- ▪
Nerve conduction studies (NCS) and electromyography (EMG) provide objective, localizing, prognostically important data about clinically suspected lesions.
- ▪
NCS and EMG also provide peripheral nerve system context about concurrent mononeuropathies, polyneuropathy, myopathy, and other neuromuscular disorders.
- ▪
NCS are generally more important in diagnosing nerve entrapments and injuries, but needle EMG is necessary to most studies for the complementary information it provides.
- ▪
Both NCS and EMG measure electrical potentials generated by nerves and muscles.
- ▪
Optimal neurophysiologic consultation is a process that calls for excellent communication between neurophysiologist and referring clinician before and after the study, as well as between patient and neurophysiologist during the study.
NCS and EMG rank second in importance only to obtaining a careful history and performing a neurologic examination for accurate and localizing diagnosis of nerve entrapments and disorders of the peripheral nervous system (PNS). Unlike clinical tests, such as Phalen’s sign, that focus on specific nerve entrapments such as carpal tunnel syndrome (CTS), , well-designed NCS and EMG provide not only objective, localizing, prognostically important data about the clinically suspected lesion but also indispensable PNS context about concurrent mononeuropathies, polyneuropathy, myopathy, and other neuromuscular disorders. Neurophysiologists should provide prompt, understandable, “bottom line” answers that define PNS lesion and context in terms of the referral question and neurologic exam. The optimal neurophysiologist is a physician , fellowship-trained and board-certified in electrodiagnostic medicine, adept in sophisticated examination and neuromuscular pattern recognition, and flexible enough to consistently perform studies tailored to each patient’s circumstance. This chapter develops a practical framework to enable referring clinicians and hand therapists to correlate clinical results and judge the quality of NCS and EMG reports.
Clinicians ordering studies seek pragmatic answers that will help their patients. Is CTS or another entrapment present? How severe is it? What is the precise localization? Are there atypical features or concurrent disorders such as radiculopathy or polyneuropathy? Do the neurophysiologic findings explain the patient’s symptoms and signs, or are they at least consistent with them? Do they indicate additional or unsuspected diagnoses, or reveal a need for radiologic or other testing? Do the study design and results support the final interpretation logically and scientifically? Numerous textbooks, reviews, and courses are available with background information on neurophysiologic theory and techniques. Patients are best served when referring clinicians and neurophysiologists communicate on the basis of mutually understood interpretative principles, including what NCS and EMG actually test, their limitations, and timing considerations crucial to meaningful conclusions. The purpose of this chapter is to elucidate that indispensable conceptual framework.
Nerve Conduction Studies
General Principles
NCS are generally more important in diagnosing nerve entrapments and injuries, but needle EMG is necessary to most studies for the complementary information it provides. Both tests measure electrical potentials generated by nerves and muscles. In sensory and motor NCS, a square-wave stimulus in milliamps (mA) is delivered, usually percutaneously, to test points along the nerve, causing it to depolarize. The patient feels an instantaneous shock but usually not the wave of induced depolarization as it travels up and down the nerve itself. NCS measure voltage potential differences between two electrodes, the first recording over a nerve or muscle at an informative site at some distance from the stimulation point, the second a “neutral” reference. Recording electrodes are placed for sensory NCS over the nerve, and for motor NCS mostly over compact, usually distal superficial muscles. NCS parameters include amplitude (µV for sensory, mV for motor), distal latency (DL, in msec) from the most distal point of stimulation, and conduction velocity (CV, in m/sec) calculated in nerve segments demarcated by the selected points of stimulation. Although exceptions occur after nerve injury (to be discussed later), as a rule of thumb, the size (amplitude or area) of the NCS response is proportional to the quantity (not number) of measurable axons, and the speed (CV, DL) may provide insight into the integrity of myelin over the nerve segment tested.
Templates for Interpretation of Nerve Conduction Studies
The primary objectives of NCS are to localize the nerve lesion, characterize it as predominantly caused by axon loss (AL) or demyelination, determine the severity and prognosis, facilitate surgical planning when appropriate, and monitor the pace of nerve regeneration.
In normal nerve, the DL, amplitudes, and segmental CV are normal ( Fig. 15-1A ). With loss of large sensory or motor axons (AL), the amplitude of proximal and distal responses is reduced and CV may be normal or mildly slowed, but not more than 20% below the lower limit of normal ( Fig. 15-1B ). Examples of conditions with AL include focal mononeuropathies and most polyneuropathies. Demyelinating patterns include significant synchronous slowing (SS) occurring diffusely or focally ( Fig. 15-1C–E ); conduction block (CB) or “neurapraxia,” which may be partial or complete ( Fig. 15-1F, G ), in which the proximally elicited response is smaller than distal; and temporal dispersion (TD), in which the proximal response has significantly longer duration and an often irregular shape ( Fig. 15-1H ). Examples of demyelination include SS across the wrist in CTS and across the elbow in ulnar neuropathy, CB at or near the elbow in some ulnar neuropathies and radial nerve compression at spiral groove, and TD in acquired demyelinating polyneuropathies such as Guillain–Barré syndrome (GBS) and chronic inflammatory demyelinating polyneuropathy (CIDP). In entrapments, sensory fibers may show one pattern (e.g., AL), and motor fibers another (e.g., SS).

Conduction Slowing, Demyelination, and Remyelination
NCS patterns suggesting primary demyelination include significant SS, TD, and CB that meet published criteria. All may be seen in acquired demyelinating neuropathies, but only SS is seen in hereditary acquired polyneuropathies such as Charcot–Marie–Tooth disease, type 1. Primary demyelinating-range slowing is not necessarily seen in a nerve segment with TD, unless SS also is present among the conducting fibers. As a rule of thumb, when amplitudes are normal or nearly so, slowing suggests primary demyelination when DL (or long latency motor “F waves” to and from the spinal cord) is prolonged 30% or more above the upper limit of normal, or CV is reduced 30% or more below the lower limit of normal. (A common interpretive error, based on ignoring these criteria, takes any slowing of CV to be evidence of demyelination). CB and AL are more likely to correlate with symptoms than SS or TD, especially in motor axons. SS and TD may be asymptomatic, although mild degrees of both may be seen with AL of large myelinated axons over long nerve segments. (A common example is CTS with motor AL; median forearm CV is decreased due to large-fiber AL.) Muscles supplied by nerves showing TD are not weak, unless significant AL or CB is also present.
Demyelination may be the first effect of a pathologic process such as nerve entrapment that can progress to AL. Primary demyelination may be reversible and AL mitigated or avoided if the focal entrapment receives timely decompression or if acquired generalized GBS or CIDP is treated effectively with immunotherapy. By contrast, other mechanisms of injury and intrinsic disorders of axons cause primary AL, which disrupts the axon–myelin interface, resulting in secondary myelin loss. Although most nerve lesions have elements of both AL and demyelination, and NCS evidence is not as certain as biopsy evidence, clinicians should expect neurophysiologists to try consistently to specify the predominant pathologic process in most neuropathic cases. (Neurophysiologists equivocate unnecessarily when they habitually report “demyelination and axon loss” in all cases.)
Precise localization requires focal SS or CB over a short nerve segment. The classic example is CTS, for which current practice guidelines stress sensitivity of sensory NCS over short nerve segments (median nerve stimulating in the palm, recording proximal to the wrist). Recent literature favors internal comparison studies between adjacent nerves over identical distances to mitigate the effects of cold on DL and CV (below 32°C in hand). Typical internal comparison studies in CTS include sensory comparisons (median vs. ulnar nerve palm to wrist and wrist to ring finger, and median vs. radial wrist to thumb) and motor comparisons (median vs. ulnar wrist to lumbrical-interosseus). Sensory internal comparisons are useful in avoiding false positive CTS due to borderline DL measured from wrist to fingers. When one internal comparison is borderline (e.g., median vs. ulnar palm to the wrist, normal difference < 0.4 msec), adding three comparisons to calculate a “combined sensory index” improves sensitivity and specificity (normal < 0.9 msec).
Slowing in many nerves suggests a generalized polyneuropathy, making it difficult to be sure that any focal slowing seen is disproportionate enough to attribute to superimposed entrapment (e.g., CTS in diabetic polyneuropathy, where motor internal comparisons may help). Focal slowing may confirm demyelination (or not meet criteria) and may occur in a symptomatic or asymptomatic location (e.g., ulnar slowing across the elbow). Focal slowing demonstrable across multiple points of entrapment, common and uncommon, symptomatic or not, suggests the autosomal dominant hereditary neuropathy with liability to pressure palsies (HNPP), for which a genetic test is available.
Conduction block is present when amplitude and area of waveforms obtained by stimulating proximal to the lesion is at least 20% less (ideally 50%) than the waveform obtained stimulating distal to it, provided the duration of the proximal response does not exceed the distal by more than 15%. Temporal dispersion is present when the duration of the proximal waveform exceeds the distal by more than 15%, regardless of any amplitude change. Like the velocity criteria for demyelination, these rules apply only when response amplitudes are near normal. Larger amplitude drops are required proximally to confirm CB when distal amplitudes are low. Given that motor responses are orders of magnitude larger than sensory ones, it is technically easier to show CB in motor conductions.
Before diagnosing CB, neurophysiologists should rigorously exclude technical errors and trivial abnormalities to minimize false positives. When apparent CB is noted between two standard stimulation points separated by a long nerve segment, it may be useful, when possible, to stimulate short segments in between to pinpoint the lesion. For example, with ulnar CB at elbow, “inching” the stimulator in 1- to 2-cm steps along the nerve may precisely localize CB at the humeroulnar aponeurosis or retrocondylar space, thus providing information far more useful to the clinician than just reporting “ulnar neuropathy with neurapraxia across the elbow.” , Anatomic variants affect interpretation. For example, a Martin–Gruber anastomosis may mimic ulnar motor CB in the forearm, manifested by a significantly larger ulnar wrist-to-hand than elbow-to-hand motor amplitude and confirmed by stimulation of the median nerve at the antebrachial space Technical errors include percutaneous stimulation too far from an intended deep nerve target (e.g., obesity, edema, intervening muscles), resulting in insufficient current reaching the nerve (mimicking CB) and the “virtual cathode” effect (CV errors due to an uncertain depolarization site and inaccurate distances). When very distal CB is possible, it may help to stimulate distal to the standard distal sites. For example, before accepting low median sensory or motor amplitudes as evidence of AL in CTS, based on standard stimulation proximal to the wrist, midpalmar stimulation or inching across the wrist may show a significantly larger digital sensory or motor response amplitude consistent with focal CB instead.
Time Dependence of Wallerian Degeneration
To distinguish between AL and CB, it is crucial to recognize that Wallerian degeneration (WD) after AL (including axonotmesis) is an active, time-dependent process. The key indicator of AL after nerve injury is what happens as a function of time after injury to the response amplitude elicited distal to the lesion compared with the response amplitude elicited proximal to it.
Immediately after nerve injury before WD has taken place, the distal amplitude is normal and the proximal is reduced or absent, reflecting conduction failure and resembling the CB pattern (see Fig. 15-1F, G ). If pure demyelinating CB is present, axon continuity is preserved through the lesion and the CB pattern persists until remyelination occurs. If pure AL is present, the distal stump undergoes WD, and the distal amplitude declines to match the already low proximal amplitude, resulting in an AL pattern (see Fig. 15-1B ). Although the time to WD is similar for motor and sensory axons, it takes 3 to 4 days to observe a decline in distal motor amplitudes after AL lesions versus 10 to 12 days for distal sensory amplitudes. The difference is explained by neuromuscular junctions (NMJs) interposed between stimulated nerve and muscle. Axonotmesis disrupts axonal trophic support of muscle mediated by slow axon transport, so NMJ failure precedes WD, causing motor amplitude drop. WD is a stump length-dependent process, seen sooner in lesions near muscle.
When NCS are done immediately after nerve injury and before WD occurs, particularly when nerve transection is suggested, nerve conduction across the lesion proves at least partial continuity . Immediate NCS after injury provides a baseline for follow-up studies, but cannot define the status of nonconducting axons across the lesion, whether caused by AL, CB, or both. Repeat studies are essential after WD becomes apparent in NCS (10–12 days) and EMG (3 weeks). In general, the amount that serially documented amplitudes decline from baseline is proportional to the amount of AL.
Sensory Nerve Conduction Studies, Injury, and Regeneration
Sensory nerve action potential (SNAP) is a summation of normally synchronous waves of depolarization traveling in large sensory axons as they pass the recording electrodes. Large sensory axons carry electrical impulses to the spinal cord that the brain interprets as touch, proprioception, two-point discrimination, vibration, and higher-order perceptions. Conventional sensory NCS do not record encoded impulses, actual perceptions, or depolarization of small axons (c-nociceptors). Nor does SNAP count the sensory axons. (Separate quantitative sensory tests [QST] are available to measure vibratory thresholds in large axons and thermal and pain perception in small axons.)
Because peripheral axons are living structural components of sensory neurons in dorsal root ganglia (DRG) located in or near spinal neural foramina, a recordable SNAP confirms continuity of large DRG neurons with their peripheral processes. In the arms, the “peripheral,” or postganglionic, process of DRG cells traverses the brachial plexus and peripheral nerves. SNAP does not test the “central,” or preganglionic, process, which traverses spinal roots to enter the spinal cord. A reduced or absent SNAP amplitude is not a localizing finding, because it suggests postganglionic AL at or distal to DRG, usually the loss of large DRG peripheral processes (mononeuropathies, polyneuropathies), but sometimes the loss of parent DRG cells (sensory neuronopathy). An abnormally low SNAP amplitude indicates partial loss of large DRG processes or cells, assuming delivery of adequate (supramaximal) stimulation. A normal SNAP amplitude proves there are “enough” large sensory axons, defined by published norms. A low-normal SNAP in a symptomatic dermatome or nerve territory calls for a side-to-side comparison, because a twofold or greater asymmetry is abnormal.
SNAPs are fraught with potential technical and interpretive pitfalls. First, with side-to-side comparisons, both sides may be affected (e.g., CTS). Second, although SNAP amplitudes usually are more prominently affected than motor potentials after axonotmesis, testing in the 5- to 9-day window after postganglionic AL is confusing because a reduced compound motor action potential (CMAP) but normal SNAP amplitude falsely suggests a preganglionic lesion. Third, a postganglionic lesion (e.g., plexopathy) does not exclude a concurrent preganglionic lesion (e.g., radiculopathy), especially when the injury may involve both (e.g., motorcycle traction injury to arm). Finally, when neurophysiologists approach injured nerves with an inflexibly preconceived notion of what they will find, they may erroneously accept a reduced or absent sensory response as confirmatory. Common examples include an absent ulnar sensory response stimulating wrist recording from the small finger in suspected ulnar neuropathy at elbow, and an absent median sensory response stimulating wrist recording from the middle finger in suspected CTS. The most circumspect interpretation of each of these isolated findings, respectively, would be a partial AL lesion at or distal to the C8 and C7 DRGs in either brachial plexus or peripheral nerves. Particularly in ulnar neuropathy, for which there are many potential sites of focal injury from axilla to its terminal branches, the burden of localization shifts from low SNAP to motor NCS and EMG.
Clinicians approach sensory symptoms thinking of the cutaneous distributions of spinal roots (dermatomes) and peripheral nerves. To understand what SNAP actually tests, it is crucial to recall that dermatomal symptoms can arise from preganglionic axons, postganglionic axons, or both. For example, C8 radiculopathies and lower-trunk plexopathies may cause similar distributions of distal sensory symptoms. With preganglionic C8 radiculopathies, postganglionic C8 axons are intact, so SNAPs are normal when recorded in the C8 dermatome, however numb the skin (e.g., ulnar nerve recording of the small finger, or dorsal ulnar cutaneous nerve recording of the dorsomedial hand). With lower-trunk plexopathies, similar sensory symptoms on the medial forearm or small finger could correspond with SNAP amplitudes that are absent, reduced in absolute terms, low-normal but less than half contralaterally, or normal if symptoms arise from irritation of large C8 postganglionic processes without AL or selective irritation or injury to c-nociceptor axons in the C8 dermatome.
Sensory nerve injuries recover through remyelination (over weeks to months) or regeneration of cut axons (1 inch/month or 1–2 mm/day). Reduced sensory amplitudes mean either that stimulation and recording distal to the point of actual partial CB is technically impossible or that the nerve is not there. As CB lesions remyelinate or large axons regenerate, SNAP amplitudes increase. However, sensory (and motor) axon sprouts may not reach their original target due to neuroma formation, blockage by scar, misdirection (aberrant regeneration), or regeneration failure (e.g., certain toxic neuropathies).
Motor Nerve Conduction Studies, Injury, and Regeneration
Interpretation of CMAPs uses similar principles and “templates” as for SNAPs, but several differences should be recognized. Even though both test living peripheral nerve systems, the proximal anatomy of the “motor unit” differs from the large DRG and peripheral process sensory system in diagnostically important ways . First, different sites of lower motor neurons (LMN) and DRG neurons aid in localization. LMN (anterior horn cells) are clustered in the spinal cord ventral horn, proximal to both spinal roots and sensory neurons located in the DRG at or near the spinal neural foramina. SNAPs do not reflect sensory root injury in cervical radiculopathies. For both motor NCS and needle EMG, neurophysiologic testing of the living “motor unit” includes LMNs, motor root and axons, NMJ, muscle membrane, and muscle fibers. Significant motor AL at LMN or root levels may be reflected as abnormal motor NCS (see Fig. 15-1B ), EMG, or both. Because muscles are supplied by more than one motor root, acute AL in a single root must be severe to reduce CMAP amplitudes recorded distally, although side-to-side CMAP comparisons may be helpful. Second, given the rather different locations of anterior horn cells (AHC) and DRG, abnormalities in CMAP or EMG together with normal SNAP from the same spinal segment define a preganglionic lesion. Although interpretations should pass the test of reasonableness, mutually reinforcing CMAP, EMG, and SNAP findings that align to suggest a preganglionic rather than an expected postganglionic lesion should be taken quite seriously. When clinical and neurophysiologic localization appear to disagree, the neurophysiologist has a responsibility to consider the timing of the study relative to WD, additional testing (side-to-side, specialized, and serial), unexpected concurrent lesions, and even neuromuscular diseases. Occasional patients with painless thenar atrophy with suspected CTS show expected low-amplitude of the abductor pollicis brevis, but on EMG are found to have generalized motor neuron disease.
Although the rates of WD and axon regeneration are similar for sensory and motor axons, the distal anatomy of the “motor unit” and recording site for CMAP relative to it differ significantly from the sensory system as reflected in SNAP. First, unlike SNAP, which amounts to a “snapshot” of a traveling wave of depolarization passing under the recording electrodes over a “named” nerve, the CMAP represents the potential difference between a recording electrode on a muscle’s “motor point” and a reference electrode off muscle. Although most reduced CMAPs recorded in patients with hand problems are caused by AL or demyelination related to focal mononeuropathies, it is important to recall that the motor unit reflected in CMAP tests more structures than just nerves. Thus, reduced or absent CMAP may reflect disorders of LMNs, roots (compressive and infiltrative), plexus, peripheral nerves, NMJ, muscle membranes, and muscles. Second, reinnervation after motor AL underlies increasing CMAP amplitudes and areas (but different wave shapes) by two distinct mechanisms: collateral sprouting and regeneration. Since sensory axons do not branch, sensory reinnervation proceeds by the sole mechanism of regeneration—the distal advance of growth cones. By contrast, after partial AL motor axons in continuity with muscle undergo collateral sprouting by 3 to 6 months to reinnervate disconnected muscle fibers. In addition, cut axons may regenerate at similar rates as sensory axons to grow back into muscle, provided they are not blocked or diverted. Muscle NMJs accept connections with ingrowing regenerating motor axons for a finite period, a year or possibly longer, depending on patient age and various strategies to prolong that receptivity. Because the CMAP (but not SNAP) is recorded from an end organ (muscle), and because there are two motor but only one sensory mechanism for reinnervation, a normal CMAP amplitude recorded in both the acute phase after nerve injury and chronic phase after reinnervation may misleadingly imply a normal number of motor axons. Further study with EMG would show reinnervation.
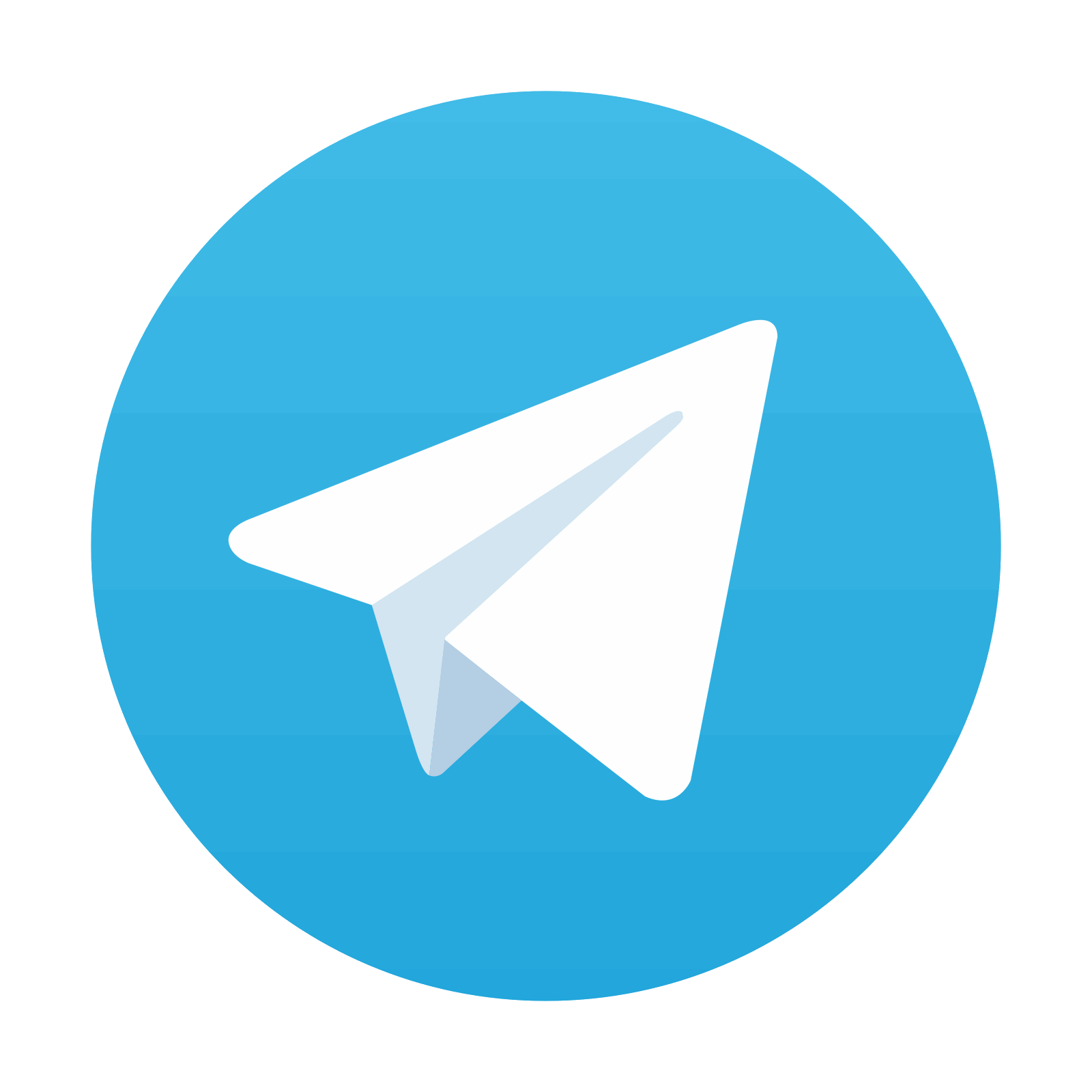
Stay updated, free articles. Join our Telegram channel

Full access? Get Clinical Tree
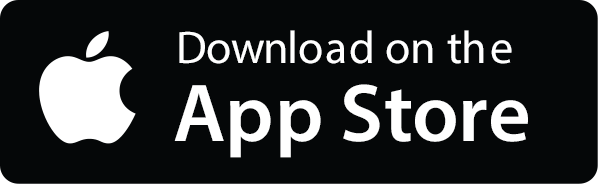
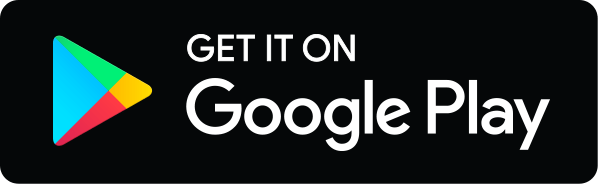