Introduction
This study aimed to evaluate the effects of varying auxiliaries on tooth movement and stress distribution when maxillary central incisors were torqued 1° with a clear aligner through finite element analysis.
Methods
Three-dimensional finite element models, including maxillary alveolar bone, periodontal ligament, dentition, and clear aligner, were constructed. According to the auxiliaries designed on the maxillary central incisor, 5 models were created: (1) without auxiliaries (control model), (2) with the power ridge, (3) with the semi-ellipsoid attachment, (4) with the horizontal rectangular attachment, and (5) with the horizontal cylinder attachment. The tooth movement and periodontal ligament stress distribution after a palatal root torque of 1° were analyzed for each of the 5 models.
Results
With 1° torque predicted, the maxillary central incisor without auxiliaries showed a tendency of labial tipping, mesial tipping, and intrusion. The rotation center moved occlusally in the power ridge model. The labiolingual inclination variation increased in the semi-ellipsoid attachment model but decreased in the power ridge model. The maxillary central incisor is twisted in the distal direction in the power ridge model. The maxillary central incisor of the horizontal rectangular attachment and the horizontal cylinder attachment model behaved similarly to the control model. Periodontal stresses were concentrated in the cervical and apical areas. The maximum von Mises stresses were 11.6, 12.4, 3.81, 1.14, and 11.0 kPa in the 5 models. The semi-ellipsoid attachment model exhibited a more uniform stress distribution than the other models.
Conclusions
Semi-ellipsoid attachment performed better efficacy on labiolingual inclination, and power ridge performed better efficacy on root control. However, a distal twist of maxillary incisors could be generated by the power ridge.
Highlights
- •
Tooth movement of a maxillary incisor torqued with clear aligners was evaluated through FEA.
- •
Maxillary central incisors exhibited labial tipping, mesial tipping and intrusion when torqued.
- •
Semi-ellipsoid attachment had better efficacy on labiolingual inclination.
- •
Power ridge had better efficacy on root control.
- •
A distal twist of the maxillary incisors could be generated by the power ridge.
With patients’ ever-increasing attention to esthetics, clear aligners (CAs) have gained popularity in recent years because of their superior esthetics and comfort compared with conventional fixed appliances. CAs contain a series of removable clear polyurethane trays (aligners), moving teeth in a predicted pattern out of the “mismatch” between the aligner and the teeth. With the advancement of material and auxiliary research, CAs are no longer limited to simple interventions focused on a broad spectrum of malocclusions. Consequently, increasing demands have been placed on the accuracy of tooth movement with the increasing applicability of CAs. However, questions concerning the tooth movement efficacy remain, as a significant proportion of patients required additional aligners, and some even have to resort to fixed appliances. Among various types of tooth movement, controlling the anterior labiolingual inclination has been reported as particularly challenging.
Torque, which was initially introduced by Andrews, represents the buccolingual or labiolingual inclination of the teeth. Because of the crucial role in both esthetics and functionality, clinicians and researchers have struggled to improve the efficacy of torque control with the assistance of auxiliaries such as attachments, power ridges, etc. The first study on the efficacy of Invisalign (Align Technology, San Jose, Calif) revealed a <50% efficacy on torque. Moreover, unwanted tipping movement occurred, and root movement was difficult. Power ridges have been widely applied in clinical practice to realize torque control. Castroflorio et al reported that torque loss was negligible within a torque correction of approximately 10°, whereas an approximately 50% torque loss was found when incisor torque exceeded 10°. In our previous retrospective study, we discovered an approximately 50% efficacy of torque movement for torque exceeding 5°, in which root movement still posed challenges. Therefore, torque control remains a challenge with CAs.
Finite element analysis (FEA), as a noninvasive virtual model, can simulate oral conditions and be applied to perform force analysis further. In the field of orthodontics, FEA has been extensively used to calculate stress and displacement patterns under different loading conditions, enabling biomechanical analysis and improving various appliances. FEA has allowed researchers to seek appropriate improvement measures in vitro before clinical validation, avoiding unnecessary clinical trials.
Regarding torque control, most scholars have focused on anterior en masse retraction, and less attention has been given to torque movement. Moreover, the effect of the power ridge and attachments on torque movement is unclear. Therefore, we conducted an FEA study to evaluate the effect of varying auxiliaries on tooth movement and stress distribution when maxillary central incisors were torqued 1° with a CA.
Material and methods
One healthy adult volunteer with Class II Division 2 malocclusion and a healthy periodontal condition was selected on the basis of the following criteria: (1) aged ≥18 years, (2) full permanent dentition except for third molars without supernumerary teeth in the anterior maxilla, (3) normal teeth morphology without root absorption, and (4) healthy periodontal condition without alveolar bone dehiscence and fenestration. The cone-beam computed tomography (CBCT) data were obtained with a NewTom VGi scanner (QR srl, Verona, Italy) with the following acquisition conditions: 110 kVp; 2 mA; voxel size, 0.3 mm; scanning time, 3.6 seconds; and volume, 15 cm × 15 cm. This study was approved by the Ethics Committee of the Affiliated Hospital of Stomatology, School of Stomatology, Zhejiang University School of Medicine (2021-03-349).
A 3-dimensional preliminary model of the maxilla and maxillary dentition was established with CBCT data using Mimics (version 20.0; Materialise, Leuven, Belgium). Then, Geomagic Wrap (version 2017; 3D Systems, Rock Hill, SC) was used to optimize the preliminary model and create a Nonuniform Rational B-Splines model. The periodontal ligament (PDL) was generated by evenly extending the outer surfaces of roots with thicknesses of 0.25 mm. To acquire the alveolar fossa of the maxilla, dentition, and PDL were subtracted from the maxilla by Boolean operation. Horizontal rectangular attachments (2 mm height, 3 mm width, and 1 mm thickness) were designed for the first molars, and vertical rectangular attachments (3 mm height, 2 mm width, and 1 mm thickness) were designed for the premolars and the canines. According to previous studies, , 4 auxiliaries were designed for the central incisors with Hypermesh (version 14.0; Altair Engineering Inc, Troy, Mich): ( Fig 1 , A ) the power ridge obtained by aligner local dented (4 mm width, 1 mm height, and 0.25 mm depth), ( Fig 1 , B ) the semi-ellipsoid attachment, ( Fig 1 , C ) the horizontal rectangular attachment, and ( Fig 1 , D ) the horizontal cylinder attachment as shown in Figure 1 . The aligner was established by extending the maxillary crown and attachment with a thickness of 0.75 mm, in which the maxillary central incisor rotated 1° counterclockwise around the crown center. Finally, the model was assembled for FEA. Models of all components are displayed in Figure 2 .


Regarding the treatment protocol in the clinic, 1° of aligner for incisor torque could be possible. Because FEA could only mimic single-step movement, a 1° palatal root torque was chosen in this study. To stimulate 1° palatal root torque, the aligner was imposed on the initial dentition, which deformed the aligner segment. The forces generated by the aligner on each tooth were then loaded back on the corresponding tooth in the reverse direction. Five models were assigned considering varying auxiliaries—(1) without auxiliaries, (2) with the power ridge, (3) with the semi-ellipsoid attachment, (4) with the horizontal rectangular attachment, and (5) with the horizontal cylinder attachment—in which all of the auxiliaries were located in the labial cervical third of crown ( Fig 3 ). The maxillary right central incisor was the main observation object, including the tooth movement and PDL stress distribution.

FEA was carried out with Abaqus (version 2022; Dassault Systems, Vélizy-Villacoublay, France). Model meshing was constructed with hexahedral elements for PDL and shell elements for the alveolar bone, dentition, and CA. The numbers of nodes and elements for all the components are summarized in Table I . Second, the material properties of each component were applied with reference to previous literature, as shown in Table II . All the components, including maxillary alveolar bone, PDL, dentition, CA, and attachment, were assumed to be linear elastic isotropic and homogeneous materials, in which bone cortex and cancellous structure were not distinguished for alveolar bone. In addition, pulp, dentin, and enamel were not distinguished for tooth. Considering the obvious rigidity difference between the PDL structure and hard tissue (alveolar bone and tooth), little influence could be caused by the above settings of the material properties.
Component | Elements | Nodes |
---|---|---|
Teeth | 129,458 | 129,130 |
PDL | 66,370 | 133,964 |
Alveolar bone | 158,104 | 159,442 |
Attachment (a) | 3328 | 3496 |
Attachment (b) | 3328 | 3496 |
Attachment (c) | 3886 | 4102 |
Attachment (d) | 5580 | 5814 |
Attachment (e) | 4736 | 4892 |
CA (a) | 88,286 | 89,186 |
CA (b) | 93,040 | 93,744 |
CA (c) | 92,336 | 93,038 |
CA (d) | 92,940 | 93,634 |
CA (e) | 92,258 | 92,938 |
Components | Young’s Modulus (MPa) | Poisson’s Ratio |
---|---|---|
Teeth | 19,600 | 0.30 |
Alveolar bone | 1370 | 0.30 |
PDL | 0.69 | 0.45 |
CA | 528 | 0.36 |
Attachment | 12,500 | 0.36 |
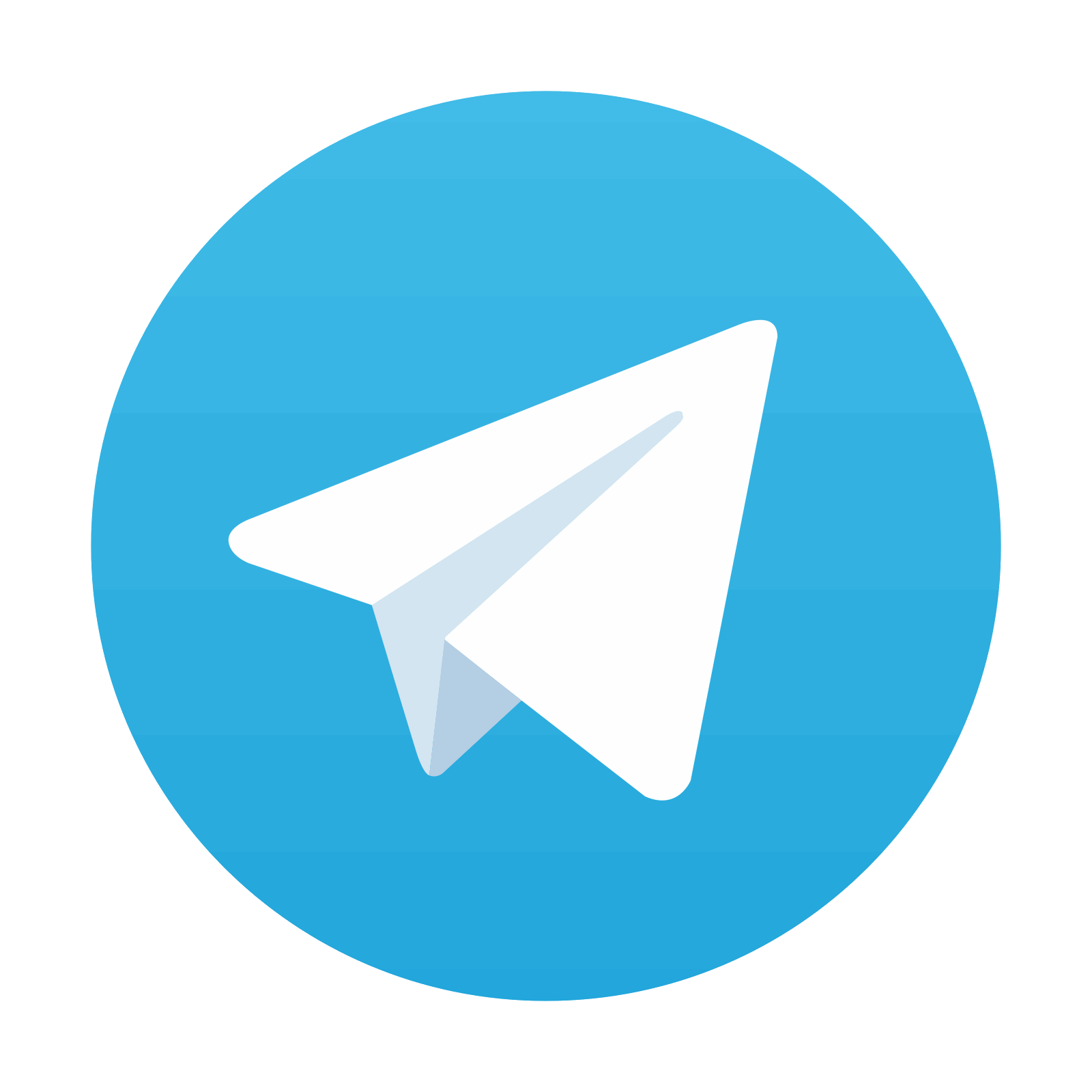
Stay updated, free articles. Join our Telegram channel

Full access? Get Clinical Tree
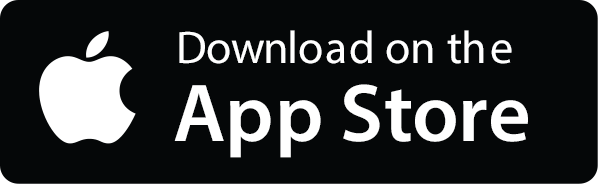
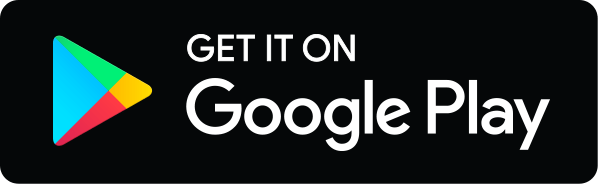
