign (normal) and pathologic fasciculation potentials. There is no reliable way to categorize whether fasciculation potentials indicate a disease state just by considering their inherent characteristics based on routine needle EMG. Perhaps the best way to evaluate fasciculation potentials is to analyze the “company they keep.” A careful analysis of voluntary MUAP morphology, combined with a search for abnormal spontaneous potentials, is required before concluding that fasciculation potentials are either a normal or an abnormal finding. Their frequency can be measured by waiting for 1 minute and documenting the number of fasciculations per minute.
Myokymic Discharge
Myokymia is frequently readily observable as vermicular (bag of live worms) or rippling movement of the skin but can be invisible on the skin. It is usually associated with myokymic discharges. The myokymic discharge consists of bursts of normal-appearing motor units with interburst intervals of electrical silence. Thus, there are two frequencies. Typically, the slow firing rate is 0.1 to 10 Hz in a semirhythmic pattern. Each burst has 2 to 10 potentials within a single burst that can fire at 20 to 150 Hz. These potentials are not affected by voluntary contraction. The sounds associated with these potentials vary and have been described as a type of sputtering often heard with a low-powered motorboat engine, marching soldiers, or bursts of machine gun. The actual discharge may be distinguished from CRDs in that myokymic discharges do not display a regular pattern of spikes from one burst to the next, nor do they typically start and stop abruptly. Myokymic discharges are groups of motor units, whereas CRDs represent groups of single muscle fibers. The groups of motor units within a burst may fire only once or possibly several times. The sputtering bursts of myokymic discharges usually sound very different than the continuous drone of a CRD.
Myokymic potentials are rarely seen in healthy people. They are likely to originate from demyelinated motor axons either spontaneously or through ephaptic transmission. Characteristics of myokymic discharges are seen in Table 8-8 .
Characteristic | Details |
---|---|
Appearance | Normal motor unit action potentials |
Rhythm | Regular |
Frequency | 0.1 to 10 Hz |
Burst frequency | 20 to 250 Hz |
Stability | Persistent firing or occasional abrupt cessation. |
Observed in | Facial: multiple sclerosis, brainstem neoplasm, polyradiculopathy, Bell palsy, normal. Extremity: radiation plexopathy, chronic nerve compression (carpal tunnel syndrome, radiculopathy), rattlesnake venom. |
Cramp Potentials
A sustained and possibly painful muscle contraction of multiple motor units, lasting seconds or minutes, can appear in normal individuals or specific disease states. In healthy individuals, a cramp usually occurs in the calf muscles or other lower limb muscles after exercise, abnormal positioning, or maintaining a fixed position for a prolonged period. Cramps might also be induced by hyponatremia, hypocalcemia, vitamin deficiency, ischemia, early motor neuron disease, and peripheral neuropathies. Familial syndromes have been reported that involve fasciculations and cramps; alopecia, diarrhea, and cramps; and simply an autosomal dominantly inherited cramp syndrome.
A needle recording electrode placed into a cramping muscle shows multiple motor units firing synchronously between 40 and 60 Hz, and occasionally reaching 200 to 300 Hz ( Figure 8-8 and Table 8-9 ). Cramps are believed to arise from a peripheral portion of the motor unit. A cramp that results in a taut muscle with electrical silence is the physiologic contracture seen in McArdle disease.

Characteristic | Details |
---|---|
Appearance | Single and multiple motor units. |
Rhythm | Can fire similar to motor units in groups. |
Frequency | Very fast, 40 to 200 Hz. |
Amplitude | Variable similar to motor units, 20 µV to 20 mV depending on needle location and motor unit characteristics. |
Stability | Variable number of units that fire, but each unit is usually the same morphology. |
Observed in | Can be seen in normal people (nocturnal or after exercise). Wide range of neuropathic, endocrinologic, and metabolic conditions. |
Neuromyotonic Discharges
Neuromyotonia is a rare “peripheral” form of continuous muscle fiber activity originating in the peripheral motor axon, usually referred to as Isaac syndrome ( Table 8-10 ). The continuous motor unit activity is eliminated by neuromuscular block but not peripheral nerve block, spinal or general anesthesia, or sleep. The motor unit activity usually begins in the lower limbs in the late teens and progresses to all skeletal muscles. Needle recordings demonstrate motor unit discharges with frequencies up to 300 Hz, including doublets, triplets, and multiplets. Intraoperative mechanical stimulation of a nerve can cause a form of neuromyotonia called neurotonic discharges. These bursts of motor units can alert the surgeon that the nerve is being mechanically disturbed and is at risk of being damaged.
Characteristic | Details |
---|---|
Appearance | Single and multiple motor units that decrease in size over the time of the contraction. |
Rhythm | Appear regular. |
Frequency | Very fast, 150 to 250 Hz. |
Amplitude | Variable similar to motor units but decrease over time, 20 µV to 20 mV depending on needle location and motor unit characteristics. |
Stability | Stable and gradually decrease but each unit is usually the same morphology. |
Observed in | Isaacs’ syndrome, extremely chronic neuropathic diseases, familial neuromyotonia. |
Motor Units
See Appendix 8B .
Motor unit morphology assessment is generally a qualitative statement and less clear than the presence or absence of fibrillations. Such motor unit changes, however, when clear and profound can be very helpful, particularly in the proper clinical context. The “rule of five” suggests that in normal situations, the ratio of highest firing rate to the number of units seen on a 100-ms display screen is less than five. This means that if the firing rate of the fastest firing motor unit is 20 Hz, approximately four distinct motor units should be seen. In practice this becomes difficult because counting the exact number of motor units can be problematic when there are distant and/or small motor units. When this firing ratio is increased to greater than 10, it indicates a dropout of motor units and is termed reduced recruitment.
Reduced recruitment with a high firing ratio represents one end of a spectrum of recruitment findings, strongly suggestive of motor axonal loss or functional dropout resulting from conduction block. At the other end of a spectrum of recruitment findings is a situation in which many units are recruited to generate a low level of force. This is termed early or myopathic recruitment and is seen in myopathies and neuromuscular junction disorders. Such recruitment is often seen with small, short-duration MUAPs that are characteristic for myopathies. At these two extremes of recruitment, findings are relatively specific for the underlying types of neuromuscular pathology. Beyond this, many recruitment findings are less clear and nearly impossible to reliably differentiate from normal. In the case of pain, poor cooperation, or upper motor neuron disorders, there can be slow firing rates with few units activated ( Table 8-11 ).
Characteristic | Details |
---|---|
Appearance | Variable can be triphasic or polyphasic. |
Rhythm | Semirhythmic or variable regularity. Not randomly irregular. |
Frequency | 5 to 50 Hz. |
Amplitude | Variable, 20 µV to 20 mV depending on needle location and motor unit characteristics. |
Stability | Stable if normal, occasional alterations in shape in some diseases. |
Observed in | Normal and abnormal patients if they have motor units |
Extent of Electrodiagnostic Testing
See Appendix 8C .
Guidelines from the AANEM, published in 1999, provide valuable guidance regarding the extent of appropriate electrodiagnostic testing in 90% of cases. The guidelines and recommendations are summarized in Table 8-12 . Guidelines in electrodiagnostic medicine provide recommendations for testing and give clinicians and insurers crucial information regarding how many tests should be conducted in most cases to expeditiously arrive at a diagnosis (e.g., what is enough and what is excessive). These guidelines were developed to improve electrodiagnostic patient care, as well as to combat unscrupulous providers of electrodiagnostic services in the United States who perform excessive studies beyond what is necessary to make a diagnosis, simply for the purpose of increasing the electrodiagnostic fee.
Suspected Condition | Sensory Testing | Motor Testing | Electromyography | Proximal Conductions | Other Special Tests |
---|---|---|---|---|---|
Radiculopathy | One sensory NCS to exclude polyneuropathy | One motor NCS to exclude polyneuropathy | Sufficient number of muscles representing all myotomes with paraspinal muscles | None | None |
Carpal tunnel syndrome | Median sensory NCS and another for comparison | Median motor NCS and another for comparison | Optional, although useful to determine severity of CTS and exclude concomitant C8 radiculopathy | None | None |
Ulnar neuropathy | Ulnar sensory NCS and another for comparison | Ulnar motor NCS and another for comparison; need to examine conduction velocity across elbow | Several ulnar-innervated muscles, always the first dorsal interosseus, as it is most frequently abnormal, plus median muscles for comparison to exclude C8 radiculopathy | None | Inching motor NCS across the elbow |
Peroneal NCS neuropathy | Superficial peroneal sensory NCS and another for comparison | Peroneal motor with stimulation below and above the fibular head, assessing for conduction velocity and amplitude changes; test another for comparison | Peroneal nerve–innervated muscles and tibial nerve muscles for comparison; suggest paraspinal and L4-L5 muscles to assess for radiculopathy | None | None |
Entrapment neuropathies | Two sensory NCSs, one in the distribution and a comparison | Two motor NCSs, one in the distribution and a comparison | Muscles in the involved distribution and comparison muscles | None | None |
Myopathy | One or two sensory NCSs in a clinically involved limb | One or two motor NCSs in a clinically involved limb | Two muscles (proximal and distal) in two limbs, one of which is symptomatic | — | Consider repetitive nerve stimulation |
Neuromuscular junction disorders | One sensory NCS in a clinically involved limb | One motor NCS in a clinically involved limb | One proximal and one distal muscle in clinically involved limbs | — | Repetitive nerve stimulation at 2 to 3 Hz in clinically weak muscle and, if normal, other weak muscles; single-fiber electromyography if high suspicion and repetitive nerve stimulation is negative—can be first test in ocular myasthenia gravis |
Polyneuropathy | Sensory NCS in at least two extremities: if abnormalities in one limb, study the contralateral limb; four or more may be necessary to classify the polyneuropathy | Motor NCS in at least two extremities: if abnormalities in one limb, study the contralateral limb; four or more may be necessary to classify the polyneuropathy | One distal muscle in both legs and a distal muscle in one arm | Consider proximal NCSs (H-reflexes, F-waves, and blink reflexes) to assess demyelination | — |
Motor neuron diseases | One sensory NCS in at least two clinically involved limbs | One motor NCS in at least two clinically involved limbs; proximal stimulation sites to exclude conduction block in the case of multifocal motor neuropathy | Several muscles in three extremities, or two extremities and cranial nerve–innervated muscles as well as lumbar or cervical paraspinal muscles; thoracic paraspinal muscles may be considered an extremity; sample distal and proximal muscles | Consider F-waves | Consider repetitive nerve stimulation |
Limitations of Electrodiagnosis
Unfortunately, very few electrodiagnostic findings are clearly specific for any single diagnostic entity. Repetitive nerve stimulation at 2 or 3 Hz can reveal decrements in neuromuscular junction disorders, but also in motor neuron disease, myopathies, peripheral neuropathies, and myotonic disorders. Fibrillations and PSWs are seen in polyneuropathies, motor neuron disease, inflammatory myopathies, radiculopathies, and entrapment neuropathies. Marked facilitation of the CMAP to more than 400% of the baseline amplitude after a brief contraction in persons with Lambert-Eaton myasthenic syndrome (LEMS) is one finding that is unique to this uncommon disease.
The time course over which a disease process progresses and the time at which electrodiagnostic testing is conducted both play major roles in determining whether the electrodiagnostic testing can provide a reasonably certain diagnosis. It is frequently necessary to repeat the study if the diagnosis remains in question or if the clinical situation changes. One important issue related to electrodiagnostic medicine is the possibility of false-positive results. In Table 8-13 , the probabilities of finding false-positive results on the basis of chance alone are shown according to the number of independent measures.
No. of Measurements | No. of “Abnormalities” | ||||
---|---|---|---|---|---|
1+ | 2+ | 3+ | 4+ | 5+ | |
1 | 2.5 | ||||
2 | 4.9 | 0.1 | |||
3 | 7.3 | 0.2 | <0.1 | ||
4 | 9.6 | 0.4 | <0.1 | <0.1 | |
5 | 11.9 | 0.6 | <0.1 | <0.1 | <0 |
6 | 14.0 | 0.9 | <0.1 | <0.1 | <0 |
7 | 16.2 | 1.2 | <0.1 | <0.1 | <0 |
8 | 18.3 | 1.6 | 0.1 | <0.1 | <0 |
9 | 20.4 | 2.0 | 0.1 | <0.1 | <0 |
10 | 22.4 | 2.5 | 0.2 | <0.1 | <0 |
15 | 31.5 | 5.2 | 0.5 | <0.1 | <0 |
20 | 39.5 | 8.5 | 1.3 | 0.1 | <0 |
* Each measurement has a 2.5% false-positive rate (mean ± 2 standard deviations for a Gaussian distribution).
It is important to realize that if five measurements are performed, there is a 12% chance of having one false-positive result. If nine measurements are made, there is a 20% chance of a spuriously false-positive result. If there are two abnormal results when six tests are conducted, however, the likelihood that they are false-positive findings is very low, less than approximately 1%. This underscores the need for electrodiagnosticians to critically examine their findings and not overdiagnose a disorder based on one subtle abnormality. If one electrodiagnostic parameter is markedly abnormal, far beyond the upper limit of normal, this can be compelling, particularly when that abnormality is consistent with the clinical impression. The study should be repeated to make certain of its validity. Two abnormalities indicating the same diagnosis, however, are far more likely to represent true findings and a clear underlying disorder.
Other common conditions, such as diabetes and its corresponding polyneuropathy, can make electrodiagnostic testing more difficult to interpret. With a diffuse polyneuropathy, the consultant is often unable to identify focal lesions against the backdrop of this disorder. In this scenario, the electrodiagnostician must assess the magnitude of the background polyneuropathy and make a judgment as to whether findings for the nerve in question are out of proportion to the background polyneuropathy. Caution is urged in this scenario to avoid overcalling entrapment neuropathies when a generalized polyneuropathy is present. These issues are another reason why it is critically important that the electrodiagnostician have sufficient training to be able to interpret the findings appropriately in the clinical context of the patient.
Anatomic variations in muscle and nerve pathways can also confound electrodiagnostic interpretation. Two well-described variants are important for the electrodiagnostician. The Martin-Gruber anastomosis is formed by a branch from the median nerve, usually the anterior interosseous nerve, joining the ulnar nerve in the forearm. In this situation, the median CMAP with wrist stimulation is smaller than that with proximal stimulation. The ulnar nerve demonstrates a larger amplitude with wrist stimulation than with below-elbow or above-elbow stimulation. If a suspected conduction block is found in the forearm when testing the ulnar nerve, then median nerve conduction should be performed to exclude a Martin-Gruber anastomosis as the cause of CMAP reduction with proximal stimulation.
An accessory deep peroneal nerve is an anomalous part of the deep peroneal nerve that remains with the superficial peroneal nerve and innervates the extensor digitorum brevis after passing behind the lateral malleolus. It should be suspected if stimulation at the fibular head yields a larger CMAP than ankle stimulation when recorded over the extensor digitorum brevis. Stimulation of the accessory deep peroneal nerve behind the lateral malleolus will confirm the presence of such an anatomic variant.
Standards of Practice for Electrodiagnostic Medicine
The AANEM has addressed the need to set standards for the evaluation and interpretation of studies in response to the burgeoning and disquieting practice of decoupling nerve conductions and needle EMG. It is the contention of the organization that electrodiagnostic studies should be performed by physicians properly trained in electrodiagnostic medicine, that interpretation of NCS data alone, absent face-to-face patient interaction and control over the process provides substandard care, and that performance of NCS without needle EMG has the potential of compromising patient care. Considerable concern exists that there has been a dramatic increase in NCSs without EMG testing. EMG testing is crucial for the assessment of radiculopathies and plexopathies as well as to complement NCSs and give additional information about entrapments and nerve injuries. Interpreting NCS findings without the benefit of a focused history and examination is also inappropriate. Communication of the consultation results to the referring physician and other health care providers is essential. The AANEM recommends that the electrodiagnostic report contain (1) a description of the problem and the reason for referral; (2) a focused history and examination; (3) tabular NCS and EMG information with normative reference values; and (4) temperature and limbs studied. It is recommended that the study results are defined as normal or abnormal, and a probable physiologic diagnosis is presented. Any limitations to the study should be included. An optional section after this summary can be a synthesis of the clinical and electrophysiologic information into clinical recommendations or a stronger set of conclusions. Such a section should be separate from the electrophysiologic testing. The EMG and NCS data and the conclusions they support should stand alone. Visual depictions of the waveforms, although not required, are an ideal means of documenting the nerve conduction findings and are usually available on modern electrodiagnostic instruments.
Pediatric Electrodiagnosis
The pediatric examination poses challenges for even the most experienced electrodiagnostic physician. Diagnosis of pediatric neuromuscular disorders is a specialized and rapidly evolving area of medicine in which genetic testing plays an ever greater role in diagnosis. Interested readers are encouraged to examine specialized textbooks and recent articles because much of this material is beyond the scope of this chapter.
In terms of equipment required for pediatric electrodiagnosis, a pediatric bipolar stimulating probe is available and is useful for small children. Monopolar needles are generally used because their diameter is smaller and insertional resistance is less, which is attributable to their Teflon coating. It is critically important to maintain a skin surface temperature of 36° C to 37° C to avoid spurious results. There are strong advocates for and against the use of sedation and analgesia during electrodiagnostic examination. Performance of all conscious sedation and anesthesia should be done by specialists in pediatric anesthesia. When general anesthetic is required, children with possible neuromuscular diseases should not be given halogenated inhalation agents such as halothane because of the risks for malignant hyperthermia. Nerve conduction testing should include both sensory and motor studies. The needle examination, although difficult, should always be part of the diagnostic evaluation. Despite the challenging nature of the needle examination, a complete needle EMG is necessary to reach appropriate conclusions. A single muscle exploration is insufficient for diagnosis in a child.
The conduction velocities in newborns are approximately half of those found in adults. There is a rapid increase in values during the first year of life. Median values for conduction velocities in children and adults are equalized by 5 years of age. Tables 8-14 and 8-15 show values for infants and newborns. Motor conduction velocities in full-term infants should be no less than 20 m/s.
Age ( n ) | Median Nerve † | Peroneal Nerve ‡ | ||||||
---|---|---|---|---|---|---|---|---|
Distal Motor Latency (ms) | Conduction Velocity (m/s) | F-Latency (ms) | Amplitude (mV) | Distal Motor Latency (ms) | Conduction Velocity (m/s) | F-Latency (ms) | Amplitude (mV) | |
7 days to 1 mo (20) | 2.23 (0.29) | 25.43 (3.84) | 16.12 (1.50) | 3.00 (0.31) | 2.43 (0.48) | 22.43 (1.22) | 22.07 (1.46) | 3.06 (1.26) |
1 to 6 mo (23) | 2.21 (0.34) | 34.35 (6.61) | 16.89 (1.65) | 7.37 (3.24) | 2.25 (0.48) | 35.18 (3.96) | 23.11 (1.89) | 5.23 (2.37) |
6 to 12 mo (25) | 2.13 (0.19) | 43.57 (4.78) | 17.31 (1.77) | 7.67 (4.45) | 2.31 (0.62) | 43.55 (3.77) | 25.86 (1.35) | 5.41 (2.01) |
1 to 2 yr (24) | 2.04 (0.18) | 48.23 (4.58) | 17.44 (1.29) | 8.90 (3.61) | 2.29 (0.43) | 51.42 (3.02) | 25.98 (1.95) | 5.80 (2.48) |
2 to 4 yr (22) | 2.18 (0.43) | 53.59 (5.29) | 17.91 (1.11) | 9.55 (4.34) | 2.62 (0.75) | 55.73 (4.45) | 29.52 (2.15) | 6.10 (2.99) |
4 to 6 yr (20) | 2.27 (0.45) | 56.26 (4.61) | 19.44 (1.51) | 10.37 (3.66) | 3.01 (0.43) | 56.14 (4.96) | 29.98 (2.68) | 7.10 (4.76) |
6 to 14 yr (21) | 2.73 (0.44) | 57.32 (3.35) | 23.23 (2.57) | 12.37 (4.79) | 3.25 (0.51) | 57.05 (4.54) | 34.27 (4.29) | 8.15 (4.19) |
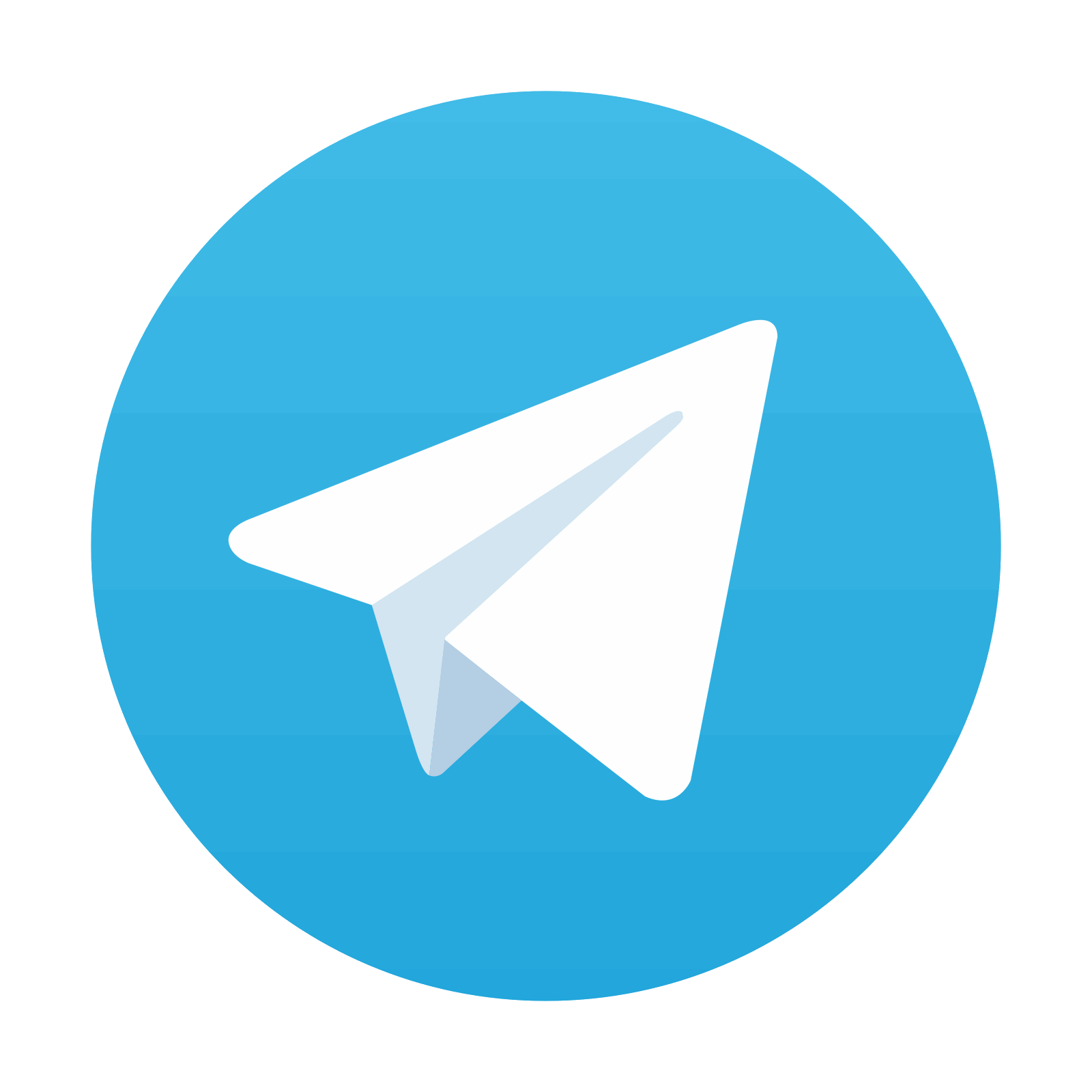
Stay updated, free articles. Join our Telegram channel

Full access? Get Clinical Tree
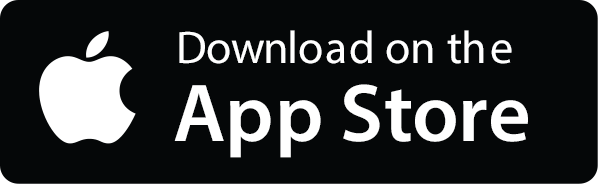
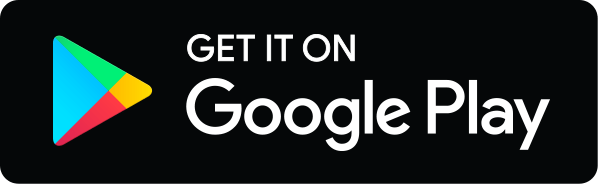
