The primary role of neuroimaging in the clinical context of sports-related concussion is the exclusion of a more severe, unsuspected intracranial injury. Computed tomography remains the test of choice for this purpose. Magnetic resonance imaging is more commonly used as a secondary test for the investigation of persistent symptoms. New imaging techniques are currently being developed to detect the molecular and cellular changes underlying concussion that are invisible with standard structural imaging. In the future, these techniques may be used as tools for directing rehabilitation after concussion and aiding in the decision of when it is safe for an athlete to return to play.
Concussion, as defined by the Third International Conference on Concussion in Sport, is a clinical diagnosis ( Box 1 ). To paraphrase, “concussion” is a transient neurologic impairment resulting from an impact to the head or body. There is no detectable structural injury on standard neuroimaging studies.
Concussion is defined as a complex pathophysiologic process affecting the brain, induced by traumatic biomechanical forces. Several common features that incorporate clinical, pathologic, and biomechanical injury constructs that may be used in defining the nature of a concussive head injury include:
- 1.
Concussion may be caused either by a direct blow to the head, face, neck, or elsewhere on the body with an “impulsive” force transmitted to the head.
- 2.
Concussion typically results in the rapid onset of short-lived impairment of neurologic function that resolves spontaneously.
- 3.
Concussion may result in neuropathologic changes but the acute clinical symptoms largely reflect a functional disturbance rather than a structural injury.
- 4.
Concussion results in a graded set of clinical symptoms that may or may not involve loss of consciousness. Resolution of the clinical and cognitive symptoms typically follows a sequential course; however, it is important to note that in a small percentage of cases postconcussive symptoms may be prolonged.
- 5.
No abnormality on standard structural neuroimaging studies is seen in concussion.
The primary role of neuroimaging in the context of concussion is the exclusion of a more serious, unsuspected diagnosis, such as an epidural hematoma. A small but significant proportion of patients presenting with a clinical history consistent with concussion have an intracranial injury or skull fracture that requires further management. Computed tomography (CT), and to a lesser extent magnetic resonance imaging (MRI), are the imaging modalities of choice for this purpose.
The challenge facing sports-related concussion, particularly in adolescents, is to determine when it is safe to return to play. Athletes with a history of concussion are at increased risk for subsequent concussions compared with teammates. There may also be a temporal window of increased vulnerability during which time even a mild head injury results in a potentially fatal outcome, termed “second impact syndrome.” Symptoms are generally used as a guide for return to play decisions, but these may not be sensitive enough or there may be incentive or social pressure for an athlete to deny symptoms to play. There is a potential role for new, more sensitive imaging techniques directed at the underlying pathophysiology of concussion to help guide rehabilitation and return to play decisions.
This article first focuses on the current role of imaging for the exclusion of unsuspected intracranial injuries in the clinical context of concussion. Emerging techniques are then discussed in the context of the current understanding of the molecular and cellular pathophysiology underlying concussion. Throughout this review, evidence pertaining to adolescent sports-related concussion is considered primarily. As our understanding of the pathophysiology of concussion evolves, it is clear that concussion represents the mild end of the spectrum of traumatic brain injury (TBI) and there is significant overlap between the terms “concussion” and “mild TBI” (mTBI). As such, evidence from mTBI or more severe injuries is also considered when appropriate.
Diagnosing concussion: excluding a more severe injury
Epidemiology of Intracranial Injuries
The Centers for Disease Control and Prevention estimates that 135,000 persons aged 5 to 18 years are treated for sports-related TBI in hospital emergency departments in the United States annually. Most patients will have suffered only a mild head injury and are neurologically normal on presentation, consistent with the diagnosis of concussion. However, a small but significant proportion of these harbor intracranial injuries evident on neuroimaging that require further management.
The frequency of traumatic intracranial injuries in adolescents after minor, sports-related head trauma is not known. Multiple studies have measured the rates of intracranial injuries (including skull fractures) on CT and subsequent neurosurgical interventions in patients presenting to emergency departments after minor head injury and with a normal Glasgow Coma Scale (GCS) score of 15 ( Table 1 ). In the general population, rates of detected intracranial injuries ranged from 3.3% to 34% and rates of intervention, usually neurosurgical, ranged from 0.3% to 1%. Studies involving only pediatric patients tended to have higher rates of intracranial abnormalities and interventions. Two meta-analyses concluded the overall rates of intracranial injury are approximately 5% to 8%, rates of neurosurgical intervention are less than 1%, and approximately 1 in 1000 patients will die.
Study, Year | Ages (Mean) | No. | LOC (%) | Positive CT | Intervention |
---|---|---|---|---|---|
Haydel & Shembekar, 2003 | 5–17 (13) | 175 | 100 | 14 (8%) | 1 (0.6%) |
Simon et al, 2001 | <16 (7) | 499 | 46 a | 120 (24%) | 5 (1%) |
Stein & Ross, 1992 | NA (NA) | 1117 | 100 | 73 (13.2%) | NA |
Haydel et al, 2000 | ≥3 (36) | 1429 | 100 | 93 (6.5%) | 6 (0.4%) |
Smits et al, 2005 | ≥16 (41 a ) | 2462 | 61 a | 185 (7.5%) | 10 (0.4%) |
Thiruppathy & Muthukumar, 2004 | All (NA) | 285 | 40 | 98 (34%) | 18 (6%) |
Nagy et al, 1999 | All (34) | 1170 | 100 | 39 (3.3%) | 4 (0.3%) |
a Statistic calculated from entire study sample, including patients with GCS 13–15.
It should be noted that although sports-related head injuries were included in these studies, the predominant mechanism of injury was motor vehicle collision, likely exposing the patient to greater forces than that seen in sports. The two pediatric studies required a “nontrivial” or “high-risk” mechanism. Furthermore, several studies included only patients with head injuries resulting in loss of consciousness. As such, these studies are complicated by selection bias, likely overestimating the true rate of unsuspected intracranial injury in patients presenting with mild head trauma. However, the devastating potential of these injuries makes even a low rate significant.
Radiography
Plain radiographs of the skull have been used in the assessment of head injury to identify fractures as a marker for intracranial injury. The advantage of radiographs is that they are cheap and ubiquitously available. Their great disadvantage is their lack of sensitivity, and since the advent of CT, skull radiographs have been essentially abandoned.
The usefulness of plain radiography of the skull in patients presenting with concussion is extremely limited. Radiographs can identify skull fractures, but not underlying brain injury. Patients may have a fracture without underlying intracranial injury or they may have a serious intracranial injury without fracture. Teasdale and colleagues examined 8051 patients, 3491 children (2–14 years of age) and 4560 adults, who presented to Scottish emergency departments between 1974 and 1984 with a head injury and a GCS of 15. Of the patients with clinically diagnosed, intracranial hemorrhage requiring neurosurgical intervention, only 18 (53%) of 34 children and 86 (71%) of 121 adults had skull fractures diagnosed by radiographs. Furthermore, skull fractures were detected in 61 (41%) of 147 of adults and 48 (73%) of 66 of children who did not require intervention. Thus, skull radiographs may be falsely reassuring if they are normal or may lead to unnecessary intervention if they are abnormal.
Other studies have shown similar results, including a meta-analysis that estimated the sensitivity of skull radiographs for the detection of intracranial hemorrhage in minor head injury to be 38%, using CT of the head as the reference standard. As a result, current guidelines for the management of pediatric or sports-related concussions ignore or discourage the use of radiography as a test for intracranial injury in favor of CT.
Computed Tomography
CT is the imaging modality of choice for initial screening of patients presenting with a history of mild head injury to exclude a more serious intracranial injury. Compared with standard MRI, CT is more widely available in terms of both locations and hours of operation, and it is significantly faster, requiring only seconds for a head CT. Aside from minimizing the time the patient is in the scanner, a faster examination limits the potential for motion artifact, a significant consideration in disoriented patients or young children.
Modern CT scanners move the patient past a rotating x-ray tube (<1 s per rotation), acquiring a helical dataset of x-ray attenuation measurements. Multidetector CT scanners have multiple rows of detectors (eg, 4, 16, 64, 128, and so forth, sometimes referred to as “slices”) along the z-axis (craniocaudal), allowing greater coverage per rotation, higher resolution, and faster scan times, although generally at a cost of more radiation exposure. A computer processes the dataset and generates gray-scale representations of x-ray attenuation. Attenuation is measured in Hounsfield units (HU), where water is set at 0 and greater attenuation (eg, bone or contrast material) results in positive values and lesser attenuation (eg, air) results in negative values (−1000 HU for air).
Modern CT scanners can achieve a resolution of fractions of a millimeter in all planes, although images of the head are most commonly reconstructed in the axial plane with a slice thickness of 5 mm. The original dataset can often be used to create reformations in any plane or even three-dimensional representations.
CT is most sensitive for the detection of acute hemorrhage and fractures ( Fig. 1 ). In the brain, white and gray matter measure approximately 40 and 35 HU, respectively, whereas acute hemorrhage measures 50 to 90 HU. Multiplanar and three-dimensional reformations increase sensitivity for fractures and lesions parallel to the standard, axial plane, such as thin subdural hematomas near the skull apex ( Figs. 2 and 3 ).
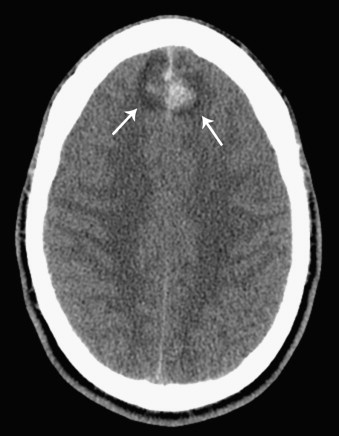
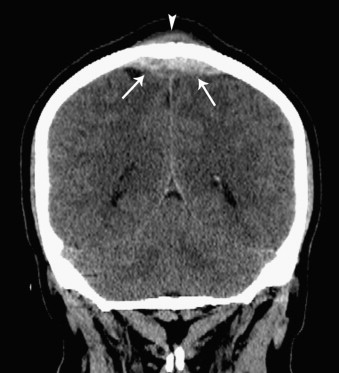
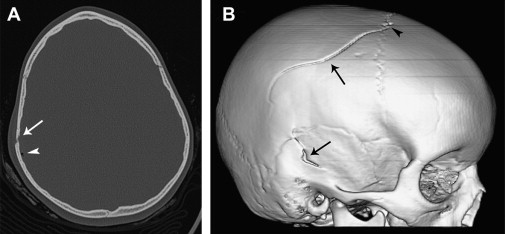
Ionizing Radiation
CT uses ionizing radiation (x-rays), which carries its own risks and deserves mention. The energy imparted in biologic tissues can be harmful in two ways. First, sufficiently high radiation exposure directly kills cells, resulting in skin erythema (burn), epilation (hair loss), or necrosis. These effects are threshold dependent and the radiation dose needed for even minor skin effects is extremely uncommon with CT.
More relevant to CT are stochastic effects, representing the likelihood of radiation exposure to induce cancer at the molecular level. These effects are generally assumed to be linearly related to dose and not threshold dependent: a single photon has the theoretical potential to induce a cancer-causing mutation. Effective dose (ED), measured in sieverts (Sv), attempts to estimate this risk by taking into account absorbed radiation dose (measured in gray [Gy]) and the radiosensitivity of the exposed organs.
A routine head CT has an ED of approximately 2 mSv (range, 0.3–6 mSv). Table 2 compares the median ED of common medical imaging tests and equivalent exposure from naturally occurring background radiation and a two-view chest radiograph series. For a variety of reasons, ED also increases with decreasing patient age. King and colleagues reported EDs for a head CT at a regional children’s hospital of 1.7, 2.1, and 2.4 mSv in age groups of 10 to 14, 4 to 9, and 0 to 3 years, respectively.
Examination | ED (IQR) | Background Radiation | Chest Radiographs |
---|---|---|---|
Head CT | 2 (2–3) | 8 months | 30 |
Neck CT | 4 (3–6) | 16 months | 55 |
Chest CT | 8 (5–11) | 32 months | 117 |
Abdomen-pelvis CT with contrast | 16 (11–20) | 64 months | 234 |
Estimates for the lifetime risk of developing cancer from radiation exposure are challenging with medical imaging because the doses are so low. The lifetime risk of developing a solid cancer in the absence of exposure from medical imaging is estimated to be 45.5% in men and 36.9% in women. A 2-mSv head CT is estimated to increase this risk by 16 per 100,000 (0.016%) in men and 26 per 100,000 (0.026%) in women. Table 3 gives complete estimates for cancers and related deaths.
All Solid Cancer | Leukemia | |||
---|---|---|---|---|
Male | Female | Male | Female | |
Cases in absence of exposure | 45,500 | 36,900 | 830 | 590 |
Cases attributable to exposure | 16 | 26 | 2 | 1.4 |
Deaths in absence of exposure | 22,100 | 17,500 | 710 | 530 |
Deaths attributable to exposure | 8.2 | 12.2 | 1.4 | 1 |
When to Image
Current guidelines for the use of CT in the context of sports-related or pediatric concussion are based on expert opinion and recommend imaging when a structural, intracranial injury is clinically suspected. No prospective studies have been performed to evaluate these guidelines.
Several studies have attempted to devise and validate clinical decision rules for the use of CT in minor head injury in the general population. The two most established of these are the New Orleans Criteria and the Canadian Head CT Rule. However, the applicability of these recommendations to adolescent sports-related concussion is not clear. Ultimately, the decision to perform a CT in the context of sports-related concussion is a clinical decision based on the risks of a serious, underlying intracranial injury or skull fracture versus the risks of harm from radiation.
Magnetic Resonance Imaging
MRI uses a strong magnetic field and radiofrequency pulses of energy to excite and detect hydrogen protons. Most importantly, it does not use ionizing radiation. A typical examination involves multiple sequences that generally must be acquired sequentially. The plane of imaging is set for each sequence and usually includes some combination of axial, coronal, and sagittal planes of various sequences. Some sequences may be acquired in a volumetric manner, allowing multiplanar reformations to be performed.
The primary sequences are T1- and T2-weighted. These reflect different measures of relaxation of hydrogen protons to their low-energy state. Fluid is dark on T1-weighted images and bright on T2-weighted images. Fluid-attenuated inversion recovery (FLAIR) is an additional T2-weighted sequence in which the signal from cerebrospinal fluid is nullified, enhancing lesion conspicuity, such as edema. Diffusion-weighted and T2∗-weighted imaging is also commonly performed in the context of trauma and is further described later.
MRI is exquisitely sensitive for parenchymal lesions because these usually involve some degree of edema and are hyperintense on T2-weighted or FLAIR images ( Fig. 4 ). Acute hemorrhage (1–3 days) is hypointense on T2-weighted images because of the paramagnetic effect of the iron contained within the hemoglobin. This paramagnetic effect is especially evident on the gradient recalled echo T2∗-weighted sequence, which is similar to T2 but is more susceptible to irregularities in the magnetic field. On this sequence, blood products result in complete signal dropout and there is also “blooming,” making even punctate hemorrhages, as seen with diffuse axonal injury (DAI), stand out ( Fig. 5 ). Susceptibility-weighted imaging is a variation of this technique that has been described and is reported to have even greater sensitivity for blood products. As blood products evolve, they undergo predictable changes in their appearance on T1- and T2-weighted images, allowing estimation of their age.
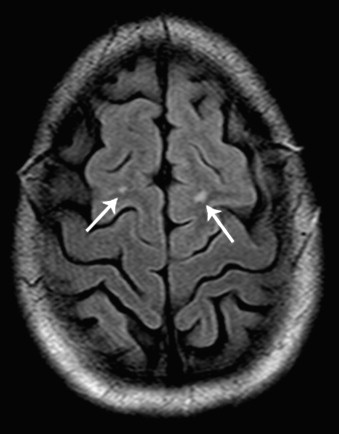
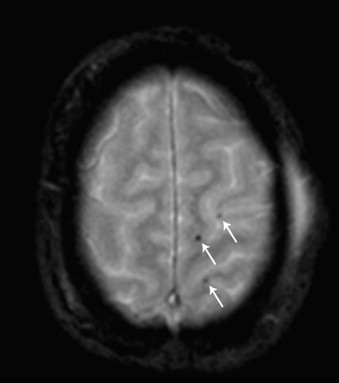
Sensitivity of CT Versus MRI
Multiple studies have demonstrated MRI to be more sensitive for the detection of intracranial injuries than CT, with the exception of subarachnoid hemorrhages and fractures. For intracranial injuries severe enough to require neurosurgical intervention, however, CT and MRI are equivalent. CT has been shown in a meta-analysis to be nearly 100% sensitive for the detection of life-threatening injuries.
The differences in sensitivity are best demonstrated in the study by Lee and colleagues using essentially modern equipment ( Table 4 ). This study prospectively compared 36 adult patients with mild TBI (GCS 13–15) using conventional CT with 5-mm axial slices and 3-T MRI using three different sequences (including three-dimensional and T2∗-weighted sequences) with 1- to 3-mm slice thickness. MRI showed greater sensitivity in detecting intraparenchymal injuries, particularly DAI and nonhemorrhagic contusions. Subdural hematomas were also detected more frequently with MRI, but this may be at least partially caused by multiple planes of imaging, which is available on modern CT scanners but not used in this study. Of note, subarachnoid hemorrhage was detected more frequently with CT. This study did not include cranial fractures, which CT is significantly better at revealing.
CT | MRI | |
---|---|---|
Abnormal | 23 | 27 |
Epidural hematoma | 4 | 3 |
Subdural hematoma | 6 | 11 |
Subarachnoid hemorrhage | 8 | 2 |
Intraventricular hemorrhage | 1 | 0 |
Intraparenchymal injury | 18 | 27 |
DAI | ||
Hemorrhagic | 8 | 17 |
Nonhemorrhagic | 0 | 4 |
Contusions | ||
Hemorrhagic | 12 | 17 |
Nonhemorrhagic | 1 | 4 |
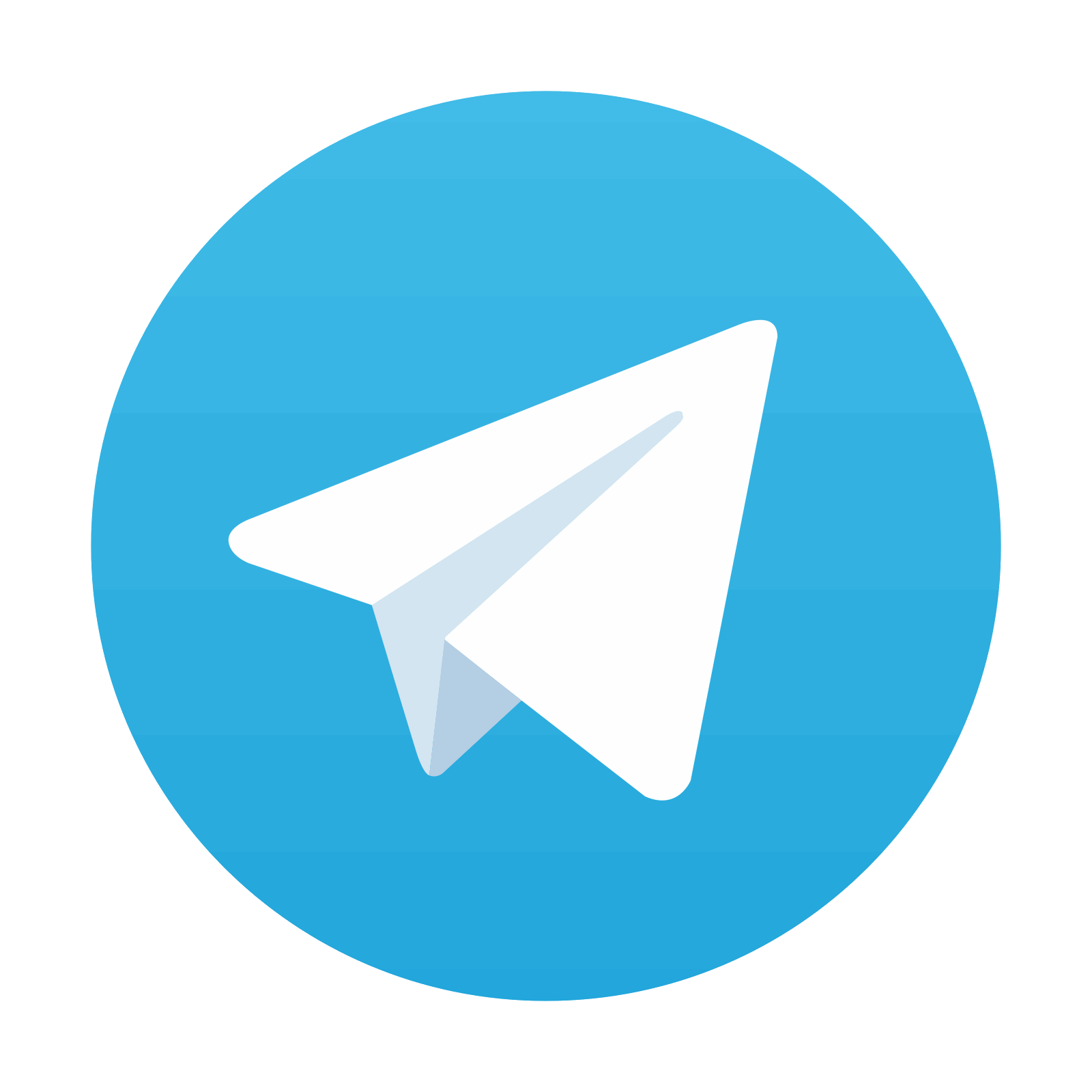
Stay updated, free articles. Join our Telegram channel

Full access? Get Clinical Tree
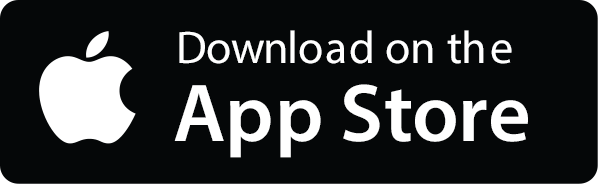
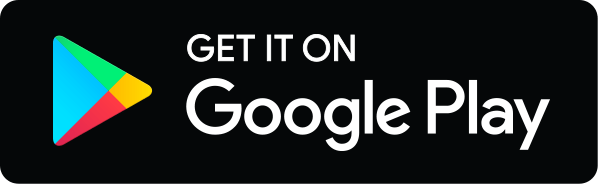
