Edema in the Lower Extremity: Pathophysiology and Differential Diagnosis
JEFFREY S. BORER
BRIAN SHAFFER
ROCK C.J. POSITANO
LORETTA CACACE
Injuries of the foot are commonly associated with edema, a concomitant of local inflammation and mechanical obstruction to local venous blood flow. Edema also can result from a variety of systemic conditions, some of which are potentially life threatening. However, “swelling, like fever, is not a disease itself but a sign of an underlying disorder.”1 Therapy, both in its form and urgency, must be targeted to the causative process, not to the physical sign. The objective of this chapter is to review the pathophysiology of edema, differentiate the pathophysiologic characteristics associated with local injury from those associated with other diseases, and present the differential diagnosis of peripheral edema, with particular reference to the clinical signs and symptoms by which the edema of localized injury might be differentiated from that resulting from other causes.
This chapter represents an update of a chapter we published earlier (Shaffer B, Borer JS. Edema and foot injuries: pathophysiology and differential diagnosis. In: Ranawat CS, Positano RG, eds. Disorders of the Heel, Rearfoot, and Ankle. New York, NY: Churchill Livingstone; 1999:116-124). Much of that material is integrated and incorporated herein by direct quotation or paraphrasing (with permission of the publisher), with addition of new material as appropriate.
Pathophysiology of Peripheral Edema
Ultimately, edema results from transudation of fluid across the capillary or proximal venular wall. Such fluid movement results from an imbalance between capillary permeability and the flow of fluid through the capillaries. Fluid flow is determined by capillary hydrostatic and oncotic pressures2,3,4; capillary permeability is a function of the performance of the endothelial cells (through which solute and fluid can move) and size of the spaces between these cells (which also serve as pathways for fluid and solute egress).3,5
Interstitial and Capillary Hydrostatic Pressure
The usual cause of abnormal capillary hydrostatic pressure is increased total intravascular volume. Concomitant with the increased intraluminal pressure, the pressure gradient between the capillary lumen and the interstitium must rise, at least transiently, precipitating fluid transudation through interendothelial interstices. Most commonly, intravascular volume expansion results from abnormal sodium retention, as is found in the setting of chronic heart failure (CHF). In CHF, cardiac output is subnormal (low-output CHF) or relatively inadequate (high-output CHF). In either case, renal perfusion is compromised by a combination of neural and humoral responses triggered by signals emanating from a series of baroreceptors and chemoreceptors; these signals are influenced by cardiac output.3,4,6,7,8 Subnormal renal perfusion (plasma volume deficiency) increases the release of renin through stimulation of the β-adrenoceptors in the juxtaglomerular cells of the renal cortex, which enhances the production of angiotensin I. In the bloodstream, angiotensin I is rapidly converted to angiotensin II. Angiotensin II causes increased secretion of the salt-retaining hormone aldosterone from the adrenal cortex, renal vasoconstriction (specifically, constriction of efferent renal glomerular arterioles), and sodium reabsorption from the proximal convoluted tubule. Angiotensin-mediated vasoconstriction increases blood pressure and decreases renal perfusion, thus increasing filtration fraction and proximal tubular reabsorption of water and sodium in the kidney. Aldosterone acts on the distal convoluted tubule and collecting duct to reabsorb sodium. Thus, the result of activating the renin-angiotensin-aldosterone system is increased plasma volume potentiating the development of edema.6,7,8,9,10
Intravascular volume also can be expanded iatrogenically by intake of large fluid volumes at a rate that exceeds renal excretory potential.11 Effective capillary volume can be increased even without an increase in total vascular volume by relaxation of precapillary arteriolar muscular sphincters, as occurs iatrogenically by administration of certain vasodilators, like nifedipine.12 Similarly, capillary volume, and concomitant intracapillary hydrostatic pressure, can be increased by obstruction to venous flow, as by venous thrombosis. Such obstruction can prevent egress of fluid from the capillary lumen into the venular lumen while forward flow continues from the precapillary arterioles, resulting in abnormal filling of the capillary lumen. Similarly, in CHF, increased central venous pressure is reflected backward throughout the venous system, and the effects of relative obstruction to outflow potentiate those resulting from the increase in total vascular volume.6,11,13
Interstitial and Capillary Colloid Oncotic (Osmotic) Pressure
Capillary osmotic pressure primarily is a function of plasma protein concentration and, most specifically, the concentration of the relatively small protein, albumin. Thus, capillary osmotic pressure is reasonably approximated as capillary oncotic pressure. Generally, plasma proteins do not pass through the capillary endothelial cells or the intercellular capillary pores. Therefore, the osmotic effects of the intraluminal plasma albumin counteract the effects of abnormal hydrostatic pressure and even tend to promote resorption of interstitial fluid. Plasma oncotic pressure is diminished by various systemic, renal, and hepatic diseases (e.g., nephrotic syndrome, malnutrition, cirrhosis, loss of protein in the gastrointestinal tract, severe catabolic state).6,11
The effects of intravascular proteins are opposed by the interstitial colloid concentration. Tissue colloid osmotic pressure can be altered by extravascular protein accumulation as a result of abnormal capillary permeability or obstruction of flow within the lymphatic system.3,6,13 Physiologically, the lymphatics remove the fluid, which is filtered by normal capillaries. Thus, normally, there is a slight flow gradient from the arterial to the venous side of the capillaries; the fluid and protein, which enter the capillaries from the arterioles, exceed the capacity of the capillaries, which filter the excess into the interstitial space. Some of this excess is resorbed into the capillary as this vessel communicates with the more compliant venule. However, the amount filtered generally exceeds the efficiency of the resorption process. The excess is removed directly by the lymphatic vessels, which drain the interstitial space, thus enabling tissue homeostasis to be maintained for water and solute.3,4,5,6,13 The lymph is returned to the venous system via anastomoses between lymphatic vessels and the larger central veins.
Capillary Permeability
Generally, only noncolloid solutes permeate the capillary wall freely. Injury to the capillary wall from chemical, bacterial, immunologic, thermal, or mechanical sources (as is characteristic of inflammation) can increase capillary transmural permeability to larger molecules, like albumin and other plasma proteins. Thus, for example, in extreme cases of capillary endothelial cell hypoxia (as might be seen with the ischemia of arteriolar or arterial obstruction or in the context of poison gas, burns, or allergic reactions), increases in permeability of capillaries and small venules can allow fluid, high in protein content, to enter the extravascular space within the injured tissue. Such abnormalities in microvascular permeability generally are not important factors in the pathophysiology of the common generalized edematous states, but can be important in the genesis of localized edema.3,6,11
Several mechanisms may underlie pathologic alterations in capillary permeability. During acute and non-necrotizing injury to the skin, release of endogenous autacoids (e.g., histamine, serotonin), presumably most often from mast cells, can cause arterial constriction, possibly associated with capillary injury (itself potentially affecting capillary endothelial function and integrity). A period of constriction generally is followed by arterial dilation, which increases flow into the capillaries. Release of autacoids appears to be triggered by local release of calcium ions from injured cells. The effects of the autacoids are highly selective, resulting in separation of the lateral borders only of the endothelial cells lining the postcapillary venules of 10 to 30 µm in diameter and not, strictly speaking, of the capillaries themselves. Indeed, the effect can be quite marked: the width of the intercellular clefts can be greatly enlarged, sufficiently even to accommodate 7 to 10 µm of formed blood elements.14 Although the microvascular basement membrane, which limits movement of colloidal particles (e.g., lipoproteins), is not affected by autacoid release, this barrier can be traversed by plasma proteins and smaller solutes, allowing edema formation.14
Alteration in the function of the endothelial cells themselves also can be important in the pathophysiology of edema formation. Endothelium lining the microvasculature forms the critical barrier controlling the exchange of molecules between blood and interstitial fluid. Interaction of blood with the endothelial cell surface (the glycocalyx) can restrict or increase transendothelial transport of specific ligands. Excessive movement of osmotically active molecules into the interstitium can promote edema formation, whereas their transport out of the interstitial space can have the opposite effect. Because these endothelial transport mechanisms depend on endothelial expression of cell surface glycoproteins, regional variations in endothelial transport can be expected and may be useful in developing tissue-directed drug therapies, which might limit edema formation in certain settings.15
The composition of the serum also can affect capillary permeability. This influence depends on the capacity of serum components to affect the configuration of the electrical charge of the glycocalyx. Normally, the net negative charge configuration at the cell surface tends to restrict transcapillary transport of polyanionic molecules to a greater degree than neutral or polycationic molecules. Endothelial cell surface binding of certain plasma anionic macromolecules, such as albumin and orosomucoids, increases the charge negativity at the glycocalyx, resulting in greater polyanion exclusion.15 In addition to its effects via surface charge alteration, serum albumin acts as a steric molecular “filter” that can resist the transport of water, small solutes, and macromolecules across the microvascular wall.15 Thus, reduction in serum proteins can promote edema formation both by reducing serum oncotic pressure and minimizing the electrostatic and physical
effects that retard movement of osmotically active molecules into the extravascular space.
effects that retard movement of osmotically active molecules into the extravascular space.
Differential Etiologic Diagnosis of Peripheral Edema
The pathophysiologic processes described previously can occur in various combinations among the many disease processes characterized by peripheral edema. Clues to the underlying cause can be inferred from associated clinical symptoms and physical signs. In approaching the differential diagnosis of edema, a useful framework for separating localized processes from systemic or diffuse diseases can be based on consideration of the following characteristics:
1. History
a. Known potential causes or contributing factors (recent trauma, surgery, concurrent illness, drug therapy)
b. Temporal factors
1. Rapidity of onset (gradual, more commonly associated with systemic diseases, versus sudden, more commonly associated with localized processes)
2. Temporal pattern (short duration/first episode, chronic, recurrent, cyclic; note that variations in edema during 24-hour periods have limited diagnostic value because, irrespective of cause, peripheral edema is greatest in dependent regions and, therefore, typically is greater in feet and ankles during the daytime, when upright posture is most common)
c. Symptoms (painless, more common in systemic diseases, versus painful, very uncommon in most systemic diseases)1,2,4,6,11
2. Physical signs
a. Laterality (unilateral, more common in localized processes, versus bilateral, more common in systemic diseases)
b. Condition of overlying skin (taut, thick, fibrotic skin generally is associated with a chronic process, more likely a result of systemic disease or venous insufficiency than musculoskeletal condition)
c. Density of edema (edema that is relatively low in protein commonly is soft and “pitting,” typical of CHF or hypoproteinemia; edema that is relatively high in protein content or involves extensive subcutaneous fibrosis generally is nonpitting, as in pretibial myxedema)
d. Location (anasarca, edema plus ascites, simultaneous upper and lower extremity edema, and other generalized patterns suggest systemic diseases; localized edema, even if bilateral, may be more closely associated with a localized process)1,2,4,6,11
Laterality of edema is perhaps the most efficient initial discriminator of systemic versus localized processes. Therefore, the etiologic differential diagnosis of peripheral edema, presented next, is keyed to this characteristic, as modified from the classification schemes of Ruschhaupt and Graor 2 and Young.1
Bilateral Edema
Bilateral edema commonly begins simultaneously in both legs at feet and ankles and proceeds symmetrically up the legs.2 Possible causes include the following:
Chronic Heart Failure
History of potential causes/contributing factors: hypertension, angina, or myocardial infarction; valvular diseases; cardiomyopathy; etc. Symptoms: dyspnea on exertion, orthopnea, paroxysmal nocturnal dyspnea; associated signs: tachypnea, rales, rhonchi, distended neck veins, tachycardia, hepatomegaly, ventricular gallop, heart murmur, etc.1,2,11 Edema of CHF typically is soft and easily pitting, occurs predominantly in dependent parts of body, and, therefore, diminishes in legs after a period of recumbency.1,2,11 CHF edema can be distinguished from other forms of dependent edema by the presence of engorged cervical veins, particularly if evidence of diffusely elevated systemic venous pressure (e.g., hepatomegaly) also is present.6
Pulmonary Hypertension
Causes of pulmonary hypertension are left-sided heart failure, chronic obstructive pulmonary disease (COPD), and sleep apnea.16 Sleep apnea is an underrecognized cause of edema and can cause hypertension due to sympathetic nervous system overactivity secondary to intermittent hypoxia.17 Pulmonary hypertension has been reported to be common in those with obstructive sleep apnea, and pretibial edema is a common sign of pulmonary hypertension in those with sleep apnea.18 Studies suggest that patients who are at risk for pulmonary hypertension and over the age of 45 with nonspecific leg edema should undergo echocardiogram in order to rule out obstructive sleep apnea.16
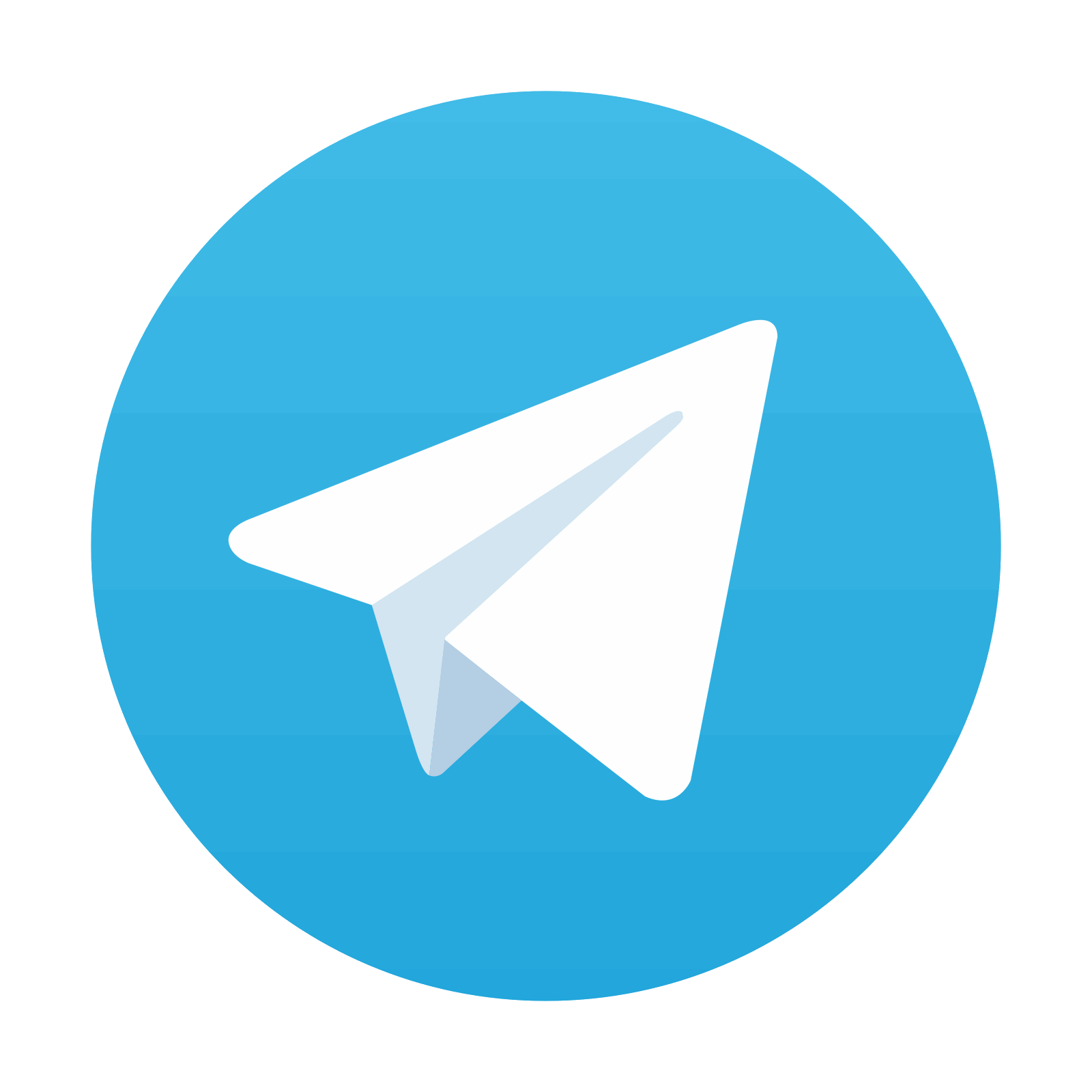
Stay updated, free articles. Join our Telegram channel

Full access? Get Clinical Tree
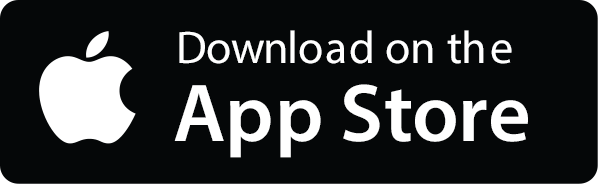
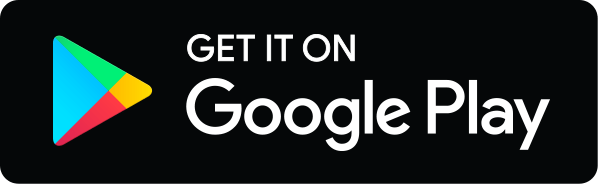
