Computer Guidance
Hiba K. Anis, MD
Nipun Sodhi, MD
Joseph O. Ehiorobo, MD
Michael A. Mont, MD
INTRODUCTION
For the modern total knee arthroplasty (TKA), some of the common mechanisms of failure are malalignment and instability.1,2 Poor positioning and malalignment often lead to prosthetic loosening and subsequent revision procedures, which in themselves are associated with higher risks for poorer outcomes. Computer guidance in arthroplasty was developed in the 1990s as a strategy to improve implant alignment and therefore improve long-term implant survivorship. Following several years of research, the first computer-navigated TKA was performed in 1997 by Saragaglia in France.3 Computer guidance has since been used worldwide for TKA as well as for unicompartmental knee arthroplasty (UKA) and conversions to TKA.4
Computer assistance in knee arthroplasty can be classified as passive, semi-active, or active.5,6,7 Computer navigation is a passive system in which intraoperative measurements are made by the system to guide implant positioning; however, the surgeon is in complete control of executing the surgical plan. In semi-active systems, although surgeons maintain control of the surgical tools, bone resections are restricted to within a three-dimensional (3D) space determined by the computer software. Active computer-assisted systems plan and execute surgical tasks without intervention of the surgeon. Although they are one of the earliest systems developed, there are no active systems currently approved by the Food and Drug Administration (FDA).
Comparisons between computer-assisted techniques and conventional instrumentation in knee arthroplasty have been well-documented at several institutions since the adoption of this new technology. Many historical and contemporary studies have reported the negative consequences of improper alignment on patient outcomes and implant longevity.8,9,10 Computer-assisted arthroplasty aims to minimize the effects of surgeon-dependent variability on implant alignment by providing real-time surgical feedback to increase the precision of bone resections and implant positioning. Compared to conventional surgical techniques, computer-assisted TKAs have been shown to increase the accuracy of limb alignment to within 3° of neutral.11,12,13,14,15,16,17,18,19 In an early prospective study comparing the two methods, Bäthis et al16 found that neutral alignment was achieved in 78% of TKAs performed with conventional technique versus 96% with computer assistance.
Although computer-assisted surgeries currently account for a small percentage of knee arthroplasty procedures, there have been great advances in this field in a relatively short period of time. Given the rapid pace of technological evolution and the growing evidence of promising results, computer guidance has the potential to become a mainstay for knee arthroplasty. Therefore, it is important for orthopedic surgeons to understand the concepts underlying computer-assisted surgery and to assess its applicability to their practices. In this chapter, we will discuss the features of different types of computer guidance available for knee arthroplasty and will review the current literature on outcomes.
COMPUTER NAVIGATION SYSTEMS
While there are several available surgical applications, certain components are common to all computer-navigated TKA systems: computer platforms, tracking systems, and registration.6,20 A computer platform interprets acquired data from the surgical field and integrates it with implant data and medical images. The resultant information is displayed in real time as a 3D image on a monitor for continuous monitoring throughout the procedure. Tracked instruments are placed either on patients’ bones or on cutting block adaptors and are detected by a tracking system. Registration of these tracked instruments by the surgeon onto the computer system allows him/her to navigate the 3D anatomical model.
Computer navigation can be broadly categorized into image-based systems or imageless systems. With image-based navigation, preoperative computed tomography (CT) or intraoperative fluoroscopy can be used to reconstruct the 3D image of the surgical field for intraoperative referencing. Imageless computer-assisted surgery is currently the most widely used modality5,6 and may involve optical or accelerometer-based navigation systems.
IMAGE-BASED COMPUTER NAVIGATION
Preoperative image-based computer navigation allows for detailed operative plans including patient-specific anatomic implant matching before surgery. These systems largely consist of two key components: (1) computer-generated models from preoperative scans and (2) intraoperative matching and registration.21
With image-based systems, sagittal and axial CT scans are used to create digital 3D models of the patient’s anatomy preoperatively. Surgical planning software utilizes these models to obtain precise measurements of anatomical axes including the mechanical and transepicondylar axes as well as other key reference points. Implant sizes, implant positions, and bony resections for optimal alignment and implant contact are then calculated according to these measurements. These comprehensive virtual plans are presented for review by the surgeons preoperatively and therefore, in addition to the benefit of precise implant alignment, these modalities may also improve operating room efficiency by accurately predicting implant component sizes.
During surgery, the 3D models of the distal femur and tibia are typically displayed and are matched to the position and orientation of the patient’s anatomy following a registration process. In order to make the bony resections determined in the preoperative plan, the system uses tracking technology to guide the placement of cutting jigs.
IMAGELESS COMPUTER NAVIGATION
Imageless computer navigation systems are more widely utilized compared to image-based systems for the simple reason that the former avoids the additional costs and radiation exposure associated with additional imaging. Instead of a preoperative scans, imageless systems intraoperatively construct 3D models of the surgical field based on the patients’ osseous anatomy with both kinematic and surface registration processes. The intraoperative acquisition of information on patients’ anatomy, including bone cysts, osteophytes, and bone density measurements, is then utilized and integrated into the surgical plan and execution.
Kinematic Registration
Accurate registration of anatomical axes and landmarks is critical for a dependable virtual bone map and ultimately for the restoration of neutral limb alignment. Kinematic registration is utilized to reference important anatomic landmarks that are not exposed and usually covered by surgical drapes during knee arthroplasty, namely the centers of the femoral head and the distal tibia which are required to identify the mechanical axes of the femur and tibia, respectively.5
Surgeons can register the center of the femoral head by stabilizing the pelvis and circumducting the femur around the hip joint. This passive, circular motion of the femur effectively creates the base of a cone and the apex of this cone closely approximately the center of the hip joint. Thus, the computer algorithm integrates dynamic reference points on the femur, tracked by an optical camera, and determines the location of the femoral head center by calculating the projected apex of the circular motion. The center of the distal tibia may be referenced with both kinematic and surface registration methods.5 Multiple kinematic methods to reference the distal tibia have been described including one which estimates the center with passive movement of the ankle from dorsiflexion to plantarflexion and another treating the ankle as a ball-and-socket joint.5,22,23,24,25
Surface Registration
Surface registration involves the surgeon contacting dozens of points on the surface of the distal femur and proximal tibia with a calibrated probe. In order to limit the time spent matching reference points between the patient’s bony surface and the virtual bone map, the reference areas are limited to small patches, or point clouds, on the bone. The computer system utilizes these reference points to identify the remaining landmarks required to establish anatomical axes.
Optical Navigation Systems
Optical and accelerometer-based systems are the two most commonly used imageless computer navigation systems in knee arthroplasty. The optical navigation system has been utilized since the inception of computer-assisted knee arthroplasty and serves as the link between the surgical field and the computer platform. Two or three charge-coupled device (CCD) cameras on a separate, standalone tower are placed approximately 2 meters from the surgical field and are used as sensors to detect markers that are attached to the femur and tibia rather than the bone itself. These markers also called as “rigid bodies” can be active or passive.6,20 Active markers are light-emitting diodes, whereas passive markers are reflecting spheres both of which are detected by the camera. In passive systems, infrared flashes are sent from the camera system and reflected back by the spheres. Optical navigation systems allow a high degree of accuracy (100 measurements/second and an accuracy of <0.1 mm at 2 m)20,26 and provide detailed intraoperative data which allow for the instantaneous calculations of flexion/extension gaps, coronal alignments, sagittal alignments, and implant sizes. Disadvantages of this system include the requirement of unobstructed line of sight between the trackers and CCD, which may force surgeons to adapt their techniques, and reduced accuracy with increasing distance between the camera and trackers.
Accelerometer-Based Systems
Unlike optical systems, the more recently developed accelerometer-based navigation systems do not require the placement of pins on the femur and tibia on which markers are mounted.27 Instead, a handheld accelerometer device which displays alignment information is attached onto tibial and femoral resection instruments. Mechanical axes of the tibia and femur are determined with a combination of surface and kinematic registration. For example, to
determine the femoral mechanical axis, the device is fixed to the distal anatomic location of the femoral mechanical axis and detects the center of the femoral head with kinematic registration through multiple passive movements of the femur by the surgeon.28,29 This system does not require an unobstructed line of sight between the surgical field and a distant computer system, as it effectively places the navigation system within the surgical field. It is important to note that accelerometer-based systems can only guide distal femoral and proximal tibial resections and therefore conventional instrumentation is required for the remaining bone cuts, implant sizings, and rotational alignments.
determine the femoral mechanical axis, the device is fixed to the distal anatomic location of the femoral mechanical axis and detects the center of the femoral head with kinematic registration through multiple passive movements of the femur by the surgeon.28,29 This system does not require an unobstructed line of sight between the surgical field and a distant computer system, as it effectively places the navigation system within the surgical field. It is important to note that accelerometer-based systems can only guide distal femoral and proximal tibial resections and therefore conventional instrumentation is required for the remaining bone cuts, implant sizings, and rotational alignments.
ROBOTIC-ASSISTED KNEE ARTHROPLASTY
Robotic-assisted knee arthroplasty has been gaining attention in recent years due in part to the growing body of literature demonstrating its potential to improve the accuracy of implant positioning and therefore implant survivorship and patient outcomes.30,31,32,33,34 Depending on the specific robotic-assisted knee system, either image-based or imageless navigation is utilized to determine the surgical plan. Unlike computer navigation systems, robotic-assisted surgery integrates predetermined, patient-specific plans with haptic feedback to allow for safe and accurate surgical execution.
Once the operative plan is determined and the robotic arm or surgical instrument is registered, the surgeon is able to burr or resect bone within a defined “safety zone.” The surgeon receives haptic feedback which can be auditory, visual, or tactile, when the boundaries of this zone are approached. Thus, quantitative data from the operative plan are utilized to enforce haptic restraints in order to improve the accuracy of bone resections. Moreover, limiting the cutting tool to within an accurately determined 3D frame of reference may better protect the surrounding soft tissues and ligaments.
Robotic-assisted knee systems that utilize image-based navigation benefit from anatomic implant matching and positioning in the preoperative period. The compatibility of implant systems is dependent on whether a closed or open platform is utilized. For closed platforms, a specific manufacturer’s implant can be used. With open platforms, different implant manufacturers and designs can be used; however, a degree of specificity and predictive value may be lost in such systems.30
CLINICAL OUTCOMES
Computer Navigation
Since its inception in the late 1990s, potential advantages of computer-assisted knee arthroplasty have been extensively investigated in a wide variety of clinical studies, reviews, and meta-analyses (Table 34-1). Computer navigation has been associated with better implant alignment in TKA compared to conventional techniques.11,12,13,14,15,16,17 In a prospective cohort study of 80 TKA patients,16 neutral alignment of the mechanical axis was achieved in 96% (n = 77) of the patients in the computer-assisted cohort and 78% (n = 62) in the conventional cohort, whereby neutral alignment was defined as ±3° of varus/valgus. Moreover, neutral coronal alignment of the femoral component was achieved in 92% (n = 72) of the patients in the computer-assisted cohort and 86% (n = 69) in the conventional cohort.
In a prospective study by McClelland et al,35 radiographic and gait analyses were performed on 121 patients who underwent computer-assisted TKA (n = 42), conventional TKA (n = 39), or served as normal controls without knee surgery or pain (n = 40). Compared to the conventional group, the knee biomechanics of patients in the computer-assisted cohort more closely resembled that of a normal knee. Ideal mechanical axis alignment (180°) and the position of the weight-bearing line relative to the medial tibial border (50%) were significantly closer in the computer-assisted group (179° and 46%) compared to the conventional group (177° and 32%, P < .01 and P = .01, respectively). Upon gait analysis, it was found that patients in the computer-assisted group walked faster on average (1.24 ± 0.02 m/s) compared to the conventional group (1.14 ± 0.02 m/s, P < .01). After accounting for speed, patients in the conventional cohort had significantly less flexion during stance and swing compared to control patients with normal knees (P < .01); however, no significant differences were observed between the computer-assisted cohort and control.
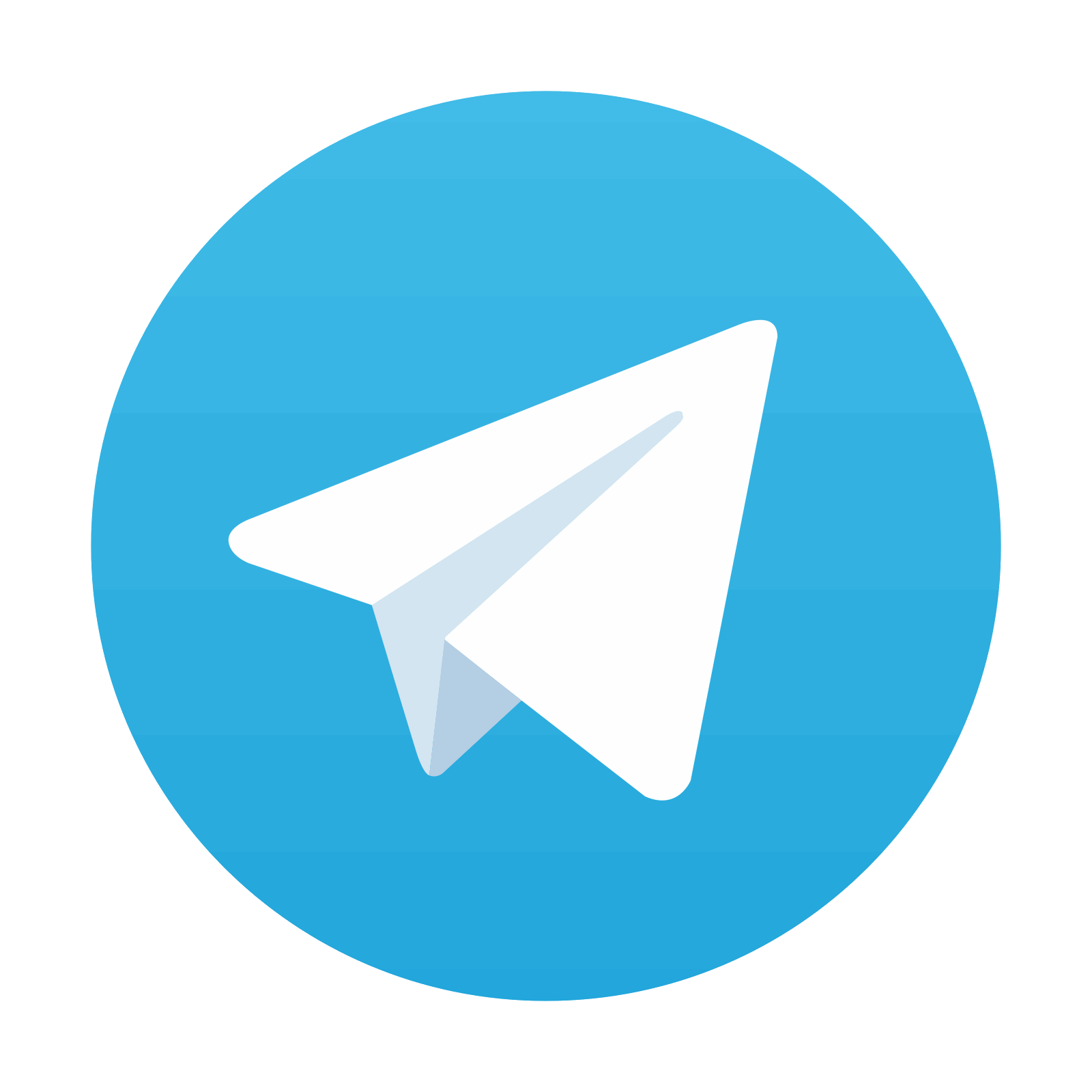
Stay updated, free articles. Join our Telegram channel

Full access? Get Clinical Tree
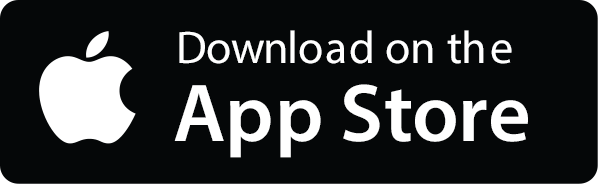
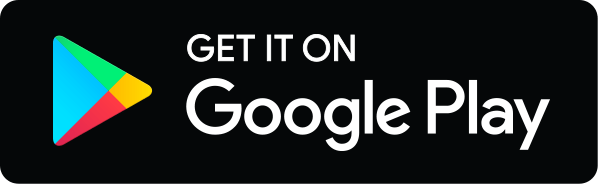
