CHAPTER OUTLINE
Basic Science 457
Chemical Structure and Molecular Weight 457
Crystallinity 457
Cross-Linking 458
Thermal Processing: Annealing and Remelting 460
Clinical Studies Comparing Cross-Linked and Conventional Polyethylene 460
Current Controversies 463
Summary and Conclusions 464
Does Cross-Linked Polyethylene Reduce In Vivo Head Penetration Rates? 464
Do Certain Cross-Linked Polyethylene Formulations Reduce In Vivo Head Penetration Rates More Than Others? 465
Does Cross-Linked Polyethylene Reduce In Vivo Wear Rates? 465
Highly cross-linked and thermally treated ultra–high-molecular-weight polyethylene (UHMWPE) (hereafter, cross-linked polyethylene) has been used in total hip arthroplasty in the United States since the late 1990s. Since that time, cross-linked polyethylene has steadily replaced conventional polyethylene for hip arthroplasty. According to recent surveys, approximately 70% of hip replacements performed in the United States employ a cross-linked polyethylene liner. For 2006, approximately 250,000 primary and revision hip total procedures were projected to be performed annually in the United States. Therefore an estimated 175,000 total hip arthroplasty patients in the United States are now considered to benefit from cross-linked polyethylene technology every year. Considering the historical growth in the use of cross-linked polyethylene since its clinical introduction in 1998, combined with the projected growth in hip arthroplasty, over one million patients will have received a cross-linked liner in the United States by 2007.
Cross-linked polyethylene has come to be widely accepted by surgeons as an alternative to hard-on-hard bearings for improving the wear resistance of hip arthroplasty. As we shall see subsequently in this chapter, the scientific foundation for such enthusiasm for cross-linked polyethylene warrants critical appraisal. In the 1990s, polyethylene wear, particle-mediated osteolysis, and aseptic loosening were regarded as among the most important clinical problems limiting the longevity of hip replacements. Dumbleton and colleagues demonstrated from review of the literature that osteolysis was likely in patients with head penetration into the bearing of more than 0.1 mm/year and unlikely in patients with head penetration of less than 0.05 mm/year. Therefore alternative bearings were urgently needed to reduce wear and secondary osteolysis, thereby improving long-term survivorship of the replacement.
During the mid-1990s, retrieval analyses also identified oxidation in the polymer, attributed to the gamma sterilization process together with long-term shelf storage or oxidation in vivo. The association between oxidation and clinical performance of hip arthroplasty was not completely clear. However, it was considered desirable in certain scientific circles to engineer polyethylene materials for not only wear resistance, but oxidation resistance as well. It is important to keep in mind that what we refer to as “cross-linked polyethylenes” throughout this chapter are actually fabricated in two steps: the first cross-linking step is designed to improve wear resistance, whereas the second thermal processing step is designed to achieve a combination of oxidation resistance and mechanical properties.
Because both cross-linking and thermal processing can deleteriously influence mechanical properties, such as fracture resistance, creep, and ultimate strength, the precise details employed in these manufacturing steps have been the subject of considerable scientific debate and controversy over the past 8 years. The polymer science and technology used to produce cross-linked polyethylene have been summarized in a monograph. Therefore in the current chapter we will provide the practicing surgeon or resident with an overview of the current concepts used to design cross-linked polyethylenes for hip arthroplasty. Readers interested in a more detailed treatment of cross-linked polyethylene technology are referred to the UHMWPE Handbook or a frequently updated online resource dedicated to this subject ( www.uhmwpe.org ).
Introduced in 1998, cross-linked polyethylene became the standard for hip arthroplasty in the United States somewhat fortuitously, at a time when many different bearing technologies, including metal on metal and ceramic on ceramic, were under investigation and commercialization. From a regulatory perspective, the approval of cross-linked polyethylenes was accomplished in straightforward fashion using the relatively streamlined 510(k) process, whereas other hip bearing technologies such as ceramic on ceramic required an investigational device exemption (IDE), involving at least a 2-year prospective randomized clinical trial. Although, as described later, prospective clinical trials were conducted for cross-linked polyethylene, they were not a prerequisite to its clinical introduction.
Because of its improved wear-resistance, cross-linked polyethylene is now regarded as a desirable technology for hip articulations. The same cannot be said unequivocally for the use of highly cross-linked polyethylene in total knee replacement, where concerns about reduced material properties have continued to limit its clinical acceptance. In this chapter, we focus specifically on applications of cross-linked polyethylene in the hip. We begin with an overview of the basic science concepts and terminology surrounding cross-linked polyethylene and the two main thermal processing techniques, which involve either annealing or remelting the polymer after cross-linking. The second part of the chapter is a critical assessment of the peer-reviewed literature on the subject of femoral head penetration and wear in cross-linked polyethylenes measured in clinical studies.
BASIC SCIENCE
This section covers the basic science concepts underlying the design of contemporary cross-linked polyethylenes. The section begins with a discussion of molecular weight, crystallinity, cross-linking, and in vivo oxidation. In this section, we also discuss annealing and remelting postirradiation thermal treatments and the pros and cons of both approaches. Readers familiar with the basic science aspects of polyethylene may wish to advance to the next section, which summarizes the clinical performance of cross-linked materials.
Chemical Structure and Molecular Weight
Polyethylene is a polymer of ethylene and consists of a carbon backbone chain with pendant hydrogen atoms. It is the simplest of polymer molecules chemically, but as the length of the polymer chain increases, so too does the complexity of the material. When the molecular weight of the polymer reaches about 40,000 daltons (low-density polyethylene), the material has a soft and ductile characteristic and is used for products such as garbage bags, for example. UHMWPE, used in orthopedic hip and knee applications since 1962, has a molecular weight ranging from 2 to 6 million daltons. By virtue of its molecular weight, UHMWPE has the desirable attributes of wear and impact resistance, together with ductility and toughness. These attributes make UHMWPE highly suitable as a bearing material.
There has been some confusion in the historical arthroplasty literature, much of it written by Charnley, in which what is now considered UHMWPE was historically referred to as high-density polyethylene (HDPE) or polythene. Today, high-density polyethylene refers to a material with a molecular weight of 100 to 250,000 daltons and is suitable for milk jugs, not artificial joints. In a hip simulator, HDPE has a wear rate that is four times higher than that of UHMWPE. It is clear, therefore, that what we consider today to be modern HDPE has never been used clinically.
We will not dwell more on the nomenclature or history of UHMWPE throughout its four decades of uninterrupted clinical use, as these topics are treated in a previous monograph and are also discussed online. For the purposes of this chapter, we are concerned primarily with modern UHMWPE, which for convenience we will continue to refer to simply as polyethylene.
Crystallinity
Crystallinity is an important attribute of all polyethylenes, including cross-linked polyethylene. The molecular chains in polyethylene have a natural tendency (driven by thermodynamics) to preferentially fold up against themselves whenever possible, hindered by the considerable crowding and thermal jostling presented by adjacent molecules. Regions of the polymer with folded chains are referred to as crystallites. Individual crystallites in polyethylene are microscopic and can typically be visualized only after staining using an electron microscope, as shown in Figure 61-1 . To the naked eye, crystalline regions diffract visible light, giving polyethylene its white appearance. In the transmission electron microscope (see Fig. 61-1A ), the crystalline regions appear like thick white lines, whereas the regions with randomly oriented polymer chains, referred to as the amorphous regions, appear dark gray.

In polyethylene, the crystallites have a particular “lamella” shape. If we were to dissolve away the amorphous regions, the crystalline lamellae in polyethylene would look something like twisted, interconnected sheets, as shown conceptually in Figure 61-1B . A close-up illustration of a crystal lamella is shown in Figure 61-1C . The molecular chains are oriented perpendicular to the plane of the lamella and may emerge to connect with adjacent lamellae. These connective polymer chains (not shown) are referred to as tie molecules. In particular, it is thought that tie molecules, or entanglements, contribute greatly to the inherent wear resistance of polyethylene.
Crystallinity is an important structural attribute of polyethylene because the size, alignment, and connectivity (via tie molecules of lamellae) play a major role in the mechanical behavior of the material. Crystals are stiffer than the amorphous regions; therefore the elastic modulus and yield stress of polyethylene will increase in direct relation to the number of crystals present. As we shall see, many of the processing steps for clinical polyethylenes are tailored specifically to optimize the crystalline structure and thereby tune its material properties.
Polyethylene typically has a crystalline content of about 50%. By raising the temperature of the bulk material above its melting temperature, around 137° C, the molecular chains have too much thermal energy to remain folded, and therefore the lamellae dissolve and “melt.” In the molten state the molecular weight of polyethylene is sufficiently high that it remains a viscous, albeit translucent, solid. The size and number of crystals in polyethylene at room temperature, and hence bulk material properties, depend on both the rate of cooling and the amount of pressure applied during the cooling process. On a commercial scale, therefore, the crystalline content and mechanical properties of polyethylene rods and sheets may be tailored by thermal processing. Different polyethylene manufacturers use proprietary combinations of pressure, temperature, and time to optimize their products.
In summary, thermal processing alters the basic organization of molecular chains in polyethylene by modifying the size and shape of the crystals. However, heating and cooling, even under pressure, are not intended to alter the polymer’s chemical structure, because covalent bonds in the material are neither broken nor created. In contrast, as discussed in the following two sections, the chemistry of polyethylene can be irrevocably modified by cross-linking (beneficial) as well as by degradation (detrimental).
Cross-Linking
Cross-linking, the foundation of all modern polyethylene total hip bearings, is a complicated subject and the foundation for many proprietary materials. Simply put, the chemical structure of polyethylene is fundamentally altered by cross-linking, which itself is defined as the joining of two independent polymer molecules by a chemical covalent bond. Cross-linking of polyethylene can be achieved by peroxide chemistry, silane chemistry, or high-energy radiation. Of these, only radiation cross-linking has been commercialized by orthopedic device manufacturers.
The mechanism of radiation cross-linking is schematically illustrated in Figure 61-2 . The first step involves irradiation of the polyethylene molecule (see Fig. 61-2A ). Next, irradiation produces a hydrogen radical, leaving a so-called “free” radical on the polyethylene molecule (see Fig. 61-2B ). Actually, the radical on the polymer chain has extremely limited mobility and is hindered by the adjacent molecule. It may be more properly referred to as a “macroradical.” For cross-linking to occur, macroradicals must be present on adjacent polyethylene molecules, and the molecules must be mobile (see Fig. 61-2C ). When the adjacent radicals react, a covalent bond, or cross-link, is formed between the two polyethylene molecules (see Fig. 61-2D ).

The extent of cross-linking in polyethylene is proportional to the absorbed dose of radiation. Historically, polyethylene bearings were gamma sterilized at a dose of 25 to 40 kGy. This dose resulted in the formation of some cross-links. Saturation of cross-linking was achieved only at approximately 100 kGy of absorbed dose. Today, cross-linked polyethylenes are processed with a total dose ranging from 50 to 105 kGy, depending on the manufacturer. In general, increasing the dose provides a proportional improvement in wear resistance, as quantified by a hip simulator, with diminished benefits observed above 100 kGy. The precise reasons why cross-linking improves wear resistance are still not completely understood but are thought to derive mostly from the improved resistance to uniaxial orientation that accompanies cross-linking. The dominant wear mechanism for polyethylene in hips involves preferential orientation, followed by debris formation resulting from cross-shear. Because cross-linking makes it more difficult for the material to become oriented preferentially, the usual wear mechanism in hip replacements is disrupted.
One choice an implant designer has to make is the method of cross-linking (e.g., gamma versus electron beam radiation). If irradiation is to be carried out using electron beam irradiation, the designer must consider the additional factor of irradiation temperature, because the rate of energy dissipation increases the temperature above the melting temperature. Of the five major orthopedic manufacturers currently producing cross-linked polyethylene implants, one has chosen electron beam irradiation, whereas the other four use gamma radiation cross-linking.
Although resistance to multiaxial deformation is desirable for wear resistance in a hip application, cross-linking reduces the ductility and resistance of polyethylene to uniaxial tension. The resistance of polyethylene to fatigue and fracture also decreases with increased dose. Therefore the dose of a cross-linked material is chosen with not only wear resistance in mind, but also the impact the dose will have on other desirable mechanical properties, such as ductility and fracture resistance. Because cross-linking improves certain properties at the expense of others, developers of orthopedic implants must balance cross-linking with the desire to maintain mechanical properties and/or oxidation resistance.
Thermal Processing: Annealing and Remelting
In the production of a highly cross-linked UHMWPE, the material is subjected to a thermal treatment step to reduce the level of free radicals via further cross-linking reactions. At higher temperatures the polymer molecules have increased mobility, thereby increasing the probability of free radicals on adjacent chains to react and form cross-links. For the thermal treatment to be effective at eliminating all free radicals, it must be conducted above the melting temperature of the material at 150° C. Heating above the melting temperature destroys the crystalline regions of the material, thus making the free radicals that were in the crystals available for cross-linking. The disadvantage of melting is the reduction in polymer crystal size and in mechanical properties (e.g., material yield and ultimate strength) that ensues when the material returns to room temperature.
A compromise solution is to heat the material to 130° C to 135° C, just below the melting temperature. This solution preserves the original crystal structure, retains mechanical properties, and makes more free radicals available for cross-linking than would be available without thermal treatment. Some free radicals are retained in the crystal domains, but the number is substantially reduced by the elevated temperature. When thermal treatment is conducted below the melt transition of 135° C, it is referred to as annealing, and above the melt transition, it is called remelting.
In summary, thermal processing is a final tuning step for cross-linked polyethylene, but it is not a casual afterthought. The choice of thermal treatment has a significant impact on the crystallinity and mechanical properties of cross-linked polyethylene. It can also influence the resistance of a material to in vivo oxidation. Although the virtues of annealing and remelting have been debated in the literature, ultimately the overall clinical performance of cross-linked polyethylene materials is the final arbiter of success.
CLINICAL STUDIES COMPARING CROSS-LINKED AND CONVENTIONAL POLYETHYLENE
Today, several formulations of cross-linked polyethylene are available clinically (e.g., Crossfire, Longevity, Marathon, XLPE, Durasul, X3, ArCom XL, AcuMatch XL, and ArCom E). Of these, published clinical data concerning only four formulations are available (Crossfire, Stryker Orthopedics, Mahwah, NJ; Durasul, Zimmer, Warsaw, IN; Marathon, DePuy Orthopedics, Warsaw, IN; and Longevity, Zimmer, Warsaw, IN), and these are summarized in Table 61-1 . As our objective in this section is to summarize the current clinical evidence reported for cross-linked as compared with conventional polyethylene, we have excluded those formulations with no track record in the peer-reviewed scientific literature from our discussion. Published clinical studies are given (in alphabetic order) for Crossfire, Durasul, Marathon, and Longevity.
Crossfire | Durasul | Marathon | Longevity | |
---|---|---|---|---|
Manufacturer | Stryker Orthopedics | Zimmer | DePuy Orthopedics | Zimmer |
Clinical introduction | 1998 | 1998 | 1998 | 1999 |
Total dose (kGy) | 105 | 95 | 50 | 100 |
Thermal treatment | Annealed | Remelted | Remelted | Remelted |
Sterilization method | Gamma in nitrogen | Ethylene oxide | Gas plasma | Gas plasma |
Detectable free radicals? | Yes | No | No | No |
Longest average published clinical follow-up | 4.9 years | 5 years | 5.7 years | 3.3 years |
Crossfire
Crossfire, an annealed highly cross-linked polyethylene, was developed by Stryker Orthopedics (Mahwah, NJ). Crossfire was clinically introduced in the fall of 1998 for the Series II liner in the Omnifit acetabular cup design. After Osteonics acquired Howmedica in 1999 and formed Stryker Howmedica Osteonics, Crossfire was subsequently extended to the System 12 and Trident acetabular cup designs. Additional information about Crossfire can be found in the UHMWPE Handbook.
In the Crossfire process, extruded rod bar stock is irradiated with a nominal dose of 75 kGy and then annealed at 130° C. Acetabular components are then machined from the bar stock, barrier packaged in nitrogen, and gamma sterilized with a nominal dose of 30 kGy. Consequently, components that have been through the Crossfire process have received a total dose of 105 kGy.
Four clinical studies of Crossfire have been published to date ( Table 61-2 ), including a 2-year prospective randomized multicenter trial. These four studies report a reduction in head penetration for Crossfire ranging from 42% to 85% (see Table 61-2 ). All of these studies employed 28-mm diameter CoCr femoral heads.
Martell et al (2003) | Rohrl et al (2005) | Krushell et al (2005) | D’Antonio et al (2005) | |
---|---|---|---|---|
Study type | RCT | Pcoh | Hcoh | Hcoh |
Number of institutions | 5 | 1 | 1 | 1 |
Cup design | Secur-Fit HA | Osteonics | Microstructured PSL | Microstructured PSL |
Cup fixation | Noncemented | Cemented | Noncemented | Noncemented |
Head size | 28 mm | 28 mm | 28 mm | 28 mm |
Head material | CoCr L-Fit | CoCr | CoCr L-Fit | CoCr L-Fit |
Average age | 60 | 58 | 69 | 57.4 |
Age range | 28-76 | 49-79 | 45-83 | — |
Number of hips | 46 (24 Crossfire) | 50 (10 Crossfire) | 80 (40 Crossfire) | 109 (56 Crossfire) |
Follow-up in years | 2.3 | 3 | 4 | 4.9 |
Range in follow-up | 1.8-3.2 | 3 | 2.6-4.7 | 4-5.8 |
Number of device failures | None | None | None | None |
Wear methodology | Martell | UmRSA | Ramakrishnan | Ramakrishnan |
Two-dimensional linear penetration (mm/yr) * —cross-linked | 0.12 ± 0.05 | 0.02 | 0.05 ± 0.02 | 0.06 ± 0.02 |
Two-dimensional linear penetration (mm/yr) * —control | 0.20 ± 0.10 | 0.16 | 0.12 ± 0.06 | 0.14 ± 0.07 |
Percent reduction | 42 | 85 | 58 | 72 |
Radiographic assessment of osteolysis | No | No | No | Yes |
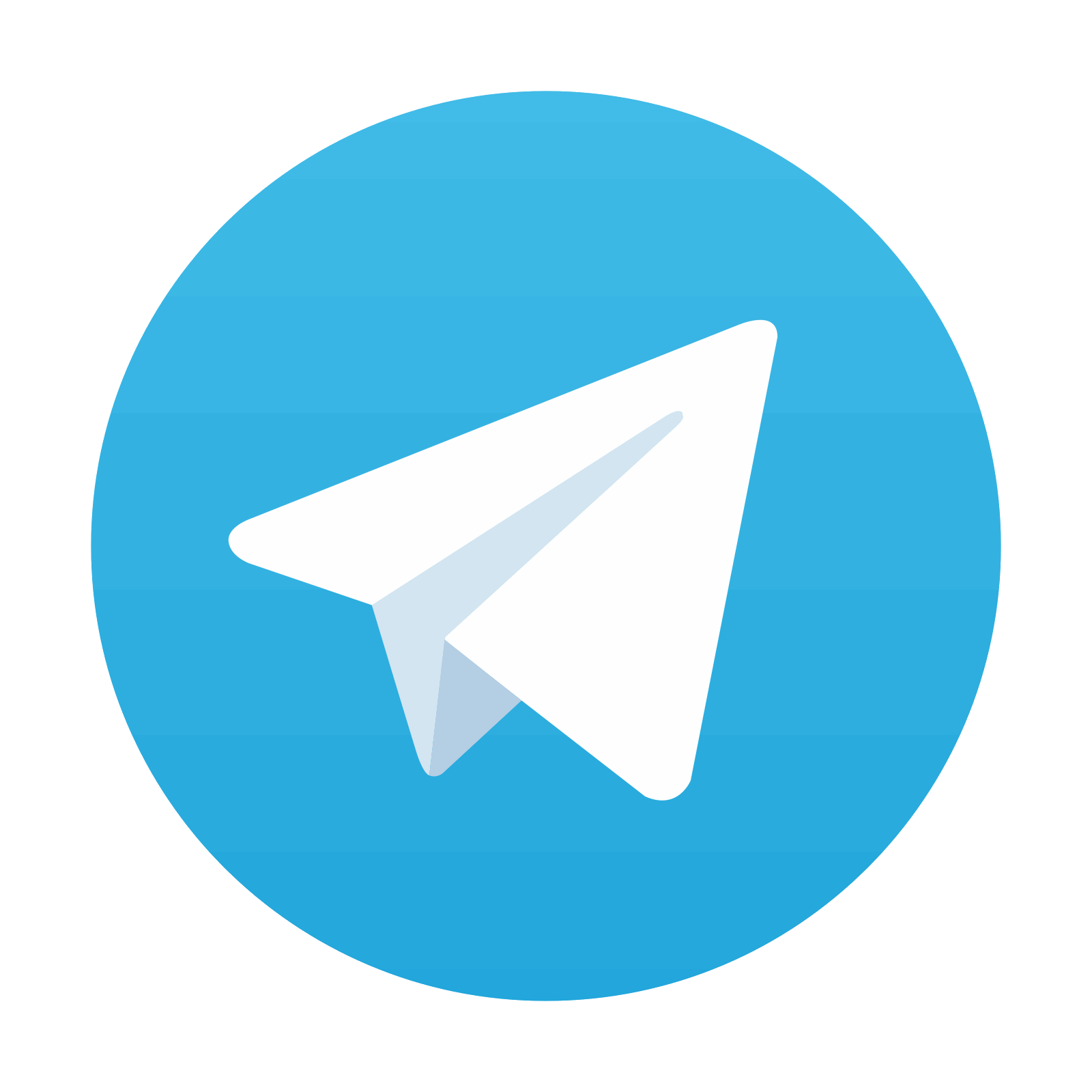
Stay updated, free articles. Join our Telegram channel

Full access? Get Clinical Tree
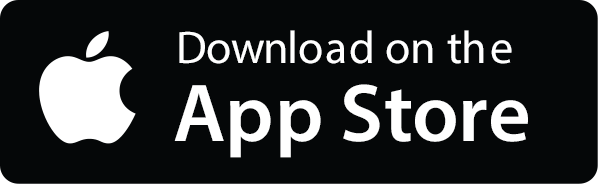
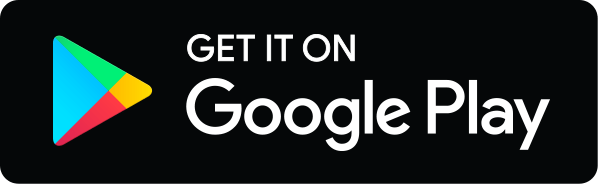
