Radiation fibrosis syndrome describes the multiple neuromuscular, musculoskeletal, visceral, and other late effects that result from radiation-induced fibrosis. Radiation can damage the spinal cord, nerve roots, plexus, local peripheral nerves, and muscles within the radiation field. This constellation is known as a “myelo-radiculo-plexo-neuro-myopathy” and can result in pain, sensory loss, weakness, and other signs and symptoms. Although there is no curative treatment for radiation damage, supportive management of symptoms can be helpful in restoring and maintaining function and quality of life.
Key points
- •
Radiation fibrosis syndrome (RFS) describes the multiple neuromuscular, musculoskeletal, visceral, and other late effects that result from radiation-induced fibrosis.
- •
Radiation can damage the spinal cord, nerve roots, plexus, local peripheral nerves, and muscles within the radiation field. This phenomenon is known as a “myelo-radiculo-plexo-neuro-myopathy” and results in multiple clinical manifestations.
- •
There is no cure for RFS, but supportive treatment of its clinical sequelae can potentially result in improved function and quality of life.
Introduction
The American Cancer Society estimates that there are approximately 14.5 million cancer survivors in the United States as of 2015. Approximately one-half of patients treated for cancer will have required radiation therapy (RT) at some time during the course of their illness. Despite the therapeutic goals of RT as either potentially curative (ie, head and neck cancer [HNC]), or palliative (ie, bone metastases), toxicity is commonly seen. Such toxicity can manifest with treatment and remain (a long-term effect) or develop and progress weeks, months, or decades later (late effect). This article describes the common neuromusculoskeletal late effects likely to be seen as a result of RT and the rehabilitation principles key to evaluating and managing these complex disorders.
Introduction
The American Cancer Society estimates that there are approximately 14.5 million cancer survivors in the United States as of 2015. Approximately one-half of patients treated for cancer will have required radiation therapy (RT) at some time during the course of their illness. Despite the therapeutic goals of RT as either potentially curative (ie, head and neck cancer [HNC]), or palliative (ie, bone metastases), toxicity is commonly seen. Such toxicity can manifest with treatment and remain (a long-term effect) or develop and progress weeks, months, or decades later (late effect). This article describes the common neuromusculoskeletal late effects likely to be seen as a result of RT and the rehabilitation principles key to evaluating and managing these complex disorders.
Radiation therapy delivery
Understanding radiation injury requires a basic knowledge of what radiation is and how it is, and has historically been, delivered. The basic unit currently used in radiation oncology is the gray (Gy). One gray is defined as the absorption of 1 J of radiation per 1 kg of matter. Radiation dosing was previously expressed in absorbed radiation dose or rads (1 rad = 0.1 J/kg = 0.01 Gy = 1 cGy). Therefore, a total dose of radiation of 5000 rad is equivalent to 5000 cGy or 50 Gy.
In general, as total dose increases, so too does the risk of radiation injury. Table 1 lists the tolerances of select tissues to therapeutic radiation. The total dose of radiation delivered is not, however, the sole determinant of radiation injury. In addition to total dose, the size of each radiation fraction, the type of tissue radiated, the time from radiation treatment, individual patient tolerance, and concomitant oncologic treatments impact the development of RFS.
Site | TD 5/5 (Gy) a | TD 50/5 (Gy) b | Complication/End Point (s) | ||||
---|---|---|---|---|---|---|---|
Portion of Organ Irradiated | Portion of Organ Irradiated | ||||||
1/3 | 2/3 | 3/3 | 1/3 | 2/3 | 3/3 | ||
Neuromusculoskeletal: | |||||||
Brachial plexus | 62 | 61 | 60 | 77 | 76 | 75 | Clinical nerve damage |
Brain | 60 | 50 | 45 | 75 | 65 | 60 | Necrosis, infarct |
Brainstem | 60 | 53 | 50 | — | — | 65 | Necrosis, infarct |
Cauda equina | No volume effect | 60 | No volume effect | 75 | Clinical nerve damage | ||
Optic nerve | No partial volume | 50 | No partial volume | 65 | Blindness | ||
Spinal cord | 50 (5 cm) | 50 (10 cm) | 47 (20 cm) | 70 (5 cm) | 70 (10 cm) | — | Myelitis, necrosis |
Temporomandibular joint | 65 | 60 | 60 | 77 | 72 | 72 | Marked limitation in joint function |
Femoral head | — | — | 52 | — | — | 65 | Necrosis |
Visceral: | |||||||
Bladder | N/A | 80 | 65 | N/A | 85 | 80 | Contracture, volume loss |
Colon | 55 | — | 45 | 65 | — | 55 | Obstruction, perforation, fistula, ulceration |
Esophagus | 60 | 58 | 55 | 72 | 70 | 68 | Stricture, perforation |
Heart | 60 | 45 | 40 | 70 | 55 | 50 | Pericarditis |
Kidney | 50 | 30 | 23 | — | 40 | 28 | Nephritis |
Liver | 50 | 35 | 30 | 55 | 45 | 40 | Liver failure |
Lung | 45 | 30 | 17.5 | 65 | 40 | 24.5 | Radiation pneumonitis |
Rectum | — | — | 60 | — | — | 80 | Severe proctitis, necrosis, fistula |
Small intestine | 50 | — | 40 | 60 | — | 55 | Obstruction, perforation, fistula |
Stomach | 60 | 55 | 50 | 70 | 67 | 65 | Ulceration, perforation |
a TD 5/5 is the average dose that results in a 5% complication risk within 5 years.
b TD 50/5 is the average dose that results in a 50% complication risk within 5 years.
The practice of RT has evolved significantly over past decades in an effort to minimize toxicity. Before the 1980s, radiation was generally delivered in an anterior-posterior (AP) and posterior-anterior (PA) fashion. Radiographic imaging was used for treatment planning, patient positioning, and tumor identification. Simple blocks were used to shape the radiation beam. Because large amounts of normal tissue were included in the radiation field, the radiation dose could not safely exceed what was tolerated by the most sensitive structure (ie, the lung or bowel) in the field. In addition to considerable amounts of normal tissue being affected, surface hotspots, and inability to maximize radiation dose to the tumor limited the effectiveness of conventional RT for some tumors. Despite these limitations, conventional AP/PA RT is still used today, as it is relatively uncomplicated and inexpensive compared with newer techniques.
To circumvent the many barriers of conventional AP/PA RT, conformal radiation techniques were developed and continue to evolve in concert with imaging and computer technology. Three-dimensional (3D) conformal techniques use 3D imaging, such as computed tomography (CT) and MRI, to identify tumor and normal tissues. As opposed to conventional 2D techniques, which direct radiation beams from only 2 directions, 3D conformal techniques use multiple beams of radiation from varied directions to sculpt the radiation to the tumor while minimizing exposure to normal tissue. In addition to delivering radiation from multiple directions, it is possible to modulate the intensity of radiation coming from each direction for enhanced control. This technique is known as intensity-modulated radiotherapy (IMRT). IMRT is a frequently used radiotherapy technique. It is often used where tumor is adjacent to critical structures. For example, in nasopharyngeal cancer, IMRT has been demonstrated to improve local recurrence-free survival while minimizing the incidence of complications, such as xerostomia. Fig. 1 compares a 3D and IMRT treatment plan in a patient with HNC.
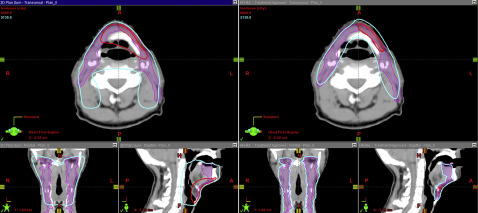
The ability to sculpt and modulate radiation with exacting tolerances has allowed the development of stereotactic radiosurgical (RS) techniques. RS is similar to IMRT in that radiation is delivered from numerous angles and focused precisely on the tumor with relative sparing of surrounding normal tissue. The primary difference is that stereotactic RS is delivered in fewer fractions; that is, 1 to 5 of higher dose. For instance, spine metastases that are considered radioresistant to conventional radiotherapy (30 Gy in 10 fractions) may be better controlled with stereotactic RS (24 Gy in 1 fraction). Fig. 2 depicts the isodose curve for radiosurgery of metastatic disease to L4 and L5.
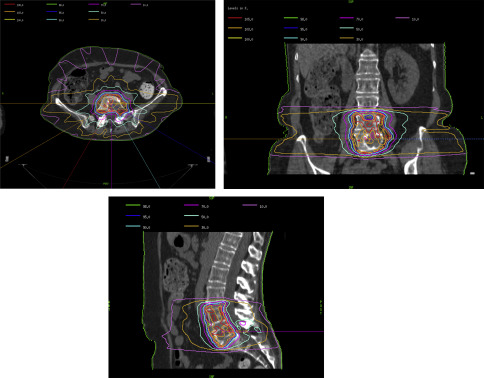
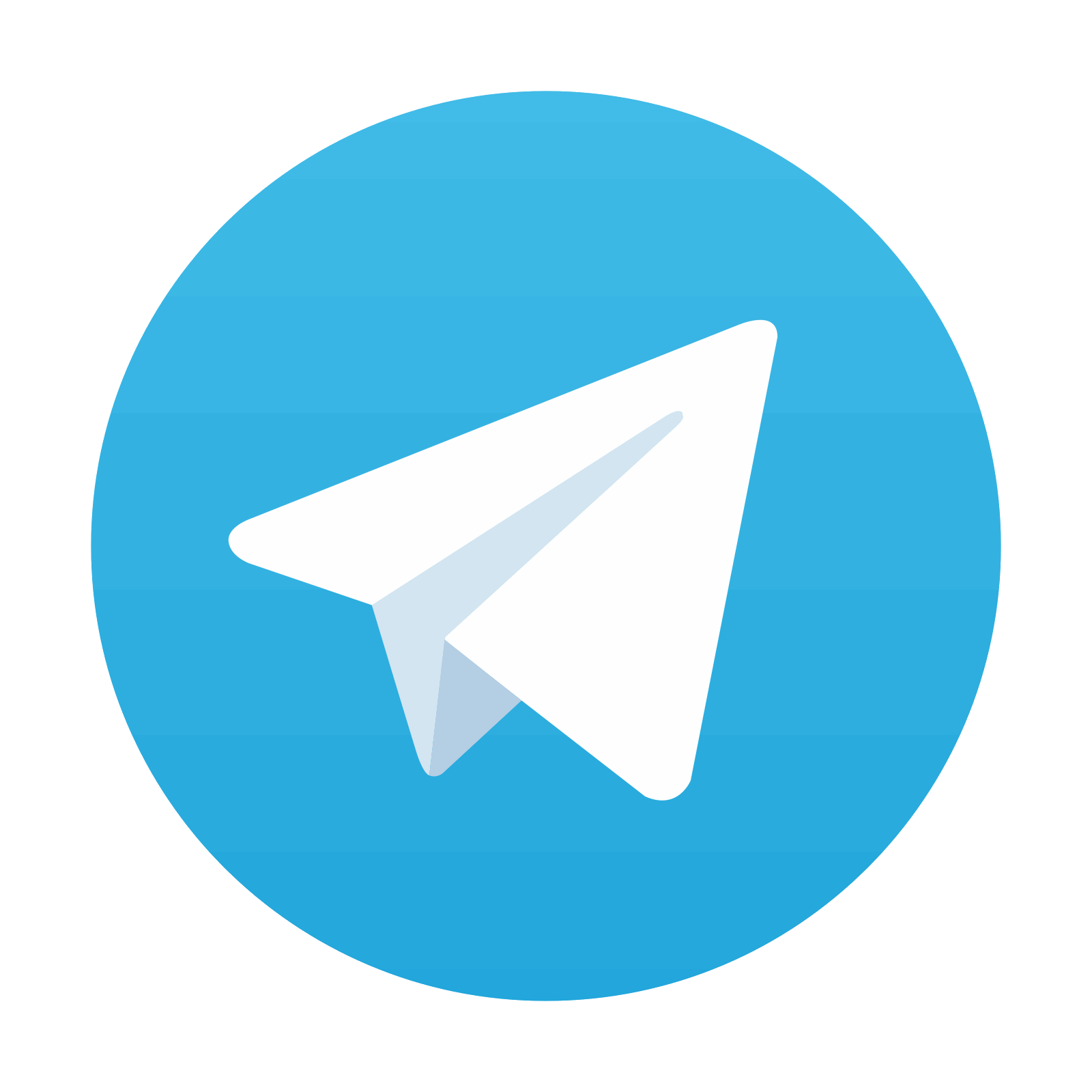
Stay updated, free articles. Join our Telegram channel

Full access? Get Clinical Tree
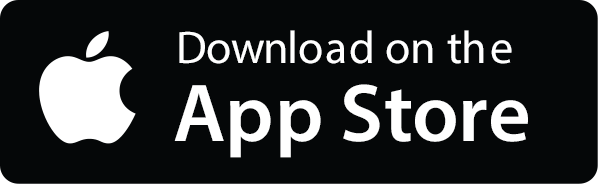
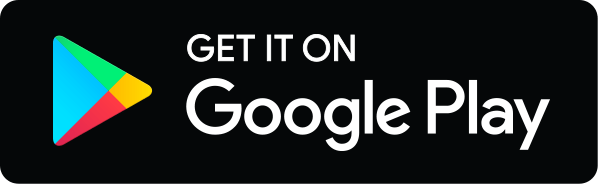
