Introduction
Clear aligners (CAs) have recently become popular and widely used orthodontic appliances. Research on CA biomechanics has become a focal point in orthodontics to improve the efficiency of CA treatment and address challenging issues, such as extraction. The biomechanical characteristics of CAs in space closure have been reported. However, previous studies have mainly focused on static biomechanical analysis that cannot demonstrate the dynamic biomechanical changes in CAs during space-closing. Given that these biomechanical changes can be significant and have considerable clinical value, this study aimed to investigate these characteristics.
Methods
Sequential extraction space-closing models were derived from included patient data and refined using modeling and CA design software. A finite element analysis was performed to obtain biomechanical raw data. This study introduced a dual coordinate system and space geometry analysis to demonstrate the biomechanical properties accurately.
Results
As space closure progressed, the instantaneous tooth displacements increased, indicating an enhanced space closure force because of the increased strain in the CA extraction area. Meanwhile, the central axis of rotation of the anterior teeth continuously moved toward the labial-apical direction, showing a gradually enhanced vertical and torque control effect.
Conclusions
During space closure, CAs undergo specific biomechanical changes, including increased contraction and control forces on both sides of the gap. These biomechanical effects are beneficial to alleviate the roller coaster effect gradually. Meanwhile, more reasonable staging design strategies can be proposed on the basis of this biomechanical mechanism.
Highlights
- •
Created sequential models to reveal mechanics change of clear aligners in space closure.
- •
We used dual reference coordinate systems to display 3-dimensional tooth displacement.
- •
We proposed space geometry codes for calculating the central axis of rotation for teeth.
- •
This study showed the stress interruption effect of clear aligners in patients with an extraction.
Clear aligners (CAs) have gained popularity recently because of their unique benefits, such as comfort, esthetics, and hygiene. , However, as a novel appliance, the biomechanics of CAs are completely different from that of fixed appliances. Thus, biomechanical research on CAs has become a crucial area of focus in orthodontics to continuously improve treatment efficiency.
CAs are designed to use the elasticity of polymer materials to progressively move the teeth to a predetermined position. Thus, CAs are required to possess both excellent retention and mechanical properties. The retention between the CA and the tooth is determined by the tooth movement pattern and morphology. For instance, correcting rotated anterior teeth is easier than correcting premolars, which have rounded shapes. Therefore, CAs exhibit varying orthodontic efficiencies in treating various malocclusions, and tooth movement usually manifests as tipping movements. Notably, in addition to retention issues, CAs also have mechanical deficiencies. Currently, CAs are made of viscoelastic materials. Unlike super-elastic materials, such as nitinol springs, viscoelastic materials will undergo creep and stress relaxation, leading to a constant loss of elasticity over extended periods of use. Therefore, not only is it difficult for CAs to fully move teeth to their target position, but deviations are magnified in patients with significant movements, such as extraction. Several recent studies have concluded that CAs are more suitable for simple nonextraction. Because extraction usually requires deep overbite correction, root control, and arch expansion, they impose more stringent requirements on orthodontists. , ,
Nowadays, to manage malocclusion and improve facial esthetics, the proportion of patients that require extraction has reached 30%. For these patients to benefit from CA treatment, several methods have been used to compensate for the CA control deficiency, such as (1) adding extra overcorrection in the target position, (2) designing torque compensation in anterior teeth and anchorage preparation in posterior teeth, (3) setting rational attachments to ensure better retention, and (4) using a long-power arm to retract the anterior teeth when strong anchorage is present. To accurately set compensation, orthodontists need to understand how control deficiencies occur during space closure. In our previous studies, we demonstrated the biomechanical characteristics of CA in space closure under different anchorages and elaborated on the biomechanical differences of CA in bimaxillary space closure, proposing reliable overcorrection strategies for CA extraction. However, simply knowing the overcorrection requirement in the target position is still insufficient, as space closure is a dynamic process that may result in changes in the overcorrection requirement. This effect could be significant because the space closure force is generated by the CA deformation in the extraction area, and the local strain of the CA determines the orthodontic force on both sides of the extraction space. At the beginning of space closure, the CA extraction area is large and soft because of the low strain (percentage), so the closing force and control force on both sides of the space are low. We call this phenomenon the stress interruption effect. When the extraction space is reduced, the CA extraction area becomes smaller, and at the same time, it becomes rigid because of the high strain, so both the closing and control forces are strengthened, and the stress interruption effect is attenuated. This dynamic change in CA has not been reported or discussed before, but it could certainly affect CA design strategies and hold considerable research value.
This study aimed to demonstrate the dynamic biomechanical effects of CAs throughout the extraction space closure. We constructed sequential space-closing models on the basis of cone-beam computed tomography (CBCT) and intraoral scan data to achieve this. A 0.2 mm space closure force was generated in finite element analysis (FEA), and then the instantaneous stress distribution and tooth displacement results were obtained to elaborate the biomechanical effects. Compared with clinical research, FEA is more stable, reproducible, and can eliminate unnecessary confounding factors, making it suitable for addressing complex biomechanical problems. In this work, several methodological improvements were made to make FEA better suited for CA research. In addition to a global coordinate, local coordinates were set for each tooth according to its mesiodistal, labiolingual, and vertical directions, allowing for a clear 3-dimensional (3D) display of the control effects of each tooth. A set of spatial geometry codes was provided to facilitate research on the biomechanical mechanism, allowing researchers to easily locate the rotation center axis of each tooth and accurately analyze tooth movement patterns. We also provided code for the coordinate system conversion, with which researchers can observe and analyze the FEA results from various perspectives. This study comprehensively demonstrates the dynamic biomechanical effects of CA during the space-closing process, discusses its clinical significance and rational coping strategies, and provides a scientific and useful research method for this field.
Material and methods
A healthy orthodontic patient’s data was selected from the West China Stomatology Hospital on the basis of the following inclusion criteria: (1) permanent dentition, (2) normal tooth morphology, (3) no obvious crowding, (4) symmetrical arch with basically normal tooth torque and occlusal curve, (5) no periodontal disease, and (6) complete CBCT and intraoral scan data. Systemic diseases that impair bone development were used as an exclusion criterion. This study was approved by the Ethics Committee of the West China Stomatology Hospital of Sichuan University.
The maxillary model was constructed from CBCT and intraoral scan data. The maxillary bone and dentition were separated from the CBCT data via threshold segmentation using Mimics Research software (version 19.0; Materialise NV, Leuven, Belgium). With Geomagic wrap 2017 (Raindrop Geomagic, 3D systems, NC), the acquired 3D geometric models could be enhanced and smoothed. To further enhance the precision of dentition modeling and construct a standard first premolar extraction maxillary model, intraoral scan data were used to modify the CBCT data. Briefly, the intraoral scan data was imported as stereolithography (STL) files into OrthoAnalyzer (3Shape, Copenhagen, Denmark), and then the dentition was aligned on the basis of Andrew’s normal occlusion to achieve a standard dentition. The aligned intraoral scan model replaced the CBCT dentition, establishing a high-precision maxillary model. The first premolars were sequentially removed to generate the first premolar extraction model. Periodontal ligaments were constructed by uniformly extending 0.25 mm outward from the tooth root. CAs and attachments were designed in Appliance Designer (3Shape, Denmark), with the CAs generated by making 0.5 mm external offsets from the dental crowns ( Fig 1 , A ). Finally, the constructed components were assembled in Unigraphics NX (Siemens PLM Software, Plano, Tex) to form the research model ( Fig 1 , B ).

The automated teeth rehearsal tool of OrthoAnalyzer was used to construct sequential extraction space-closing models. The first premolar extraction model was imported into the software, and a final position was set with the extraction space completely closed. Then, by setting a space-closing rate of 0.2 mm per step, a simulated 36-step space-closing procedure was automatically generated on the basis of the initial and final position of the dentition ( Supplementary Fig 1 ). Notably, the dentitions created during space closure did not differ in form from the initial dentition, except for the residual extraction space. This means that the generated 36-step models maintained the same dentition morphology, relative teeth position within the anterior/posterior teeth, and the occlusal curve, greatly eliminating unnecessary confounding factors. To further investigate the dynamic biomechanical effects of the space closure, 4 sequential representative groups were selected: (1) the initial step-0 model with a tooth extraction gap of 7.2 mm, (2) the step-10 space-closing model with a 5.2 mm residual space, (3) the step-20 space-closing model with a 3.2 mm residual space, and (4) the step-30 space-closing model with a 1.2 mm residual space. All 4 groups had the same space contraction area and direction ( Fig 1 , C) .
The assembled models were discretized into 3D finite element models and meshed by tetrahedral elements (C3D10) in ABAQUS 6.14 (Dassault Systemes, Providence, RI). The discretization parameters are listed in Table I . Model components were set as isotropic, homogeneous, and linearly elastic properties. The material properties were set according to previous literature reference ( Table II ). To prevent bodily motion while the force was applied, fixed boundaries were set at the base of the maxilla. A contact friction coefficient (μ) of 0.2 was added between the CA and the dentition. As described in previous studies, the thermal contraction method was used to apply an adequate space-closing force. Briefly, by altering the material temperature and linear expansion coefficient parameters, a 0.2 mm contraction was precisely achieved in a 1 mm area in the extraction region, achieving a space closure effect ( Supplementary Fig 2 ). Finally, after the loading configuration was applied, the FEA was performed using Abaqus software (version 6.14, Abaqus; Dassault Systemes Simulia Corp, Providence, RI), and the nodal displacements and von Mises stress distributions were extracted for further analysis.
Group | Total no. of nodes | Total no. of elements |
---|---|---|
Step-0 | 815,891 | 453,063 |
Step-10 | 818,361 | 447,677 |
Step-20 | 823,659 | 449,958 |
Step-30 | 970,781 | 543,164 |
Material | Young modulus, E (MPa) | Poisson ratio, v |
---|---|---|
Alveolar bone | 1.37 × 10 4 | 0.30 |
Teeth | 1.96 × 10 4 | 0.30 |
Periodontal ligament | 0.69 | 0.45 |
Clear aligner | 528 | 0.36 |
Attachment | 1.25 × 10 4 | 0.36 |
To quantitively analyze the tooth movement pattern and clarify the results, 2 coordinate systems were used in this work. The global coordinate was set on the basis of the occlusal plane to analyze tooth vertical movement ( Fig 2 , A ). Local coordinates were set at each tooth to calculate their 3D movements. Specifically, 2 local coordinates were located separately at the body center of the clinical crown and root apex point (or center of root apex points for multirooted teeth). The x, y, and z-axes were consistent with the labiolingual, mesiodistal, and tooth long axis direction of each tooth ( Fig 2 , B ). Based on the 2 coordinate systems, a tooth movement table could be summarized in detail, consistent with the current CA design system, such as the Clincheck of Invisalign (Align Technology, Santa Clara, Calif), providing considerable practical reference values.

The center of rotation is a significant indicator of tooth tipping movement. In previous orthodontic biomechanical research, the center of rotation was usually simplified as a point in 2-dimensional space. However, it is an axis in 3D space ( Fig 2 , C ). To accurately analyze the tipping movement of each tooth in 3D space, this work provided a spatial geometry algorithm to locate the center axis of rotation for the first time. An ordinary least squares (OLS) mathematical model was used to fit the center axis of rotation. Ten randomly selected node displacements were extracted from the tooth surface and then imported into Halcon (version 23.11; MVTec Software, Munich, Germany) to calculate the equation of the center axis of rotation ( Fig 2 , D ). With the equation of the center axis of rotation, the distance between the central axis of rotation and the crown and the root apex could be easily calculated in MetLab (version 9.12; The Mathworks, Natick, Mass) ( Fig 2 , E ). In total, we used spatial geometry to calculate the distance to the center of rotation and that the algorithm obtained by the OLS mathematical model is accurate and reliable. The specific algorithm content, usage, and verification methods are provided in Supplementary Figures 3-4 .
Statistical analysis
Descriptive analysis was used to display the regular biomechanical effects among research groups, whereas Pearson product-moment correlation analysis was used to test the correlations between the space closure process and the biomechanical effects. GraphPad Prism 9 (version 9.0; GraphPad Software, La Jolla, Calif) was used for the statistical analysis.
Results
The same 0.2 mm contraction force was applied in the 4 groups with different residual extraction spaces. The FEA results are presented in Figure 3 . The contour diagram in Figure 3 , B displayed the overall displacements, which demonstrated that the teeth displacements were constantly increasing during the space-closing progress. The vector diagram in Figures 4 , C and D further intuitively depicts the displacement directions and distances in the anterior and posterior teeth. All 4 groups presented a similar tooth movement pattern: the anterior and posterior teeth tipped toward the extraction spaces, exhibiting a roller coaster effect. With the space-closing, both anterior and posterior teeth maintained similar displacement direction but presented increasing displacement distance. To further quantitively analyze this phenomenon, 3D tooth displacement data was extracted from Table III , showing that the displacements in all 4 groups exhibited regular increases. Although the instantaneous tooth displacement commonly increased in all directions with space closure, the most apparent displacement consistently occurred in the sagittal direction that coincided with the direction of the space contraction. Therefore, the vertical and sagittal displacement changes were summarized in Figure 3 , E for further analysis. The sagittal displacement and tipping were consistent with the qualitative analysis. Both the anterior and posterior teeth exhibited increasing instantaneous displacement during the space closure, but the magnitude of the increase slowed down during this process. It should be noted that, at the end of the space closure, the extrusion of the anterior teeth (especially the lateral incisor) and the intrusion of the molars both decreased, indicating a trend toward better vertical control of CA.


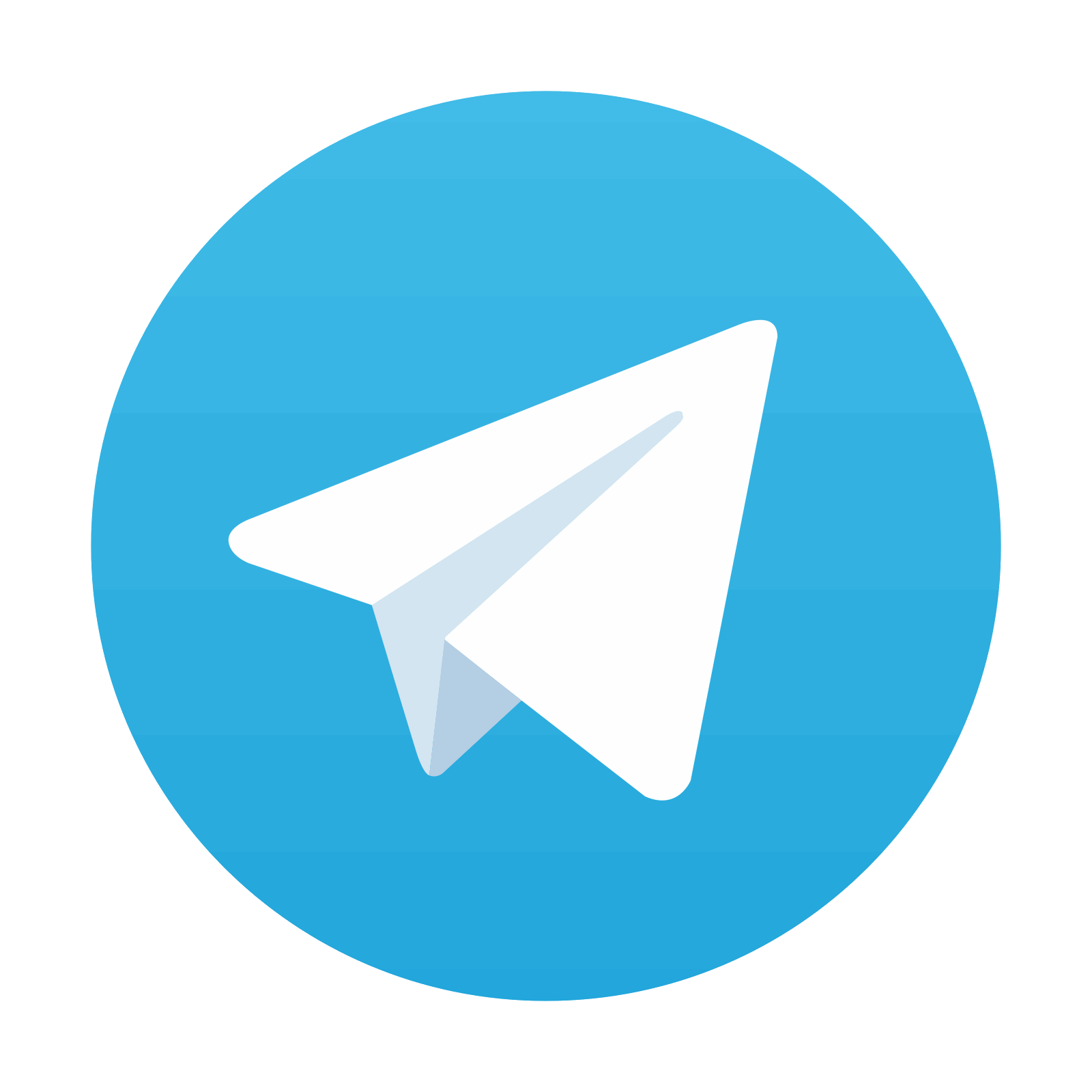
Stay updated, free articles. Join our Telegram channel

Full access? Get Clinical Tree
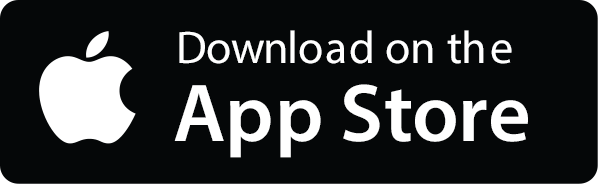
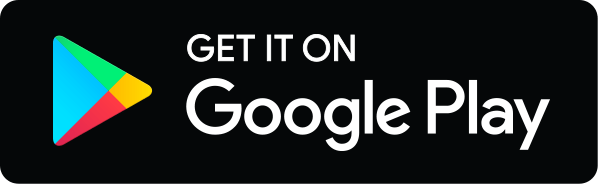
