As our human ancestors evolved to become bipedal, the scapulohumeral complex evolved to comply with the specific demands that arise from an orthograde posture and to facilitate prehension. The inherent osseous articular congruity required for weight-bearing activities was sacrificed in the upper extremities for soft tissue stability to afford a greater degree of mobility at the glenohumeral joint. Understanding this anatomic evolution is important as we consider various pathologies, such as instability, trauma, and arthrosis of the glenohumeral joint.
As in previous editions, this chapter focuses on the developmental anatomy of the shoulder girdle and the anatomy of the adult glenohumeral joint. Although much of the content of this chapter remains constant, several studies have added to our understanding of the development and anatomy of the human glenohumeral joint and have consequently been incorporated into this text.
Comparative anatomy
General development
The forelimb in humans is a paired appendage whose evolutionary roots can be traced to the longitudinal lateral folds of epidermis in the early fish species Rhipidistian crossopterygian . These folds extended caudad from the region just behind the gills to the anus ( Fig. 2.1 ). The pectoral and pelvic fins subsequently developed from the proximal and distal portions of these folds, respectively, and were the predecessors of the human upper and lower limbs ( Fig. 2.2 ).


Over time, muscle buds, along with the ventral rami of spinal nerves, migrated into these pectoral fins to allow for coordinated movement. Peripheral fibers repeatedly divided to form a plexus of nerves, and different regions of muscle tissue often combined or segmented as function evolved.
Cartilage rays called radials ( Fig. 2.3 ) developed between the muscle buds to form a support structure, and the proximal portions of these radials coalesced to form basal cartilage, or basilia . The radials began to fuse at their base and eventually formed a concrescent central axis, or pectoral girdle ( Fig. 2.4 ). These paired basilia eventually migrated ventrally toward the midline anteriorly to form a ventral bar , which corresponds to the paired clavicles in some mammals, as well as the cleithrum , a membranous bone that attached the pectoral girdle to the skull. The basilia also projected dorsally over the thorax to form the precursor of the scapula. Articulations within the basilia eventually developed at the junction of the ventral and dorsal segments (the glenoid fossa) with the remainder of the pectoral fin, which corresponds to the glenohumeral joint in humans ( Fig. 2.5 ).



As these prehistoric fish evolved into amphibians, their osseous morphology also changed to adapt to gravity out of water. The head was eventually freed from its attachments to the pectoral girdle, and in reptiles, the pectoral girdle migrated a considerable distance caudally. The pelycosaur of the late Paleozoic Era (235 to 255 million years ago) is among the oldest reptiles believed to have been solely land dwellers. These early tetrapods ambulated with the proximal part of their forelimbs held in the horizontal plane, and the distal part flexed at a 90-degree angle in the sagittal plane. Locomotion was attained by rotation of the humerus around its longitudinal axis. The cleitrum disappeared entirely during this reptilian stage.
Structural stability was primarily achieved via osseous congruity in these early reptiles, whereas the shoulder evolved to allow greater flexibility and mobility in subsequent species. The basic mammalian pattern developed with articulations arising between a well-developed clavicle and sternum medially and a flat, fairly wide scapula laterally. The coracoid enlarged during this period, and the scapular spine developed in response to new functional demands ( Fig. 2.6 ). Four main variations on this scheme are seen. Mammals adapted for running have lost their clavicle to further mobilize the scapula, and the scapula is relatively narrow. Mammals adapted for swimming also have lost the clavicle, although the scapula is wider and permits more varied function. Shoulder girdles modified for flying have a large, long, well-developed clavicle with a small, narrow, curved scapula. Shoulders modified for brachiating (including those of humans) developed a strong clavicle, a large coracoid, and a widened, strong scapula.

Furthermore, relative positioning of the forelimb became increasingly important to function as evolution moved towards an orthograde posture away from a quadruped and more primitive knuckle-walking behavior. As such, glenohumeral mobility took precedence over the stability required by quadrupeds in the setting of an upright posture. In addition to the development of a strong clavicle and widening of the scapula, the scapula is also noted to migrate and take on a more mediolaterally wide and anteroposteriorly shallow morphology.
Other adaptations for the erect posture were a relative flattening of the thorax in the anteroposterior dimension, with the scapula left approximately 45 degrees to the midline ( Fig. 2.7 ), and evolution of the pentadactyl limb with a strong, mobile thumb and four ulnar digits. This pentadactyl limb is very similar to the human arm as we know it.

In the following sections, we individually discuss the evolution of the different regions of the shoulder and pectoral girdle as they approach a more human form.
Development of individual regions
Scapula
The scapula in humans is suspended by muscles alone and clearly reflects the adaptive development of the shoulder. It has shifted caudally from the cervical position in lower animals; as a result, the shoulder is freed from the head and neck and can serve as a base or platform to facilitate arm movement. The most striking modification in the development of the bone of the scapula itself is in the relationship between the length (measured along the base of the spine) and the breadth (measured from the superior to the inferior angle) of the scapula, or the scapular index ( Fig. 2.8 ). This index is extremely high in pronograde animals with a long, narrow scapula. The scapula is broader in humans and other primates, with the most pronounced differences observed in the infraspinatus fossa, a modification referred to as an increase in the infraspinatus index.

Broadening of the infraspinatus fossa has resulted in a change in the vector of muscle pull from the axillary border of the scapula to the glenoid fossa and has consequently altered the action of the attached musculature. This adaptation allows the infraspinatus and teres minor muscles to be more effective in their roles as compressors and external rotators of the humeral head. Over time, the supraspinatus fossa and muscle have changed little in size or shape, but the acromion, which is an extension of the spine of the scapula, has enlarged (see Fig. 2.6 ). In pronograde animals, the acromion process is insignificant; however, in humans, it is a massive structure overlying the humeral head. This difference reflects the increasing role of the deltoid muscle in shoulder function. The broader attachment of this muscle on the acromion and its more distal insertion on the humerus have increased its mechanical advantage in shoulder motion.
The coracoid process has also undergone an increase in size over time (see Fig. 2.6 ). We have performed biomechanical studies showing that, with the shoulder in 90 degrees of abduction, the coracoid extension over the glenohumeral joint can mechanically limit anterior translation of the humerus relative to the glenoid. One shoulder tested after sectioning the capsule would not dislocate anteriorly in full abduction until the coracoid process was removed ( Fig. 2.9 ).

Humerus
Like the scapula, the humerus has undergone several morphologic changes during its evolution. Over time, the head of the humerus has moved proximally, underneath the torso, as well as from the horizontal plane to a more vertical resting orientation. The insertion site of the deltoid muscle has migrated distally, improving its leverage ( Fig. 2.10 ). ,

In addition, the distal humeral shaft underwent an episode of torsion relative to the proximal end of the humerus, thereby rotating the humeral head internally relative to the epicondyles. As the thoracic cage flattened in the anteroposterior plane, the scapula and glenoid fossa assumed a more dorsal position within it, which led to the glenoid fossa being directed more laterally (see Fig. 2.7 ). As a consequence, there was external rotation of the humeral head and internal rotation of the shaft relative to it, which led to medial displacement of the intertubercular groove and a decreased size of the lesser tuberosity relative to the greater tuberosity. The resultant retroversion of the humeral head has been reported to be 33 degrees in the dominant shoulder and 29 degrees in the nondominant shoulder relative to the epicondyles of the elbow in the coronal plane.
The other effect of this torsion on the humerus is that the biceps muscle, which was previously a strong elevator of the arm, has been rendered biomechanically ineffective unless the arm is externally rotated. In this fashion it can be used as an abductor, which is often seen in infantile paralysis.
Clavicle
The clavicle is not present in animals, such as horses, that use their forelimbs for standing. However, in animals that use their upper limbs for holding, grasping, and climbing, the clavicle allows the scapula and humerus to be positioned away from the body to help the limb move free of the axial skeleton. In humans, it also provides a means of transmitting the supporting force of the trapezius to the scapula through the coracoclavicular ligaments, a bony framework for muscle attachments, and a mechanism for increasing the range of motion at the glenohumeral joint.
Scapulohumeral muscles
The scapulohumeral muscles include the supraspinatus, infraspinatus, teres minor, subscapularis, deltoid, and teres major. The supraspinatus has remained relatively static morphologically but has progressively decreased in relative mass ( Fig. 2.11 ). In contrast, the deltoid has more than doubled in its proportional representation and constitutes approximately 41% of the scapulohumeral muscle mass. This increase in size also increases the overall strength of the deltoid. In lower animals, a portion of the deltoid attaches to the inferior angle of the scapula, whereas in humans, these fibers correspond to the teres minor muscle; this explains the identical innervation by the axillary nerve in these two muscles.

The infraspinatus is absent in lower species; however, in humans, it makes up approximately 5% of the mass of the scapulohumeral muscles. The subscapularis has undergone no significant change, except for a slight increase in the number of fasciculi concomitant with elongation of the scapula, and it makes up approximately 20% of the mass of the scapulohumeral group. This adaptation allows the lower part of the muscle to pull in a downward direction and assists the infraspinatus and teres minor to act as a group to function as compressors as well as stabilizers of the head of the humerus against the glenoid during arm elevation.
Axioscapular muscles
The axioscapular muscles include the serratus anterior, rhomboids, levator scapulae, and trapezius. All these muscles (except the trapezius) originated from one complex of muscle fibers arising from the first eight ribs and the transverse processes of the cervical vertebrae, inserting into the vertebral border of the scapula. As differentiation occurred, the fibers concerned with dorsal scapular motion became the rhomboid muscles, the fibers controlling ventral motion developed into the serratus anterior muscle, and finally, the levator scapulae differentiated to control cranial displacement of the scapula. The trapezius has undergone little morphologic change throughout primate development.
The axioscapular group of muscles acts to anchor the scapula to the thoracic cage while allowing freedom of motion. Most authorities report the ratio between glenohumeral and scapulothoracic motion to be 2:1. , The serratus anterior provides horizontal stability and prevents winging of the scapula.
Axiohumeral muscles
The axiohumeral muscles connect the humerus to the trunk and consist of the pectoralis major, pectoralis minor, and latissimus dorsi. The pectoral muscles originate from a single muscle mass that divides into a superficial layer and a deep layer. The superficial layer becomes the pectoralis major, and the deep layer gives rise to the pectoralis minor. The pectoralis minor is attached to the humerus in lower species, whereas in humans it is attached to the coracoid process.
Muscles of the upper part of the arm
The biceps in more primitive animals has a single origin on the supraglenoid tubercle and often assists the supraspinatus in limb elevation. In humans the biceps has two origins and, because of the torsional changes in the humerus, is ineffective in shoulder elevation unless the arm is fully externally rotated.
The triceps has not undergone significant morphologic change, but the size of the long head of the triceps has been progressively decreasing.
Embryology
Prenatal development
Three germ layers give rise to all the tissues and organs of the body. The cells of each germ layer divide, migrate, aggregate, and differentiate in rather precise patterns as they form the various organ systems. The three germ layers are the ectoderm, mesoderm, and endoderm. The ectoderm gives rise to the central nervous system, peripheral nervous system, epidermis and its appendages, mammary glands, pituitary gland, and subcutaneous glands. The mesoderm gives rise to cartilage, bone, connective tissue, striated and smooth muscle, blood cells, kidneys, gonads, spleen, and the serous membrane lining of the body cavities. The endoderm gives rise to the epithelial lining of the gastrointestinal, respiratory, and urinary tracts; the lining of the auditory canal; and the parenchyma of the tonsils, thyroid gland, parathyroid glands, thymus, liver, and pancreas. The development of the embryo requires a coordinated interaction of these germ layers, orchestrated by genetic and environmental factors under the influence of basic induction and regulatory mechanisms.
Prenatal human embryologic development can be divided into three major periods: the first 2 weeks, the embryonic period, and the fetal period. The first 2 weeks of development are characterized by fertilization, blastocyst formation, implantation, and further development of the embryoblast and trophoblast. The embryonic period comprises weeks 3 through 8 of development, and the fetal period encompasses the remainder of the prenatal period until term.
The embryonic period is important because all the major external and internal organs develop during this time, and by the end of this period, differentiation is practically complete. All the bones and joints have the form and arrangement characteristic of adults. Exposure to teratogens during this period can cause major congenital malformations. During the fetal period, the limbs grow and mature as a result of a continual remodeling and reconstructive process that enables bones to maintain their characteristic shape. In the skeleton in general, increments of growth in individual bones are in precise relationship to those of the skeleton as a whole. Ligaments show an increase in collagen content, bursae develop, tendinous attachments shift to accommodate growth, and epiphyseal cartilage becomes vascularized.
Few studies have focused on the prenatal development of the glenohumeral joint. The contributions by DePalma and Gardner were essential but did not emphasize clinical correlations between the observed fetal anatomy and the pathology seen in the postnatal shoulder. Most studies on the developing shoulder have focused primarily on bone maturation, and analysis of the soft tissue structures of the developing shoulder, such as the joint capsule and the labrum, is still incomplete.
Most recently a 2018 study from Hita-Contreras et al. reported on human embryos and fetuses with focus on glenohumeral joint development (PMID 29193070). The investigators further contributed to the literature regarding soft tissue development of the joint including the labrum, capsule, biceps, and glenohumeral ligaments. Despite the continued reports of human glenohumeral development, there is still a need for further studies on more specific portions of the glenohumeral soft tissue anatomy including the inferior glenohumeral ligament complex (IGHLC), which has been shown to be an integral component for stability in the adult shoulder. The seminal studies of the fetal glenohumeral joint were completed before the role of the soft tissue structures in shoulder stability was elucidated. There is now a greater appreciation of the anatomy and biomechanics of the static and dynamic stabilizers of the glenohumeral joint and their role in shoulder stability.
Embryonic period
The limb buds are initially seen as small elevations on the ventrolateral body wall at the end of the fourth week of gestation. The upper limb buds appear during the first few days of the fourth week and maintain a growth advantage over the lower limbs throughout development. Because development of the head and neck occurs in advance of the rest of the embryo, the upper limb buds appear disproportionately low on the embryo’s trunk ( Fig. 2.12 ). During the early stages of limb development, the upper and lower extremities develop in a similar fashion, with the upper limb buds developing opposite the lower six cervical and the first and second thoracic segments.

At 4 weeks, the upper limb is a sac of ectoderm filled with mesoderm and is approximately 3 mm long. Each limb bud is delineated dorsally by a sulcus and ventrally by a pit. The pit for the upper limb bud is called the fossa axillaris . The mesoderm in the upper limb bud develops from somatic mesoderm and consists of a mass of mesenchyme, which is loosely organized embryonic connective tissue. Mesenchymal cells can differentiate into different types of cells, including fibroblasts, chondroblasts, and osteoblasts ( Fig. 2.13 ). Most bones first appear as a condensation of these mesenchymal cells, from which a core called the blastema is formed. , This development is orchestrated by the apical ectodermal ridge ( Fig. 2.14 ), which exerts an inductive influence on the limb mesenchyme, promoting growth and development.


During the fifth week, a number of developments occur simultaneously. The peripheral nerves grow from the brachial plexus into the mesenchyme of the limb buds. Such growth stimulates development of the limb musculature, where in situ somatic limb mesoderm aggregates and differentiates into myoblasts and discrete muscle units. This process differs from the development of the axial musculature, which arises from the myotomic regions of segments of two longitudinal columns of paraxial mesoderm known as somites ( Fig. 2.15 ). Also at this time, the central core of the humerus begins to chondrify, although the shoulder joint has not yet formed. There is an area in the blastema called the interzone that does not undergo chondrification and is the precursor of the shoulder joint ( Fig. 2.16 ). The scapula at this time lies at the level of C4 and C5 ( Fig. 2.17 ), and the clavicle begins to ossify. (Along with the mandible, the clavicle is the first bone to begin to ossify.)



During the sixth week, the mesenchymal tissue in the periphery of the hand plates condenses to form digital rays. The mesodermal cells of the limb bud rearrange to form a deep layer, an intermediate layer, and a superficial layer. This layering is brought on by differential growth rates. Such differential growth in the limb also stimulates bending at the elbow because the cells on the ventral side grow faster than those on the dorsal side, which stretches to accommodate the ventral growth. The muscle groups divide into dorsal extensors and ventral flexors, and the individual muscles migrate caudally as the limb bud develops. In the shoulder joint the interzone assumes a three-layered configuration, with a chondrogenic layer on either side of a loose layer of cells. At this time, the glenoid labrum is discernible ( Fig. 2.18 ), although cavitation or joint formation has not yet occurred. Initial bone formation begins in the primary ossification center of the humerus. The scapula at this time undergoes marked enlargement and extends from C4 to approximately T7.

Early in the seventh week, the limbs extend ventrally and the upper and lower limb buds rotate in opposite directions ( Fig. 2.19 ). The upper limbs rotate laterally through 90 degrees around their longitudinal axes, with the elbow facing posteriorly and the extensor muscles facing laterally and posteriorly. The lower limbs rotate medially through almost 90 degrees, with the knee and extensor musculature facing anteriorly. The final result is that the radius is in a lateral position in the upper limb, and the tibia is in a medial position in the lower limb, although these are homologous bones. The ulna and fibula are also homologous bones, and the thumb and great toe are homologous digits. The shoulder joint is now well-formed, and the middle zone of the three-layered interzone becomes progressively less dense with increasing cavitation ( Fig. 2.20 ). The scapula has now descended and spans from just below the level of the first rib to the level of the fifth rib. The brachial plexus has also migrated caudally and lies over the first rib. The final few degrees of downward displacement of the scapula occur later when the anterior portion of the rib cage drops obliquely downward.


By the eighth week the embryo is approximately 25 to 31 mm long, and through the growth of the upper limb, the hands are stretched with the arms pronated ( Fig. 2.21 ). The musculature of the limb is now also clearly defined. The shoulder joint has the form of the adult glenohumeral joint, and the glenohumeral ligaments can now be visualized as thickenings in the shoulder capsule. ,

Although certain toxins and other environmental factors can still cause limb deformities (e.g., by affecting the vascular supply), it is the embryonic period that is most vulnerable to congenital malformations, with the type of abnormality depending on the time at which the orderly sequence of differentiation was interrupted. In gross limb abnormalities, such as amelia, injury to the apical ectodermal ridge is one important factor, which has a strong inductive influence on the limb mesoderm. Matsuoka and colleagues mapped the destinations of embryonic neural crest and mesodermal stem cells in the neck and shoulder region using Cre recombinase–mediated transgenesis and proposed a precise code of connectivity that mesenchymal stem cells of both neural crest and mesodermal origin obey as they form muscle scaffolds. The conclusions suggested that knowledge of these relationships could contribute further to identify the etiology of diseases, such as Klippel-Feil syndrome, Sprengel deformity, and Arnold-Chiari I/II malformation. Clearly, the timing of embryonic development is critical for understanding anomalies and malformations and is an area of further study.
Fetal period
Fetal development is concerned mainly with expansion in size of the structures differentiated and developed during the embryonic period. Ossification proceeds rapidly during this period, especially during the 13th to 16th weeks. The first indication of ossification in the cartilaginous model of a long bone is visible near the center of the shaft. Primary centers appear at different times in different bones but usually between the 7th and 12th weeks. The part of the bone ossified from the primary center is called the diaphysis . Secondary centers of ossification form the epiphysis . The physeal plate separates these two centers of ossification until the bone grows to its adult length, and from the 12th to the 16th week, the epiphyses are invaded by a vascular network. In the shoulder joint the epiphysis and part of the metaphysis are intracapsular. The tendons, ligaments, and joint capsule around the shoulder are also penetrated by a rich vascular network during the same part of the fetal period (i.e., the third to fourth month of gestation).
A morphologic study of the prenatal developing shoulder joint concluded that the most important changes take place around the 12th week of gestation. At about this time, the glenoid labrum, the biceps tendon, and the glenohumeral ligaments formed a complete ring around the glenoid fossa and led the authors to believe that these structures play a role in stabilizing the joint and increasing the concavity of the glenoid fossa. The glenoid labrum consists of dense fibrous tissue and some elastic tissue but no fibrocartilage (as is seen in the meniscus of the knee). The acromioclavicular joint develops in a manner different from that of the shoulder joint. Its development begins well into the fetal period (not the embryonic period), and unlike the glenohumeral joint, a three-layered interzone is not seen ( Fig. 2.22 ). Most of the bursae of the shoulder, including the subdeltoid, subcoracoid, and subscapularis bursae, also develop during this time.

Fealy and colleagues studied 51 fetal glenohumeral joints from 37 specimens to evaluate shoulder morphology on a gross and histologic level and compare it with known postnatal anatomic and clinical findings in fetuses from 9 to 40 weeks of gestation. Specimens were studied under a dissecting microscope, histologically, and with the aid of high-resolution radiographs to evaluate the presence of ossification centers. The fetal gross anatomy and morphology were similar to those of normal postnatal shoulders in all specimens. As noted previously, only the clavicle and spine of the scapula were ossified in the fetal shoulder. The humeral head and glenoid gradually and proportionally increased in size with gestational age. Comparative size ratios were consistent, except for the fetal coracoid process, which was noted to be prominent in all specimens ( Fig. 2.23 ).

In a study by Tena-Arregui and colleagues, frozen human fetuses (40 shoulders) were grossly evaluated arthroscopically, with similar findings. They concluded that the anatomy observed was easier to discern than what is observed in the adult shoulder arthroscopy ( Fig. 2.24 ).

Coracoacromial arch anatomy
By 13 weeks of gestation, the rotator cuff tendons, coracoacromial ligament (CAL), and coracohumeral ligament are present. The acromion is cartilaginous and consistently has a gentle curve that conforms to the superior aspect of the humeral head, similar to a type II acromion ( Fig. 2.25 ). These data suggest that variations in acromial morphology are acquired. As Codman noted, the congruity of the coracoacromial arch likely plays some role in stability—as evidenced by the conversion of a rotator cuff–deficient patient from a coper to pseudoparalysis following resection of the CAL.

A macroscopic and histologic study conducted by Shah and colleagues analyzed 22 cadaveric shoulders to establish what, if any, developmental changes occur in the differing patterns of acromia. In all the curved and hooked acromia (types II and III), a common pattern of degeneration of collagen, fibrocartilage, and bone was observed, consistent with a traction phenomenon. None of these changes was exhibited by the flat acromion (type I). They therefore supported the conclusion that the different shapes of acromion are acquired in response to traction forces applied via CAL and are not congenital.
In the fetus, CAL consists of two distinct fiber bundles that lie in the anterolateral and posteromedial planes, as it does in the mature shoulder. Histologic studies have shown that CAL continues posteriorly along the inferior surface of the anterolateral aspect of the acromion. CAL has well-organized collagen fiber bundles by 36 weeks of gestation.
In a study by Kopuz and colleagues, 110 shoulders from 60 neonatal cadavers were dissected and analyzed for CAL variations. Three CAL types were identified: quadrangular, broad band, and U-shaped. Histologic analysis showed that the U-shaped ligaments had a thin central tissue close to the coracoid. The data suggested that the primordial CAL is broad shaped but assumes a quadrangular shape because of the different growth rates of the coracoid and acromial ends. In addition, broad and U-shaped CALs account for the primordial and quadrangular types, respectively, and Y-shaped ligaments account for the adult types of the single- or double-banded anatomic variants. They concluded that various types of CALs are present during the neonatal period and that the final morphology is determined by developmental factors rather than degenerative changes.
Glenohumeral capsule and glenohumeral ligaments
The anterior glenohumeral capsule has been found to be thicker than the posterior capsule. The fetal shoulder capsule inserts onto the humeral neck in the same fashion as in the mature shoulder and has been found to be confluent with the rotator cuff tendons at their humeral insertion. Superior and middle glenohumeral ligaments are identifiable as capsular thickenings, whereas the inferior glenohumeral ligament is a distinct structure identifiable by 14 weeks of gestation. Anterior and posterior bands are often noticeable in the ligament, consistent with the known IGHLC anatomy in the adult shoulder. The anterior band of the IGHLC contributes more to the formation of the axillary pouch than does the posterior band.
Histologically, the fetal IGHLC consists of several layers of collagen fibers that are highly cellular and have little fibrous tissue during early development. This tissue becomes more fibrous later in gestation. Polarized light microscopy demonstrates that these fibers are only loosely organized but are more organized than the adjacent capsular tissues. Arthroscopic images of the superior glenohumeral ligament have revealed a defined attachment to the humeral head, forming an intersection of the biceps tendon as it enters the bicipital groove and the attachment of the upper edge of the subscapular muscle tendon.
A rotator interval defect was noted in fetuses by 14 weeks of gestation. This capsular defect was seen consistently in the 1 o’clock position in a right shoulder or the 11 o’clock position in a left shoulder. The interval defect was often covered by a thin layer of capsule that extended from the middle glenohumeral ligament and passed superficially to the defect. Removal of this capsular layer revealed a clear defect between the superior and middle glenohumeral ligaments. Histologic examination of the interval defect in a specimen from the 19th week of gestation revealed a thin surrounding capsule with poorly organized collagen fibers. To our knowledge, this is the first suggestion that the capsular defect is not acquired. Specimens with larger rotator interval defects had greater amounts of inferior glenohumeral laxity. Closure of a large rotator interval defect in adults has been shown to be an effective treatment for inferior glenohumeral instability.
Biceps tendon
Although rare, the proximal portion of the long head of the biceps tendon (LHBT) has been reported to develop anomalously. As such, the developmental anatomy of the biceps has been brought to focus in recent studies. Specifically, Audenaert et al. suggested that the proximal LHBT migrates from the glenohumeral capsule and synovial layer into the intra-articular space prior to weeks 20 or 24 of fetal development. Although not widely adopted, this pattern has been observed and reported by others. Several case reports of anomalous biceps anatomy seen intraoperatively among adults led to the hypothesis that aberrant development can occur at any point throughout this migration. Developmental fusions of the biceps tendon to the supraspinatus, fibrous capsule, and synovial lining have all been observed and would suggest confirmation of this migratory process at varying endpoints. Audenaert et al. proposed a classification of these stages in the embryo, in which the proximal portion of the LHBT originates in the intracapsular position, moves through the synovial layer, and comes to rest in the intra-articular position ( Fig. 2.26 ). Anatomic variation in the long head of the biceps exists as a double structure or insertion within the fibrous capsule. In a recent case, Provencher et al. reported bilateral absence of the intra-articular long head of the biceps in a patient with no history of congenital development abnormalities. Another recent case was reported of a trifurcate biceps tendon. Electromyographic analysis of the shoulder motion demonstrates that, despite its presence within the joint, the long head of the biceps is not involved in glenohumeral motion.

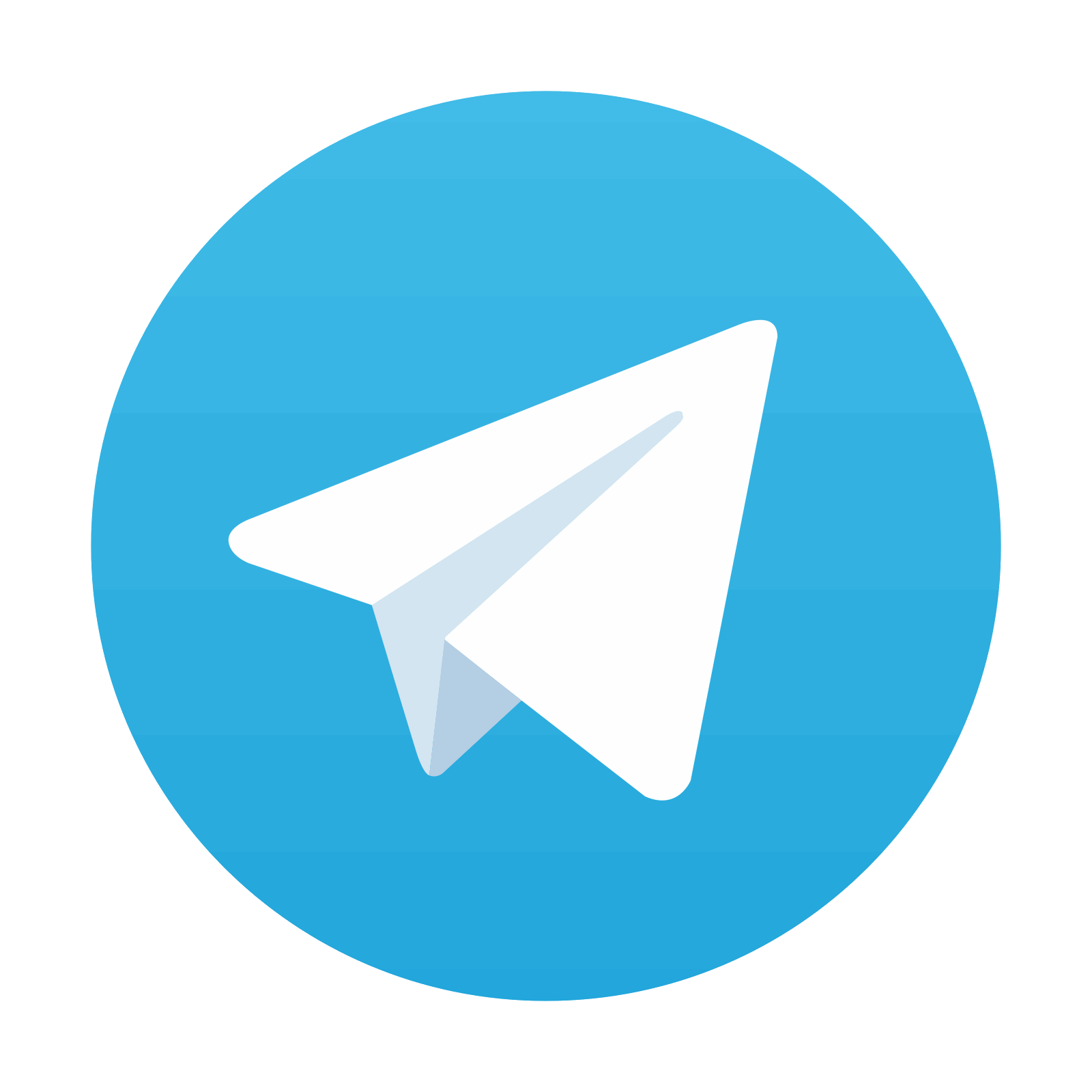
Stay updated, free articles. Join our Telegram channel

Full access? Get Clinical Tree
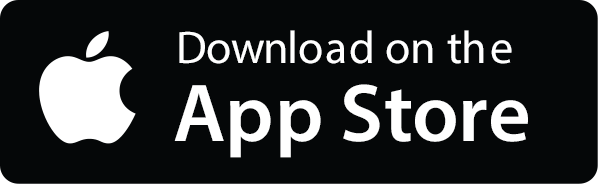
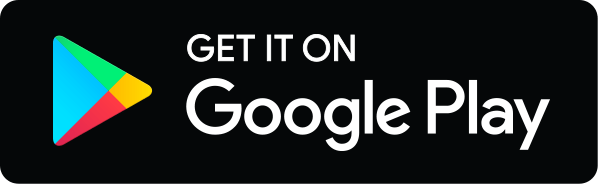
