Abstract
This position paper introduces an assessment method using staged calculation of coefficients of impairment in spastic paresis, with its rationale and proposed use. The syndrome of deforming spastic paresis superimposes two disorders around each joint: a neural disorder comprising stretch-sensitive paresis in agonists and antagonist muscle overactivity, and a muscle disorder (“spastic myopathy”) combining shortening and loss of extensibility in antagonists. Antagonist muscle overactivity includes spastic cocontraction (misdirected descending command), spastic dystonia (tonic involuntary muscle activation, at rest) and spasticity (increased velocity-dependent reflexes to phasic stretch, at rest). This understanding of various types of antagonist resistance as the key limiting factors in paretic movements prompts a stepwise, quantified, clinical assessment of antagonist resistances, elaborating on the previously developed Tardieu Scale. Step 1 quantifies limb function (e.g. ambulation speed in lower limb, Modified Frenchay Scale in upper limb). The following four steps evaluate various angles X of antagonist resistance, in degrees all measured from 0°, position of minimal stretch of the tested antagonist. Step 2 rates the functional muscle length, termed X V1 (V1, slowest stretch velocity possible), evaluated as the angle of arrest upon slow and strong passive muscle stretch. X V1 is appreciated with respect to the expected normal passive amplitude, X N , and reflects combined muscle contracture and residual spastic dystonia. Step 3 determines the angle of catch upon fast stretch, termed X V3 (V3, fastest stretch velocity possible), reflecting spasticity. Step 4 measures the maximal active range of motion against the antagonist, termed X A , reflecting agonist capacity to overcome passive (stiffness) and active (spastic cocontraction) antagonist resistances over a single movement. Finally, Step 5 rates the residual active amplitude after 15 seconds of maximal amplitude rapid alternating movements, X A15 . Amplitude decrement from X A to X A15 reflects fatigability. Coefficients of shortening (X N – X V1 )/X N , spasticity (X V1 – X V3 )/X V1 , weakness (X V1 – X A )/X V1 and fatigability (X A – X A15 )/X A are derived. A high (e.g., >10%) coefficient of shortening prompts aggressive treatment of the muscle disorder – e.g. by stretch programs, such as prolonged stretch postures –, while high coefficients of weakness or fatigability prompt addressing the neural motor command disorder, e.g. using training programs such as repeated alternating movements of maximal amplitude.
Six decades ago, Tardieu defined spasticity as an increase in stretch reflexes that could be characterized and measured by the speed required to elicit the reflexes . After these initial briskness measurements, Tardieu moved to a measurement of the angle of the muscle reaction to fast stretch . During that period, Ashworth published an ordinal scale to rate resistance to passive movement in patients with spastic paresis . In the era of botulinum toxin in particular, the Ashworth scale would become considered as a tool rating spasticity and would see its use markedly increase . Fifteen years after the publication of that scale, a complex consensus definition of the word spasticity was proposed, largely following Tardieu’s inspiration but not always understood or followed since .
As will be seen below, such a strict definition of spasticity has been useful as a way to characterize patients affected with a common syndrome; however, it failed to adequately represent the key issues that hamper function and quality of life in paretic patients. The author has given the full name deforming spastic paresis to the clinical syndrome caused by lesions involving the corticospinal pathways. This syndrome combines a neural disorder made of agonist paresis and antagonist overactivity (see below the definitions of each of these items) and a soft tissue disorder combining shortening and loss of extensibility, in muscle in particular. Sufficient data exist today to individualize this specific muscle disorder, which may be termed spastic myopathy . In the full name deforming spastic paresis, the adjective deforming finds double justification: first, patients see their bodies deformed in this syndrome, a cosmetic aggression that causes a significant component of their loss of quality life ; second, if no appropriate therapeutic program is implemented, the syndrome continues to dynamically deform bodies over time. It seems important to remind of such a fundamental component of a syndrome in its name.
After revisiting the definitions and characterizations of the key phenomena in deforming spastic paresis, this position paper revises a stepwise method to quantify their assessment, by proposing the staged calculation of four coefficients of impairment.
1
Definitions – pathophysiology – taxonomy in deforming spastic paresis
Deforming spastic paresis is thus a syndrome combining a neural disorder of motor command and a muscle disorder of extensibility loss . The neural disorder entails two components, superimposed around each joint and acting synergistically to challenge active movements: stretch-sensitive paresis in agonists and muscle overactivity in antagonists . The muscle disorder, which can be termed spastic myopathy, can be clinically subdivided into two constitutive elements, physical shortening and visco-elastic loss of extensibility, as a part of soft tissue contracture, challenging both active and passive movements . The neural and muscular disorders of deforming spastic paresis are unevenly distributed around joints, which creates force imbalances and thus deformities and asymmetrical impairments of active movement, with both muscular and neural resistances greater in attempts at moving against the more shortened muscles . These symptoms of deforming spastic paresis appear in the following order after a lesion to the central pathways of motor command execution:
- •
stretch-sensitive paresis is defined as a quantitative reduction of the voluntary recruitment of agonist motor units, further diminished by antagonist stretch . Paresis is chronologically the first manifestation of a central lesion to the motor pathways. In addition, paresis of central origin will act as the trigger of a cascade of nervous system and soft tissue adaptations, in particular muscle adaptations that will lead to stretch-sensitivity of virtually all subsequent symptoms . One example is the sensitivity of agonist paresis to antagonist stretch, which represents one of the consequences of soft tissue adaptations ;
- •
soft tissue contracture manifests itself as a two-fold clinical issue: physical shortening and – at equal length – loss of extensibility (stiffness) by increased muscle viscosity and elasticity, particularly obvious when applying high tensions . Soft tissue contracture originates in the muscle aggression represented by the immobilization (complete or partial) in short position of some muscles, which begins with the onset of paresis . Such immobilization is often insufficiently compensated, or sometimes promoted, by healthcare teams. Muscle contracture then occurs through acute modifications of gene transcription in muscle fibers immobilized in short position, with deleterious quantitative and qualitative changes . Overall reduced rates of protein synthesis and induced expression of genes for disuse atrophy promoters (REDD1, REDD2, MAFbx, MuRF1) represent changes not seen when the muscle fiber is immobilized in long position . This phenomenon follows an acute time course, as most of the contracture has actually developed by the end of the acute/subacute period, within the days and weeks following immobilization onset . Muscle contracture, which can be termed spastic myopathy, as a form of myopathy characterized by both increased muscle tension and stretch-sensitive evolution, thus represents a true muscle disorder, essentially avoidable, that comes to superimpose on the neurological disorder. Contracture becomes both the first factor of body deformity in patients with deforming spastic paresis and, through increased spindle sensitivity in the contractured muscle , a factor greatly limiting passive and active movement (see below) ;
- •
spastic muscle overactivity comprises different forms of increased involuntary recruitment of motor units, of which the following three, most often co-existing with one another, are of particular importance:
- ∘
spasticity is simply defined as an increase in the velocity-dependent reflexes to phasic stretch, detected and measured at rest . Using such strict definition, spasticity is a concept that is useful to the clinician for being both a simple marker of this patient population and a clinical parameter quantifiable at bedside (in contrast with functionally more important forms of muscle overactivity, see below), provided a valid and precise measure is used . In addition, spasticity is somewhat correlated with other forms of spastic muscle overactivity as it may partially reflect both motoneuronal hyperexcitability and spindle responsiveness . However, spasticity per se is not the main factor limiting active movement in patients with deforming spastic paresis, with the likely exception of attempts at fast or ballistic movements ,
- ∘
spastic dystonia is an excessive, chronic, tonic muscle activation of supraspinal origin, detected and measured at rest, potentially reduced after maintained stretch of the dystonic muscle . Spastic dystonia is likely related to increased involvement of brainstem descending pathways (rubro-, vestibulo-, tecto-, and ipsilateral reticulo-spinal pathways) undergoing abnormal branching onto deafferented hyperexcitable motor neurons following higher lesions . Most of these pathways are excitatory and have reduced capacities of neuronal rest, compared to the corticospinal pathway . Within the most shortened muscles, spastic dystonia comes to superimpose on soft tissue contracture to represent a second major factor of deformity in patients with spastic paresis ,
- ∘
spastic cocontraction is an excessive degree of antagonistic activation elicited by voluntary agonist command . This type of overactivity is thus revealed and measured only during voluntary command directed to the agonist; it has supraspinal origin and is aggravated by stretch of the cocontracting muscle . Antagonist coactivation actually was the first identified form of muscle overactivity in patients with deforming spastic paresis, before the term spasticity was coined . Spastic cocontraction is a critical factor of limitation – sometimes reversal – of active movement in subjects with deforming spastic paresis .
- ∘
Stretch-sensitive paresis, soft tissue contracture and spastic muscle overactivity make up the syndrome of deforming spastic paresis and represent the three main factors hindering movement in this syndrome. Reciprocal potentiation ends up developing between the muscle disorder, soft tissue contracture in the shorter of the two muscles around a joint, and the neural disorder, antagonist muscle overactivity in that muscle and stretch-sensitive paresis in the opposing muscle .
A behavioral modification typically comes to further aggravate this picture, as a self-imposed behavior of general hypo-activity, reduced social participation and therefore sensorimotor restriction emerges as a natural psychological reaction to the lesion-induced body deformities and motor limitations . Self-imposed sensorimotor restriction becomes particularly marked for the paretic limbs, as patients specifically disuse them in a behavior that was initially called functional motor amnesia by Meige and later, perhaps more accurately, learned non-use by Taub and Berman . Learned non-use of the paretic limbs – sometimes encouraged by rehabilitation systems, in particular when the care to compensate overshadows the effort to restore – is both a consequence of the paresis and a factor of its aggravation. This represents the second vicious cycle that comes to imprison patients with deforming spastic paresis . Indeed, prolonged disuse universally deteriorates central nervous system responsiveness, motor command capabilities and muscle mass regulation . In the case of a paretic limb, disuse specifically reduces cortical excitability and plasticity in the very areas that could be utilized to restore motor command to that limb .
2
From a stepwise, quantified, clinical assessment to staged coefficients of impairment
When considering the main factors of movement limitation that are reviewed above, one comes to recognize that:
- •
most of the movement-limiting factors are antagonist resistances (soft tissue contracture, spastic dystonia, spastic cocontraction, spasticity);
- •
at least three of the more important factors, paresis, spastic dystonia, spastic cocontraction, are not clinically ratable, taken individually.
Under these circumstances, a stepwise strategy of clinical evaluation is proposed here, that leads to the definition of four coefficients of impairment around each joint. This strategy provides both quantitative measurements and a rationale to guide multifocal treatment. According to this strategy, clinical evaluation of deforming spastic paresis may be first quantified using five steps, among which Steps 1 to 3 are already validated . This stepwise quantitative assessment and the derived coefficients may then be recorded on a log sheet, of which examples are proposed in Appendix 1 (upper limb) and Appendix 2 (lower limb) .
2.1
Step 1
Assessment begins with an objective evaluation of active (or passive in most severe cases) functional performance at the clinic. This initial step will actually guide both the subsequent analytical evaluations (steps 2 to 5) and the therapeutic choices of neurorehabilitative techniques, including potentially transient focal muscle weakening.
In the lower limb, active function, if ambulation is possible, may be measured by ambulation speed over 10 meters or maximal walking speed over 2 minutes, which have excellent ecological validity in particular . Among the various possible testing modalities (barefoot or with shoes, with or without assistive device, at comfortable or fast speed, including or not sit-to-stand, u-turn and stand-to-sit), one that may both serve as a better indicator for real life functional mobility and carry higher sensitivity to change should involve imposing maximal strain on the neural motor system . This can be achieved by providing minimal assistance to the patient, for example testing gait barefoot without assistive device if possible, at maximal rather than comfortable speed, and including sit-to-stand, u-turns and stand-to-sit (modified up-and-go test) . For example, a 10-meter ambulation test at maximal speed, barefoot, starting and ending seated, has been the most sensitive to change (more sensitive than comfortable speed barefoot tests or tests with shoes) after therapeutic interventions involving neurorehabilitation programs (guided self-rehabilitation contracts) and repeat injections of neuromuscular blocking agents . In addition to quantifying gait speed, step length and cadence, such gait tests lend themselves to visual observation, which may also allow to qualify the gait patterns. When observing proximal lower limb activity in spastic paresis in particular, it is possible to define an “anterior pattern”, in which overactivity in quadriceps (resisting passive knee flexion during the swing phase) predominates over that in hip extensors (hamstrings and gluteus maximus, resisting active hip flexion in swing). Anterior patterns are characterized by relatively preserved step length but slow step speed on the paretic side (leading to a particularly slowed gait cadence), a lack of hip extension at late stance (due to rectus femoris shortening/overactivity), preserved knee reextension at late swing, and a degree of knee flexion that remains below 90° in a test of rapid alternating hip flexions. Anterior patterns are commonly seen following ischemic brain lesions in adult patients and typically point to the need to reduce quadriceps resistances to knee flexion. At the other end of the spectrum, “posterior patterns” are produced when overactivity in hip extensors (hamstrings and gluteus maximus) predominates over that in quadriceps. Posterior patterns are characterized by markedly reduced step length on the paretic side, preserved hip extension at late stance, insufficient knee reextension at late swing (due to hamstrings overactivity), and a knee flexion that systematically passes 90° in a test of rapid alternating hip flexions (backward “leg recall”). Posterior patterns are commonly seen after spinal cord lesions (traumatic or inflammatory) or in infant paresis, although in the latter patterns are often mixed and typically point to the need to reduce mainly hip extensor resistances to hip flexion.
In the upper limb, for optimal ecological validity one may elect to use a scale of active function directly testing everyday life tasks, such as the Modified Frenchay Scale . The scale involves video recordings of six bimanual and four unimanual everyday tasks, which may be stored in the patient’s file and serve later as references . After such active task evaluation, one may also assess the patient’s subjective opinion as to the usefulness and the daily use of the paretic upper limb (subjective evaluation questionnaires such as the Global Subjective self-Assessment, the Disability Assessment Scale or the Motor Activity Log) as well as the daily use of performances in real life (actimetry, pedometry) .
The subsequent four steps represent attempts at explaining the functional results obtained at step 1. These tests focus on assessing each muscle group as a potential antagonist, quantifying resistances from it, instead of assessing agonist command as in peripheral disorders . Each of these steps measures an angle, from zero being the theoretical position of minimal stretch of the tested antagonist . The first two angle measurements (step 2 and step 3) constitute what the author has previously developed as the “Tardieu Scale” . These two angle measurements have been shown to hold good to excellent intra- and inter-rater reliability, both in children and adults, without the use of a goniometer .
2.2
Step 2
The objective is to assess passive soft tissue extensibility. The test is thus only completed after ensuring that the patient optimally rests the tested muscles immediately prior . One visually (without goniometer) measures the functional muscle length (or maximal passive range of motion) for each tested muscle group. This is done by exerting the slowest and strongest possible stretch on the muscle group and associated soft tissue (fascia, tendons, ligaments, capsules, skin, nerves, vessels) to move the limb segment as far as possible without causing pain or jeopardizing soft tissue integrity. The rationale behind using extreme slowness is to minimize recruitment of stretch reflex afferents, which might create a spastic reaction that would impact on the measured amplitude . The rationale behind using strong stretching force (maximal force for the examiner, compatible with preserving soft tissue integrity) is to optimally overcome the spastic dystonia present in the assessed muscle group, such that this type of overactivity be minimally involved in explaining the angle of arrest. The measured angle may be termed X V1 (V1, slowest velocity) . In effect, X V1 (functional muscle length) represents the amplitude that active movement against the tested antagonist should reach in theory, if the disorder were limited to muscle. Obviously, various non-muscular underlying joint alterations may impact on X V1 , such as arthritis, capsulitis, or immobilization-induced joint retractions. However, these pure joint limitations should not in general cause the major part of X V1 limitations . In addition, X V1 may not represent pure muscle or joint contractures as it might also reflect extensibility losses in fascia, aponeuroses, skin, vessels, etc. . These limitations are acknowledged, hence the proposed term functional muscle length. Depending on the degree of invincible contracture and spastic dystonia, X V1 may be more or less remote from the expected anatomical angle at the tested joint, a “normal” angle that may be called X N (using standard anatomical values or an estimation based on the contralateral side in hemiparetic patients). The ratio (X N – X V1 )/X N may be useful as the coefficient of shortening (“functional” shortening) of the tested muscle.
2.3
Step 3
The objective is to assess the response of the tested muscles to their own stretch (spasticity), which reflects both motoneuronal excitability and increased transmission of the stretching forces to the spindles . The maneuver is thus also performed after ensuring that the patient optimally rests the tested muscles immediately prior . One may even use a few brisk movements in the opposite direction prior to the test, to optimize rest in the tested muscle . The test maneuver then consists in stretching the muscle group of interest as fast as possible until a catch is felt. If the catch repeatedly and reliably occurs before functional length (X V1 ) is reached and is followed by a clear release, the visually measured (without goniometer) angle of catch then represents the threshold for a transient resistance that is due to an elicited stretch reflex. Occasionally the catch is not followed by a clear release but still reliably occurs at an angle that is different from – and smaller than – the muscle functional length X V1 . The absence of clear release may then be explained by residual spastic dystonia in the tested muscle or by the encounter of visco-elastic passive resistance from other muscles of the tested muscle group, which have not reached their stretch reflex threshold. If the catch is followed by a release and then a re-catch, this is called a clonus. The angle of catch that is obtained using this maneuver is called X V3 (V3, fastest possible velocity for the examiner). Depending on the presence or absence of a catch or a clonus, one may also determine a spasticity grade, noted as Y, an ordinal variable . The determination of X V1 , X V3 and Y corresponds to what the author previously developed and validated as the Tardieu Scale . Historically, the Tardieu scale was named and proposed in 2000 , following Held and Pierrot-Deseilligny early attempts at standardizing Tardieu’s historical method of clinical examination into a scale . Here, the ratio (X V1 – X V3 )/X V1 may serve as the coefficient of spasticity, which quantifies spasticity regardless of the functional length of the muscle.
2.4
Step 4
The objective is to assess the capacity of agonist activation to overcome passive and active resistance of the tested muscle group (antagonist). One thus asks the patient to accomplish one movement of maximal amplitude against the tested muscles. Unlike for the measures of X V1 and X V3 , for which the goniometer does not improve reliability , it is recommended to use a goniometer to measure this active angle. The amplitude reached at the arrest of the active movement represents balance between the forces generated by agonist activation and those related to passive (stiffness/increased visco-elasticity) and active (spastic cocontraction) resistances opposed by the tested muscle group. The measured angle may be called X A , maximal active amplitude against the tested muscle, over one single movement. Its improvement may be a primary objective of the neurorehabilitative techniques selected. The ratio (X V1 – X A )/X V1 is the coefficient of weakness, which measures impairment of active command against the tested antagonist, regardless of its functional length.
2.5
Step 5
The objective is to assess the repeatibility of overcoming passive and active resistances of the tested muscle . One asks the patient to accomplish as many movements against the tested muscle in a given time (15 seconds for example), keeping amplitude as large as possible on each movement. For each tested antagonist, the maximal active amplitude still reached at the end of the series and the mean movement frequency are recorded. The final maximal amplitude may be called X A15 , amplitude reached against the tested muscle after 15 seconds of maximal active movement repetitions. The amplitude decrement from X A reflects fatigability of performance, essentially of central origin . The ratio (X A – X A15 )/X A is the coefficient of fatigability, which quantifies amplitude decrement over a 15-second series, regardless of the maximal amplitude reached over a single movement.
Its improvement, as well as that of the maximal frequency of alternating movements, may correlate with real life functional enhancement and should be another goal of neurorehabilitation in spastic paresis. Validation of the latter two steps (4 and 5) requires further studies.
Taking these assessment steps may help clarify which of the muscle or the neural disorder predominantly affects a particular antagonist . A predominant muscle disorder would be revealed by a particularly high coefficient of shortening while a prominent neural disorder may be detected by a high coefficient of weakness or of fatigability. Depending on the situation, treatment may then focus more on muscle lengthening or on motor training, possibly using advanced technologies , or both.
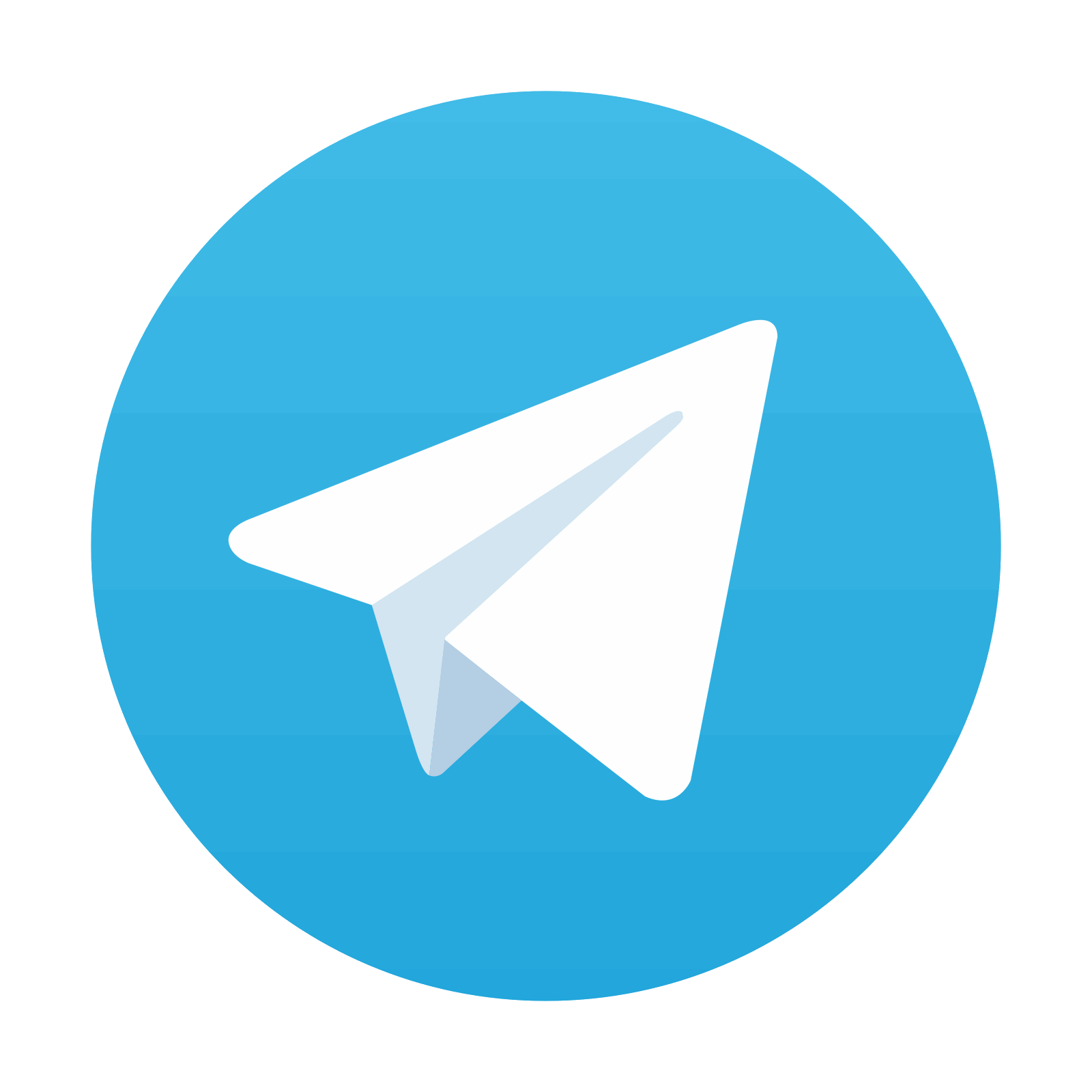
Stay updated, free articles. Join our Telegram channel

Full access? Get Clinical Tree
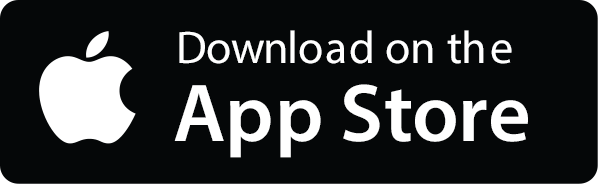
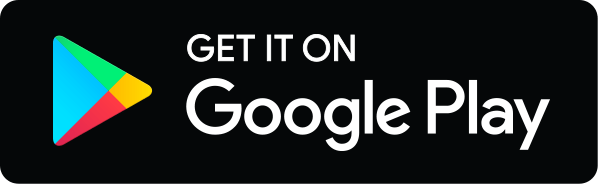