Abstract
Over the past two decades, there have been several advances in the assessment and management of connective tissue disease-related pulmonary arterial hypertension (CTD-PAH) that improved outcomes of the treatment of this lethal disease, and this will be the focus of this study. Systemic sclerosis is the leading cause of CTD-PAH, followed by systemic lupus erythematosus, mixed connective tissue disease, idiopathic inflammatory myositis, rheumatoid arthritis, and Sjogren’s syndrome. Clinical registries have been invaluable in informing about the burden of disease, risk and prognostic factors, and temporal trends with respect to treatment and outcome in CTD-PAH. The major advances have centered on improved disease classification and diagnostic criteria, screening and early diagnosis, the emergence of evidence-based therapies including combination goal-orientated treatment strategies, and the establishment of centers with expertise in PAH.
Introduction
Autoimmune connective tissues diseases (CTD) refer to a heterogeneous group of conditions characterized by immune dysfunction, leading to end organ damage due to inflammation, endothelial dysfunction, and fibrosis. Clinical observational registries have been invaluable in describing real-life patients, treatment patterns, and prognostic outcomes of an otherwise rare and diverse group of conditions that can be complicated by pulmonary arterial hypertension (PAH), which is associated with significant morbidity and mortality. Although CTD-PAH will be described more broadly, the focus of this study is on systemic sclerosis-related PAH (SSc-PAH) as the leading cause of CTD-PAH worldwide, thereby providing a unique window to various aspects of pulmonary hypertension assessment and management.
Updated classification of pulmonary hypertension
Pulmonary hypertension (PH) refers to a hemodynamic and pathophysiological state defined by an increase in mean pulmonary artery pressure (PAP) ≥ 25 mmHg at rest as assessed by right heart catheterization (RHC), and is further subclassified according to the pulmonary artery wedge pressure (PAWP) into precapillary (PAWP ≤ 15 mmHg) or postcapillary PH (PAWP > 15 mmHg) . In general, precapillary PH develops in the setting of pulmonary vascular diseases, where obstructive remodeling of the pulmonary arteries results in elevated pulmonary vascular resistance (PVR). By contrast, postcapillary PH develops due to passive transmission of elevated PAWP without necessarily an increase in PVR.
As PH can be the consequence of different clinical conditions, the current PH classification has been further divided into key groups determined according to shared pathophysiology and approach to therapy ( Table 1 ) . Clinical groups included in precapillary PH are PAH (group 1), PH due to lung diseases (group 3), chronic thromboembolic PH (group 4), and PH with unclear and/or multifactorial mechanisms (group 5), while postcapillary PH chiefly comprises PH due to left heart disease (group 2).
1. Pulmonary Arterial Hypertension (PAH) |
1.1 Idiopathic PAH |
1.2 Heritable PAH |
1.3 Drugs or toxins induced |
1.4 Associated with: |
1.4.1 Connective tissue diseases |
1.4.2 HIV infection |
1.4.3 Portal hypertension |
1.4.4 Congenital heart disease |
1.4.5 Schistosomiasis |
1′. Pulmonary veno-occlusive disease and/or pulmonary capillary hemangiomatosis |
1′.1 Idiopathic PVOD |
1′.2 Heritable PVOD |
1′.3 Drug and toxins induced |
1′.4 Associated with: |
1′.4.1 Connective tissue diseases |
1′.4.2 HIV infection |
1″. Persistent pulmonary hypertension of the newborn (PPHN) |
2. Pulmonary hypertension due to left heart disease |
2.1 Left ventricular systolic dysfunction |
2.2 Left ventricular diastolic dysfunction |
2.3 Valvular heart disease |
2.4 Congenital/acquired left heart inflow/outflow obstruction and congenital cardiomyopathies |
3. Pulmonary hypertension due to lung disease and/or hypoxia |
3.1 Chronic obstructive pulmonary disease |
3.2 Interstitial lung disease |
3.3 Other pulmonary disease with mixed restrictive and obstructive pattern |
3.4 Sleep-disordered breathing |
3.5 Alveolar hypoventilation disorders |
3.6 Chronic exposure to high altitude |
3.7 Developmental lung disease |
4. Chronic thromboembolic pulmonary hypertension |
5. Pulmonary hypertension with unclear multifactorial mechanisms |
5.1 Hematologic disorders: chronic hemolytic anemia, myeloproliferative disease, splenectomy |
5.2 Systemic disorders: sarcoid, pulmonary histiocytosis, lymphangioleiomyomatosis |
5.3 Metabolic disorders: glycogen storage disease, Gaucher disease, thyroid disorders |
5.4 Others: tumoral obstruction, fibrosing mediastinitis, chronic renal failure, segmental PH |
Updated classification of pulmonary hypertension
Pulmonary hypertension (PH) refers to a hemodynamic and pathophysiological state defined by an increase in mean pulmonary artery pressure (PAP) ≥ 25 mmHg at rest as assessed by right heart catheterization (RHC), and is further subclassified according to the pulmonary artery wedge pressure (PAWP) into precapillary (PAWP ≤ 15 mmHg) or postcapillary PH (PAWP > 15 mmHg) . In general, precapillary PH develops in the setting of pulmonary vascular diseases, where obstructive remodeling of the pulmonary arteries results in elevated pulmonary vascular resistance (PVR). By contrast, postcapillary PH develops due to passive transmission of elevated PAWP without necessarily an increase in PVR.
As PH can be the consequence of different clinical conditions, the current PH classification has been further divided into key groups determined according to shared pathophysiology and approach to therapy ( Table 1 ) . Clinical groups included in precapillary PH are PAH (group 1), PH due to lung diseases (group 3), chronic thromboembolic PH (group 4), and PH with unclear and/or multifactorial mechanisms (group 5), while postcapillary PH chiefly comprises PH due to left heart disease (group 2).
1. Pulmonary Arterial Hypertension (PAH) |
1.1 Idiopathic PAH |
1.2 Heritable PAH |
1.3 Drugs or toxins induced |
1.4 Associated with: |
1.4.1 Connective tissue diseases |
1.4.2 HIV infection |
1.4.3 Portal hypertension |
1.4.4 Congenital heart disease |
1.4.5 Schistosomiasis |
1′. Pulmonary veno-occlusive disease and/or pulmonary capillary hemangiomatosis |
1′.1 Idiopathic PVOD |
1′.2 Heritable PVOD |
1′.3 Drug and toxins induced |
1′.4 Associated with: |
1′.4.1 Connective tissue diseases |
1′.4.2 HIV infection |
1″. Persistent pulmonary hypertension of the newborn (PPHN) |
2. Pulmonary hypertension due to left heart disease |
2.1 Left ventricular systolic dysfunction |
2.2 Left ventricular diastolic dysfunction |
2.3 Valvular heart disease |
2.4 Congenital/acquired left heart inflow/outflow obstruction and congenital cardiomyopathies |
3. Pulmonary hypertension due to lung disease and/or hypoxia |
3.1 Chronic obstructive pulmonary disease |
3.2 Interstitial lung disease |
3.3 Other pulmonary disease with mixed restrictive and obstructive pattern |
3.4 Sleep-disordered breathing |
3.5 Alveolar hypoventilation disorders |
3.6 Chronic exposure to high altitude |
3.7 Developmental lung disease |
4. Chronic thromboembolic pulmonary hypertension |
5. Pulmonary hypertension with unclear multifactorial mechanisms |
5.1 Hematologic disorders: chronic hemolytic anemia, myeloproliferative disease, splenectomy |
5.2 Systemic disorders: sarcoid, pulmonary histiocytosis, lymphangioleiomyomatosis |
5.3 Metabolic disorders: glycogen storage disease, Gaucher disease, thyroid disorders |
5.4 Others: tumoral obstruction, fibrosing mediastinitis, chronic renal failure, segmental PH |
Epidemiology of CTD-PAH
Registry data have shown that CTD-PAH is the second leading cause of PAH (∼25% of all cases), next to idiopathic PAH (∼46%) . SSc-PAH accounts for almost 75% of cases of CTD-PAH; the remaining, in order of decreasing frequency, are due to systemic lupus erythematosus (SLE, 8–19%), mixed connective tissue disease (MCTD, 8–9%), rheumatoid arthritis (RA, 3–5%), dermatomyositis/polymyositis (DM/PM, 4%), undifferentiated connective tissue disease (2%), and Sjogren’s syndrome (1%) .
Approximately 1 in 10 SSc patients will develop PAH in his/her lifetime. However, much less is known about the true incidence of SSc-PAH, with one longitudinal observational study reporting an incidence rate of 0.61 cases per 100 patients years, in 384 SSc patients with no “high risk” features for PH assessed noninvasively over a mean period of 41.03 ± 5.66 months . This relatively low incidence increases considerably among patients with significant “high risk” factors for PH (systolic PAP at transthoracic echocardiography (TTC) > 40 mmHg and/or diffusing capacity for carbon monoxide (DLCO) < 55% predicted and/or forced vital capacity (FVC)/DLCO ratio > 1.6), with a recent study reporting the frequency of PH in this population to be 10% at 2 years, 13% at 3 years, and 25% at 5 years . Data on the prevalence of PAH in CTDs other than SSc are much less reliable because of the lack of RHC-based studies, but prevalence rates using RHC have been reported in SLE (0.005–9.3%) .
Survival in CTD-PAH: poorer than iPAH
In the vast majority of patients, SSc-PAH is a progressive disease associated with significant mortality. In a recent large systematic study, inclusive of 17 studies and 2011 SSc patients with PAH, 1-, 2-, and 3-year pooled survival rates were reported at 82% (95% CI 79–85%), 67% (95% CI 63–72%), and 56% (95% CI 51–61%), respectively .
The poor prognosis of SSc-PAH patients compared to patients with iPAH has been recognized in a number of studies. In the REVEAL registry, 641 patients with CTD-PAH (including 399 patients with SSc-PAH) were compared with 1251 patients with iPAH, and were shown to have a lower 1-year survival rate (86% vs. 93%, p < 0.0001) and freedom from hospitalization rate (67% vs. 73%, p = 0.03) at 1 year, despite the CTD-PAH group having better pulmonary hemodynamics and right ventricular function on echocardiography than the iPAH group . The CTD-PAH group also had a higher prevalence of pericardial effusion, higher brain natriuretic peptide (BNP) levels (432.8 ± 789.1 vs. 245.6 ± 427.2 pg/mL, p < 0.0001), lower 6-min walk distances (6 MWDs, 300.5 ± 118.0 vs. 329.4 ± 134.7 m, p = 0.01), and lower DLCO levels (44.9 ± 18.0% vs. 63.6% ± 22.1% predicted, p < 0.0001). Compared with the more broad CTD-PAH group, the SSc-PAH subgroup had a lower 1-year survival rate (82% in SSc-PAH vs. 94% in SLE-PAH, 88% in MCTD-PAH, and 96% in RA-PAH).
Importantly, survival rates in SSc-PAH appear to have improved in recent years due to the focus on screening for and early detection of PAH, emergence of evidence-based therapies and treatment strategies, and establishment of centers with expertise in SSc and PAH. The Australian Scleroderma Cohort Study (ASCS) is a prospective, multicenter study of risk and prognostic factors for cardiopulmonary outcomes in SSc and MCTD, in which an early detection algorithm is applied; reported 1- and 3-year survival rates in the CTD-PAH group were 94% and 73%, respectively . Similarly, in the Pulmonary Hypertension Assessment and Recognition of Outcomes in Scleroderma (PHAROS) registry, 131 SSc patients with incident PAH, who were followed for a mean ± SD of 2.0 ± 1.4 years, had 1-, 2-, and 3-year cumulative survival rates of 93%, 88%, and 75%, respectively . While it is often difficult to directly compare different cohorts due to confounders (unknown or difficult to statistically correct for such as regulations that govern access to treatment/therapeutic strategies, disease trajectory, or patient adherence), it seems likely that these increased survival rates compared to previously reported SSc cohorts are attributable to screening and early detection .
In the large Pittsburgh and EUSTAR registries, SSc-PAH was the second leading cause of SSc-related mortality, next to interstitial lung disease (ILD) . In the ASCS, pulmonary disease (PAH and/or ILD) accounted for approximately 75% of SSc-related deaths and almost half of all deaths in 4 years of follow-up . SSc-PAH has been shown to be an independent predictor of mortality, conferring a twofold increased risk of death (HR 2.018, 95% CI: 1.440–2.827, p < 0.001) in the EUSTAR registry and a sevenfold increased risk of mortality (HR 7.246, 95%CI: 4.000–13.158, p < 0.0001) in the large French ItinerAIR Sclerodermie Study . The combination of ILD and PH predicts a particularly grim prognosis, with 1-, 2-, and 3-year survival rates of 77% (95% CI 70–83%), 48% (95% CI 40–55%), and 35% (95% CI 24–47%), respectively .
Several important predictors of increased mortality have been identified in SSc-PAH, including an advanced functional class of impairment at diagnosis, worse hemodynamic profile at diagnosis (increased mPAP, mean right atrial pressure and PVR, and reduced cardiac index (CI)), age > 60 years, male sex, a pericardial effusion at diagnosis, and severely impaired DLCO . These factors emphasize the clinical imperative to reduce the time to diagnosis and commencement of effective therapies. They also provide a rationale for closer monitoring of patients in poor prognostic groups, including repeated hemodynamic assessments, rapid escalation of therapy (including dual and triple therapy combinations), and enrollment in clinical trials of novel therapies and/or treatment strategies.
Diagnostic evaluation of pulmonary hypertension in CTD
The diagnostic evaluation of a patient with suspected PH requires a systematic approach to (1) confirm the presence of PH via invasive hemodynamic assessment with RHC; (2) determine the main mechanism(s) contributing PH according to the clinical classification; and (3) evaluate severity of the disease based on the degree of functional and hemodynamic impairment. Table 2 describes the key investigations that should be performed in a CTD patient presenting with suspected PH.
Investigation | Key utility and interpretation |
---|---|
Electrocardiogram | Signs of right heart strain in PAH. Ischemic changes may suggest coronary artery disease. Normal ECG does not exclude PAH. |
Chest X-ray | Enlarged central pulmonary arteries and/or enlarged right heart chambers in PAH. Parenchymal changes may suggest interstitial lung disease. Normal CXR does not exclude PAH. |
Ventilation-perfusion scintigraphy | Mismatched perfusion defects suggest thromboembolic disease. More sensitive than CT pulmonary angiogram in detecting chronic thromboembolic PH. |
Computed tomography | Enlarged main pulmonary artery and enlarged right heart chambers in PAH. Recticulation and fibrotic changes due to interstitial lung disease. Mosaic attenuation may be found in chronic thromboembolic PH. Lymphadenopathy, smooth septal lines, and centrilobular ground glass opacities suggest pulmonary veno-occlusive disease. CT pulmonary angiogram may detect stigmata of thromboembolic disease such as bands, webs, and mural thickening. |
Lung function and blood gas | Isolated reduction in DLCO is the classic pattern seen in PAH. Reduced lung volumes and vital capacity may indicate interstitial lung disease, myopathy, or extrathoracic restriction. Severe hypoxemia may suggest interstitial lung disease or pulmonary veno-occlusive disease |
Six-minute walk distance | Exercise desaturation may occur in both PAH and interstitial lung disease |
N-terminal pro-brain natriuretic peptide | Elevated NT-pro BNP can occur in PAH, left heart disease and renal insufficiency |
Echocardiography | Elevation of estimated systolic pulmonary artery or abnormal dimension of right heart chambers suggest PAH. Abnormal left ventricular systolic/diastolic function, dilated left atrium, or abnormal left-sided valvular function suggest postcapillary PH. |
Right heart catheterization | Required to confirm a diagnosis of PH and allows stratification into either precapillary or postcapillary PH. |
SSc is a particularly instructive and challenging prototype CTD for PH, as SSc patients are at risk of multiple potential causes of pulmonary hypertension, and different causes can coexist in the same SSc patient, posing diagnostic and management challenges. The most common cause is PAH (group 1) followed by PH due to left heart disease (group 2) and PH due to lung disease (group 3), with uncommon but underrecognized causes including PH due to pulmonary veno-occlusive disease (PVOD, group 1) and PH due to chronic thromboembolic disease (CTEPH, group 4) patients. In clinical practice, a systematic evaluation of these possibilities is vital to determine the relative contribution of one or more of these causes in managing a particular patient. A multidisciplinary approach is recommended given the complexities involved in the evaluation of PH in CTD .
PAH, or group 1 PH, is a clinical condition characterized by restricted flow through the pulmonary circulation, where the primary disease process is due to a vasculopathy affecting the small pulmonary arteries. The disease process is characterized by progressive remodeling of the small pulmonary arteries due to proliferation of vascular cells, leading to luminal obstruction of vessels and elevation of PVR . It is diagnosed by finding precapillary PH at RHC with a PVR > 3 Wood Units (WU) in the absence of significant lung disease and chronic thromboembolic disease.
PH secondary to left heart disease affects up to 12% of SSc patients, and is diagnosed by the finding of postcapillary PH at RHC (PAWP > 15 mmHg) . The underlying pathogenesis often reflects primary myocardial fibrosis, which is a common, but often occult process in SSc. Alternatively, left heart disease can be related to the presence of traditional risk factors for left heart dysfunction such as older age, hypertension, smoking, or renal disease .
In general, lung disease-associated PH is due to ILD in CTD, and refers to the RHC finding of precapillary PH coupled with significant ILD; significant ILD in PAH trials has been variably defined according to the extent of involvement on high-resolution computed tomography (HRCT) of the chest (usually more than mild) together with lung function testing abnormalities (TLC < 70% or FVC < 60–70%) . Importantly, only a small proportion of patients with severe ILD will actually develop PH, which is fortunate given the grim prognosis of this group . It seems likely that in a disease like SSc, a true pulmonary vasculopathy (group 1) may coexist with significant ILD, making further research into this group important.
Finally, PH due to PVOD is probably underrecognized in CTD, particularly in the setting of SSc . PVOD needs particular mention, since differentiating PVOD from PAH can be clinically challenging and PAH-specific therapy tends to be tolerated poorly by patients with PVOD. The vasculopathy of PVOD is characterized by preferential involvement of small pulmonary veins together with patchy capillary proliferation , in contrast to the predominant involvement of small pulmonary arteries in PAH. However, the invasive hemodynamic features of PAH and PVOD are indistinguishable, as both are characterized by precapillary PH at RHC . Chest HRCT is the investigation most likely to reveal underlying PVOD, and is characterized by the triad of mediastinal lymphadenopathy, smooth interlobular septal thickening, and centrilobular ground glass opacities . In addition to HRCT signs, a very low DLCO and severe hypoxemia are other features suspicious of PVOD . Features suggestive of PVOD have been found in as many as two-thirds of patients who are classified as “SSc-PAH” based on postmortem histological examination . The high prevalence of veno-occlusive disease in SSc may explain, in part, the refractoriness to targeted PAH therapy in some patients. The importance of identifying PVOD as the cause for precapillary PH depends on the fact that PAH therapy can actually precipitate severe pulmonary edema .
CTEPH can occur in CTD, particularly in the setting of antiphospholipid syndrome. Although less common in SSc, the diagnosis of CTEPH needs to be excluded in all patients with precapillary PH. In CTEPH, organized thrombi result in luminal occlusion of the more proximal pulmonary arteries . It is important to identify this condition, as it can be potentially cured surgically .
In CTDs other than SSc, the pathogenesis of PH varies according to the underlying CTD. In SLE, pulmonary vasculitis and thromboembolic disease are seen relatively more commonly. In the inflammatory myositis conditions, the extent of ILD seems to be the main contributor to PH, although higher rates of acute thromboembolism have also been noted. Relatively little is known about the pathogenesis of PH in RA.
When to refer for RHC
In general, the threshold for RHC referral should be low for patients with CTD who present with either symptoms and/or investigations that are suggestive of PH. Although recommendations are provided by current guidelines on who should proceed to RHC based on noninvasive investigations, it is important to recognize that all diagnostic tests suffer from limitations with imperfect sensitivity or specificity.
Most commonly, the suspicion of PH is raised when TTE suggests the presence of elevated PAP. PAP is usually estimated from the tricuspid regurgitation velocity (TRV) , but this measurement is subject to imprecision and may not be interpreted with confidence in 20–40% of patients who present for evaluation of PH . Other TTE features such as the size and function of right heart chambers, pulmonary artery diameter and acceleration time, and estimation of right atrial filling pressures may suggest the presence of PH and the need for prompt referral for RHC. Table 3 summarizes the approach to referral for RHC based on TTE findings in symptomatic CTD patients with suspected PH.
Tricuspid regurgitation velocity a | Ancillary echocardiographic signs of PH b | Echocardiographic risk of PH | Referral for RHC |
---|---|---|---|
≤2.8 m/s or not measurable | No | Low | Usually not required, unless other reasons to suspect PH c |
≤2.8 m/s or not measurable | Yes | Intermediate | RHC should be considered |
2.9–3.4 m/s | No | Intermediate | RHC should be considered |
2.9–3.4 m/s | Yes | High | RHC is recommended |
>3.4 m/s | Yes or No | High | RHC is recommended |
a Tricuspid regurgitation velocities of 2.8 and 3.4 m/s correspond to systolic PAPs of 36 and 50 mmHg, respectively, assuming a right atrial pressure of 5 mmHg.
b Ancillary echocardiographic signs include enlarged right heart chamber dimensions, flattening of the interventricular septum, dilated main pulmonary artery, and short pulmonary artery acceleration time.
c Other reasons to suspect PH may include unexplained dyspnea and/or low DLCO, and/or elevated NT-pro BNP.
Given the limitations of TTE, ancillary investigations can be used to determine the need to proceed to RHC. This is particularly relevant in the context of early diagnosis and screening of asymptomatic patients for PAH. Lung function parameters (DLCO) and biomarkers of right ventricular dysfunction (BNP) are useful noninvasive tools that can facilitate the triage of patients for RHC (see below on Early Detection and Screening for PAH) .
Invasive hemodynamic evaluation
RHC remains the gold standard to confirm the diagnosis of PH and to determine whether pulmonary vasculopathy (precapillary PH) or left heart disease (postcapillary PH) is the main mechanism accounting for PH . The hemodynamic criteria for PAH require the demonstration of precapillary PH with a mean PAP ≥ 25 mmHg, PAWP ≤ 15 mmHg, and PVR > 3 Wood units . Because the hemodynamic diagnosis of PAH is critically dependent on the accurate measurement of the PAWP and left heart disease is common in patients with SSc, concurrent left heart catheterization should be considered in the appropriate clinical context . This can enable direct measurement of left ventricular filling pressures and the performance of coronary angiography if coronary artery disease is suspected.
Acute vasoreactivity testing is not required for patients with CTD-PAH . This is in contrast to idiopathic, heritable, or anorexigen-induced PAH, where acute vasoreactivity is mandatory to identify vasoreactive patients who are suspected of high-dose calcium channel blockade . In the unlikely event of an SSc patient showing acute vasoreactivity (<1%), there is no evidence to support the use of high-dose calcium blockers for the treatment of CTD-PAH .
Early detection and screening for PAH in SSc
Reliance on the development of symptoms such as exertional dyspnea is insensitive for the early diagnosis of PAH, and is often confounded by other manifestations of CTD such as musculoskeletal disease or anemia . Given the high lifetime risk of developing PAH in SSc, the current availability of efficacious drug therapies, and the progressive nature of SSc-PAH, systematic screening for PAH has been currently recommended by professional bodies worldwide for patients with SSc or SSc-spectrum disorders . At present, screening has not been routinely offered to other CTD subtypes, given the low overall frequency of PAH outside the setting of SSc.
Longitudinal observational studies embedded within clinical practice have provided important insights as well as evidence that systematic screening for SSc-PAH can be associated with improved outcomes. A French study showed that SSc patients who had PAH diagnosed via a screening program had milder disease and improved long-term survival compared with those who were diagnosed via routine clinical care. In this study, a systematic and structured screening program using TTE was offered to all patients with SSc, regardless of symptoms. Patients with a peak tricuspid regurgitation jet velocity of >3 m/s or a peak tricuspid regurgitation jet velocity of 2.5–3 m/s accompanied by unexplained dyspnea underwent RHC to confirm the presence of PAH. The group that underwent systematic TTE-based screening was then compared to a control group who had PAH detected via standard clinical care. The screening cohort had significantly less severe PAH at diagnosis as measured by the New York Heart Association (NYHA) functional class (50.0% vs. 12.5% in class I or II; p = 0.036) and pulmonary hemodynamics (PVR index: 734 ± 486 vs. 1299 ± 428 dyn s m −5 m 2 ; p = 0.01). Importantly, major improvements in survival rates were observed in patients who were diagnosed through the screening program compared with those who were provided routine care. The 1-, 3-, 5-, and 8-year survival rates in the screening cohort were 100%, 81%, 73%, and 64% versus 75%, 31%, 25%, and 17%, respectively ( p = 0.0037) in the routine care cohort, allowing for the fact that lead time bias is difficult to exclude.
Although TTE remains the most widely used noninvasive modality to screen for PAH, other noninvasive markers have also showed their utility for the detection of SSc-PAH. These include lung function parameters and biomarkers of ventricular dysfunction. A low DLCO is associated with the presence of current PAH and can also predict the occurrence of future PAH in SSc patients without ILD . Patients with SSc-PAH typically have moderate-to-severe reduction in DLCO at the time of diagnosis, and the French ItinerAIR study found that a predicted DLCO of <60% was associated with a markedly increased probability of PAH (OR 9.23, 95%CI 2.73–31.15) . The Pittsburgh Scleroderma Databank showed that a reduction and/or progressive fall in DLCO could be documented 4–5 years before the diagnosis of SSc-PAH . Because DLCO is influenced by lung volumes, pulmonary vascular disease usually results in a disproportionate reduction in DLCO compared with lung volumes. Therefore, FVC/DLCO ratio [or DLCO/VA (alveolar volume)] has been postulated to be a refined lung function parameter to indicate the presence of pulmonary vasculopathy in SSc-PAH .
B-type natriuretic peptide is released from ventricular myocytes in response to increased wall tension, and has been shown to be a useful and widely available biomarker for the presence of SSc-PAH. In patients with established PAH, N-terminal pro-brain natriuretic peptide (NT-pro-BNP) correlates with the severity of pulmonary vascular disease and the degree of right ventricular dysfunction . Screening studies have documented varying NT-pro-BNP cutoff values (210–395 pg/mL) for the optimal detection of SSc-PAH , with the acknowledgment that these thresholds may potentially miss patients with very mild disease and normal right ventricular function, as NT-pro-BNP is a surrogate marker of ventricular strain. In clinical practice, NT-pro-BNP levels can also be elevated due to conditions other than PAH such as left ventricular dysfunction and advanced renal insufficiency , which can also complicate SSc.
Screening algorithms for detection of SSc-PAH
It is intuitive that the accuracy of screening algorithms for SSc-PAH can be improved by combining different tests that are predictive of the presence of PAH. Indeed, current evidence supports the use of a multimodal approach to improve diagnostic performance and guide referral for confirmatory RHC.
The ASCS investigated the combination of NT-pro-BNP and lung function parameters for the detection of SSc-PAH. A model that used a composite of NT-pro-BNP (cutoff > 210 pg/mL) together with lung function parameters (DLCO < 70% predicted with FVC/DLCO ratio > 1.8) significantly improved diagnostic accuracy compared with that using lung function parameters alone . In this proposed algorithm, patients were deemed to have screened positive if they fulfilled the NT-pro-BNP and/or lung function criteria. This proposed screening algorithm was then externally validated in 49 consecutive high-risk SSc patients who had undergone RHC for suspected PAH. The ASCS algorithm was associated with sensitivity, specificity, positive predictive value, and negative predictive value of 94.1%, 54.5%, 61.5%, and 92.3%, respectively, for the detection of PAH . It should be noted that the external validation cohort was highly enriched for PAH (17 of 49 patients had confirmed PAH) as they fulfilled at least one of the following at-risk criteria including a systolic PAP ≥ 40 mmHg on TTE and/or DLCO ≤ 50% predicted with FVC > 85%, and/or fall in DLCO ≥ 20% compared with the previous year and/or unexplained dyspnea. The particular utility of this screening algorithm is that it shifts the reliance of screening away from TTE, which can be a technically demanding and high-cost modality with potential implications for healthcare economics, and instead reserves TTE for screen-positive cases to help guide the decision to perform an RHC and exclude other cardiac causes of dyspnea .
The DETECT study was undertaken to address the need for a validated, evidence-based algorithm for screening for SSc-PAH . This was the first study where RHC was mandated in all study participants ( n = 488 enrolled with n = 466 subsequently undergoing RHC), such that the number of false-negative results could be determined . Because RHC was mandated in all participants, the investigators of DETECT stipulated the inclusion criteria of disease duration > 3 years and DLCO < 60% predicted, to avoid RHC in patients who were deemed to be at relatively low risk for PAH. The DETECT algorithm used a two-step approach to determine the need for confirmatory RHC. In step 1, six parameters (telangiectasia, anticentromere antibodies, right-axis deviation on electrocardiogram, FVC/DLCO ratio, uric acid, and NT-proBNP) were used to construct a composite score to determine the need for echocardiography. In Step 2, the TRV and right atrial area on echocardiography were quantified to determine referral for RHC. The DETECT algorithm was set to minimize false-negative cases while accepting that a substantial number of patients without PAH would be subjected to RHC. The DETECT algorithm resulted in only a very small rate of missed diagnosis of 4% ( n = 3) in comparison to a rate of 29% ( n = 24) if the 2009 ESC/ERS TTE-based recommendations were used. This study showed that an approach based on TTE alone may lack sensitivity, particularly if the aim is to identify patients with very early disease and minimally elevated PAP. Similarly, other studies have shown that a DLCO > 60% in isolation is insufficiently sensitive for the exclusion of PAH (sensitivity 74%, specificity 54%, positive predictive value 70%, and negative predictive value 50%).
On the basis of the results of recent screening studies, the new 2015 ESC/ERS guidelines recommend that a combined screening approach (using biomarkers, lung function, echocardiography) should be considered to predict the presence of PAH in SSc . For patients with disease duration > 3 years and DLCO < 60% predicted, the two-step DETECT algorithm may also be considered .
Because of the cross-sectional design of studies such as DETECT, the frequency of repeat noninvasive screening in asymptomatic SSc patients is currently unclear. Furthermore, the follow-up of patients who have screened positive but do not have PH confirmed at RHC is also unclear. However, recently published results of the PHAROS study have shown that the 2-, 3-, and 5-year rates of PH development were 10%, 13%, and 25%, respectively, in a cohort of SSc patients with a systolic PAP > 40 mmHg on TTE, and/or DLCO < 55% predicted, and/or FVC/DLCO ratio > 1.6 at baseline enrollment. The PHAROS study confirmed that SSc patients who present high-risk features for PAH require careful follow-up. At present, annual TTE, DLCO, and NT-pro-BNP have been proposed for all SSc patients , but high-quality evidence lacks supporting this approach. This highlights the need for further longitudinal studies to determine the most cost-effective and high-yield approach to detect SSc-PAH at an early stage.
“Borderline” pulmonary hypertension
Some SSc patients who undergo RHC for suspected PH will have hemodynamics that fail to reach criteria for a formal diagnosis of PH, but have the so-called “borderline PH” with an mPAP between 21 and 24 mmHg, which is not strictly normal, as the upper limit of normal is 20 mmHg .
This group of patients with mPAP between 21 and 24 mmHg may have an intermediate phenotype between overt PH and “normality.” In a recent study, patients with SSc in whom PH and significant ILD had been excluded at baseline, were enrolled in a structured follow-up program with prespecified criteria (based on new symptoms, TTE and DLCO parameters), which prompted repeat catheterization to reassess for incident PH. Of the 228 patients enrolled in this study, 86 had an mPAP between 21 and 24 mmHg at baseline and 76 patients underwent repeat catheterization, of whom 29 developed PH at an average follow-up of 48 ± 35 months . Patients with an mPAP between 21 and 24 mmHg were more likely to develop PH than patients with mPAP ≤ 20 mmHg (HR 3.7, p < 0.001). A transpulmonary gradient (mPAP − PAWP) > 11 mmHg at baseline also predicted future PH (HR 7.9, p < 0.001). The study also showed that the development of incident PAH was not benign, with a mortality of 18% within 3 years . Thus, SSc patients with an mPAP between 21 and 24 mmHg require close monitoring because of their high risk of progression to manifest PH.
Exercise PH
Exercise stress may potentially unmask early pulmonary vascular disease despite the presence of normal or near-normal resting hemodynamics . This is an appealing concept, because resting PH only develops when majority (>60%) of the pulmonary circulation has been obstructed . Patients with early pulmonary vascular disease may manifest with an abnormal increase in PAP when challenged by exercise stress . One difficulty with this concept of exercise is that there remains no current consensus on the hemodynamic definition of “exercise PH,” although accumulating data suggest that exercise PH can be diagnosed when mPAP and total pulmonary vascular resistance (TPVR) exceed 30 mmHg and 3 WU, respectively, during exercise . A UK-based registry showed that approximately 20% of an SSc-PAH population with exercise PH developed resting PAH over a 5.5-year follow-up. However, more outcome data are needed to show the prognostic implications of exercise PH, and whether PAH therapy could potentially improve exercise capacity or prevent progression to overt PAH at rest.
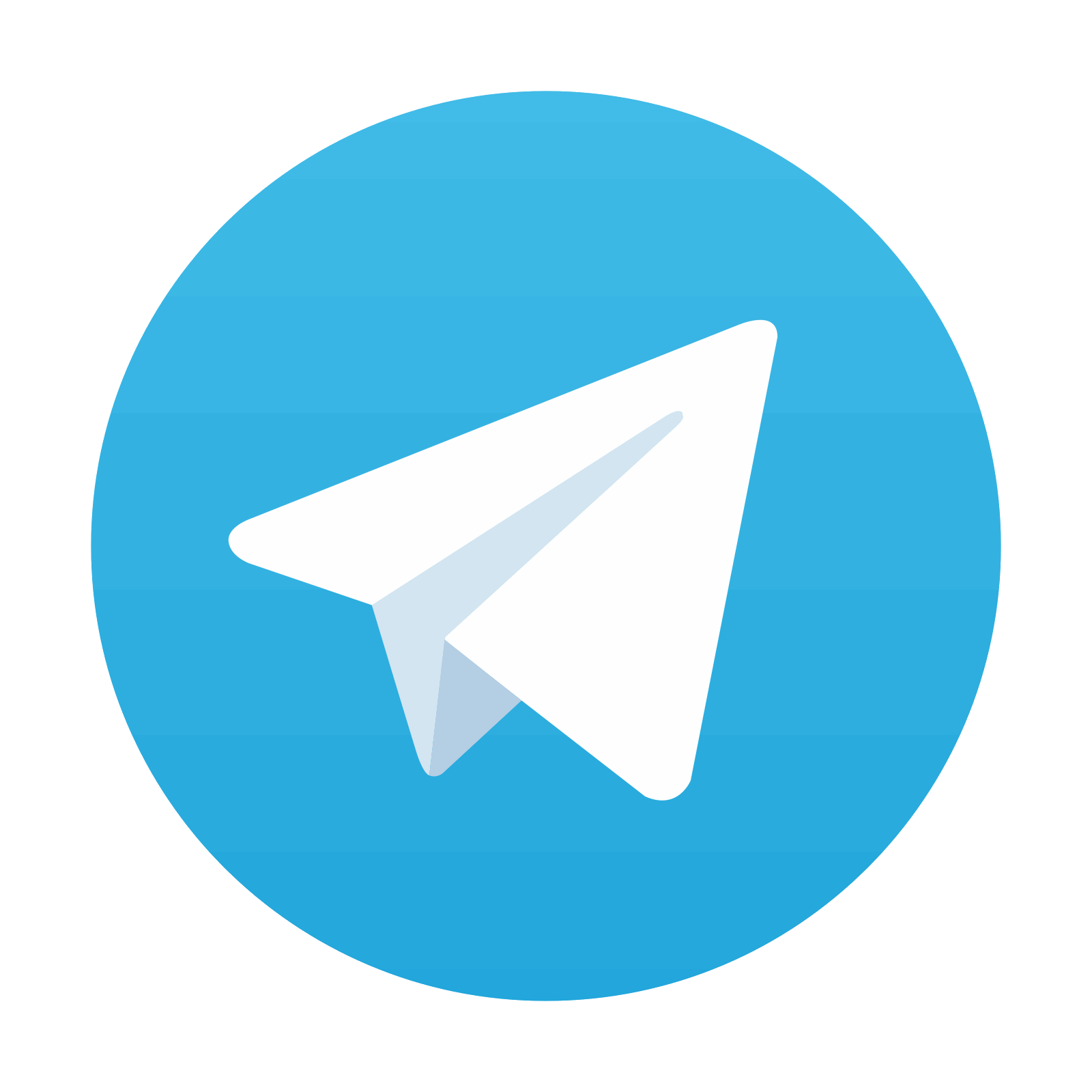
Stay updated, free articles. Join our Telegram channel

Full access? Get Clinical Tree
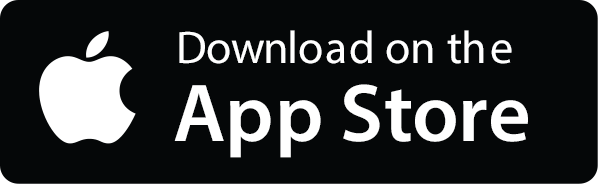
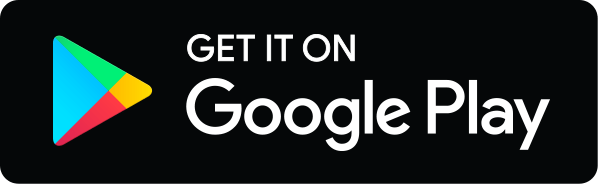