Abstract
The average orthopaedic trainee about to sit the FRCS (Tr & Orth) exam requires a basic knowledge of genetics.
This doesn’t need to be encyclopaedic, but candidates will need to have a sound grasp of disease inheritance and genetic disorders. Trainees should be able to draw a family pedigree of single gene inheritance and know the gene mutations of the more common orthopaedic conditions.
By comparison, mention genetic viva questions to any examiner and you get a slightly puzzled look back. Safe to say, it is not a major A-list topic for the vivas and probably doesn’t even make the B-list. However, the subject does intermittently appear in the vivas and therefore it is definitely worthwhile knowing how the questions will run in order to uncomplicate a potentially complicated topic.
Introduction
The average orthopaedic trainee about to sit the FRCS (Tr & Orth) exam requires a basic knowledge of genetics.
This doesn’t need to be encyclopaedic, but candidates will need to have a sound grasp of disease inheritance and genetic disorders. Trainees should be able to draw a family pedigree of single gene inheritance and know the gene mutations of the more common orthopaedic conditions.
By comparison, mention genetic viva questions to any examiner and you get a slightly puzzled look back. Safe to say, it is not a major A-list topic for the vivas and probably doesn’t even make the B-list. However, the subject does intermittently appear in the vivas and therefore it is definitely worthwhile knowing how the questions will run in order to uncomplicate a potentially complicated topic.
Some genetic material will find its way into Section 1 SBA and EMI papers so common genetic terminology and definitions need to be reasonably well understood.
General information
Nucleic acid structure (Figure 27.1)
Figure 27.1 Nucleic acid structure.
There are two main types of nucleic acid, DNA (deoxyribonucleic acid) and RNA (ribonucleic acid), which each consist of a sugar-phosphate backbone with projecting nitrogenous bases. The nitrogenous bases are of two types, purines and pyrimidines.
In DNA, there are two purine bases, adenine (A) and guanine (G), and two pyrimidine bases, thymine (T) and cytosine (C) (Figure 27.2).
Figure 27.2 DNA structure.
RNA also contains adenine (A), guanine (G) and cytosine (C), but contains uracil (U) instead of thymine (T).
In DNA the sugar is deoxyribose, whereas in RNA it is ribose. The nitrogenous bases are attached to the 1′ (one prime) position of each sugar, and the phosphate links 3′ and 5′ hydroxyl groups.
Each unit of purine or pyrimidine base together with the attached sugar and phosphate group(s) is called a nucleotide.
A molecule of DNA is composed of two nucleotide chains that are coiled clockwise around one another to form a double helix with approximately 10 nucleotides per complete turn of DNA (Figure 27.2).
The two chains run in opposite directions (i.e. 5′ to 3′ for one and 3′ to 5′ for the other) and are held together by hydrogen bonds between A in one chain and T in the other or between C and G.
Pedigree charts
Pedigree charts can be complicated to understand if you are unfamiliar with the basics.
A standard set of symbols are used (Figure 27.14). The father is conventionally placed on the left, and all members of the same generation are placed on the same horizontal level. Roman numerals are used for each generation, starting with the earliest, and Arabic numerals are used to indicate each individual within a generation (numbering from the left).
Single gene disorders are due to mutations in one or both copies or alleles of an autosomal gene or to mutations in genes on the X or Y chromosome (sex-linked inheritance). These disorders show characteristic patterns of inheritance in family pedigrees.
Structured oral examination question 1
Autosomal dominant (AD) inheritance
COMMENT: This is much easier to explain if candidates are able to draw out a Punnett square. Just get the pen out and practise (Figure 27.3).
EXAMINER: What pattern of inheritance does the pedigree chart show and why (Figure 27.4)?
CANDIDATE: This is autosomal dominant because:
There are people with the disease in each generation.
Both males and females are affected in approximately equal numbers.
All forms of transmission are present (male to female, female to male, female to female and, in particular, male to male, which would not be present if the condition were X-linked).
At conception, each child has a 1 in 2 (50%) chance of inheriting the condition.
COMMENT: Unaffected persons do not transmit the condition if the condition is fully penetrant (e.g. in achondroplasia).
It is equally likely that a child will receive the mutant or normal allele from the affected parent.
On average there is a 1 in 2 or 50% chance that each child of a heterozygous parent will inherit the gene mutation.
There is usually a variation in time of onset and severity of condition with AD traits. This is likely to be due in part to the effects of other ‘modifier’ genes and also lifestyle/environmental factors.
EXAMINER: Choose an autosomal dominant condition and discuss the genetics.
COMMENT: A gift if you have done your homework. The three most obvious disorders to choose are (1) achondroplasia, (2) osteogenesis imperfecta or (3) neurofibromatosis. Plenty to talk about with each condition.
It is unwise to choose an obscure disorder that the examiner knows very little about. The conversation will dry up and the examiners will invariably switch back to more difficult basic science questions.1 Candidates are throwing away easy scoring opportunities.
The other situation is that the clinical condition to discuss has already been decided beforehand.
Achondroplasia
About 80% of people with achondroplasia have the condition as the result of a new mutation; the incidence of the condition increases with increasing paternal age.
Achondroplasia is inherited as an autosomal dominant condition. Each child of someone who has achondroplasia has a 1 in 2 (50%) chance of inheriting the condition.
If both parents have achondroplasia, and a child inherits a copy of the altered gene from both parents, the condition is severe and not compatible with life.
The gene for achondroplasia is on the short arm of human chromosome 4 at locus p16.3.
The condition is the result of a mutation in the gene that codes for fibroblast growth factor receptor 3 (FGFR3), which is a key component of cartilage development.
The main defect is abnormal endochondral bone formation in the cartilaginous proliferative zone of the physis.
COMMENT: Clinical features of achondroplasia may be discussed in more detail and these can easily be mugged up in a standard textbook:2 kyphosis, spinal stenosis, genu varum, trident hand, radial head subluxation, etc.
EXAMINER: What is the genetic mutation responsible for this condition, and what effect does this have?
CANDIDATE: Achondroplasia is caused by a single point mutation in the gene encoding the fibroblast growth factor receptor 3 (FGFR3). The FGFR3 is believed to regulate bone growth by limiting endochondral ossification. Two mutations in the FGFR3 gene are responsible for 99% of cases of achondroplasia. These mutations both cause substitution of a glycine amino acid by an arginine and lead to prolonged receptor activation after ligand binding and result in excessive growth limitation. Soluble FGFR3 has successfully been used in mice to act as a decoy for FGF in order to restore normal growth in achondroplasia.
EXAMINER: What is the mode of inheritance of this condition?
CANDIDATE: It is autosomal dominant.
EXAMINER: This child’s parents do not have this condition. How is this possible?
Osteogenesis imperfecta
There is a qualitative or quantitative defect of type 1 collagen synthesis. Originally classified by Silence3 into four subgroups, we now know there are many more different subgroups of OI.
The vast majority of cases are autosomal dominant.
Type 1 collagen is the major extracellular protein in bone, skin and tendon.
The structural unit of type 1 collagen is called tropocollagen and is a heterotrimer composed of three polypeptide chains. Two chains are pro-α1(I) and the third chain is pro-α2(I). The three chains form a distinctive unit in which the polypeptide chains wrap around each other for most of their length, forming a tight triple helical braid.
The triple helical structure is not the same as the pro-α helix that is formed by a single polypeptide chain, which is the defining feature of all collagen.
The collagen triple helix forms because both the α1 and α2 chains contain repeat sequences of amino acids (–Gly–X–Y), where Gly is glycine, X is proline and Y is usually hydroxyproline. This arrangement results in a constrained structure that imparts a tight kink in the polypeptide chain with the glycine chain preventing steric hindrance that would otherwise impair wrapping of the helical band.
Only Gly, which has no side chain, can pack into the centre of the triple-helix structure without distortion. A missense mutation4 leading to the replacement of even one Gly in the repeating (Gly–X–Y)n sequence by a larger residue may lead to a pathological condition.
The pro-α1(I) chain is encoded from the COL1A1 gene on chromosome 17 and the pro-α2(I) residue is encoded by the COL1A2 gene on chromosome 7.
Mutations in the COL1A1 gene on chromosome 17 or COL1A2 gene on chromosome 7 are responsible for OI.
In the type 1 condition the COL1A1 mutant allele results in failure of the production of the pro-α1(I) chain due to a premature codon stop. There is only 50% production of normal pro-α1(I) chain.
In more severe forms of the condition there are mutations of either the COL1A1 gene or the COL1A2 gene resulting in a mixture of abnormal and normal collagen chains.
Structured oral examination question 2
Autosomal recessive (AR) inheritance
EXAMINER: Can you please draw a Punnett square demonstrating an autosomal recessive trait (Figure 27.5).
CANDIDATE: Recessive means two copies of the gene are necessary to have the trait/disease. Parents of affected children are both carriers of the condition.
Typically, heterozygotes for the trait/disease are not affected but are gene carriers. Most people do not know they carry a recessive gene for a condition until they have a child with the disease.
If the parents are both carriers the risk of them having an affected child is 25% (1/4) and the risk of them having a child who is a carrier is 50%.
Unaffected adult offspring of carrier parents have a 2/3 risk of carrier status.
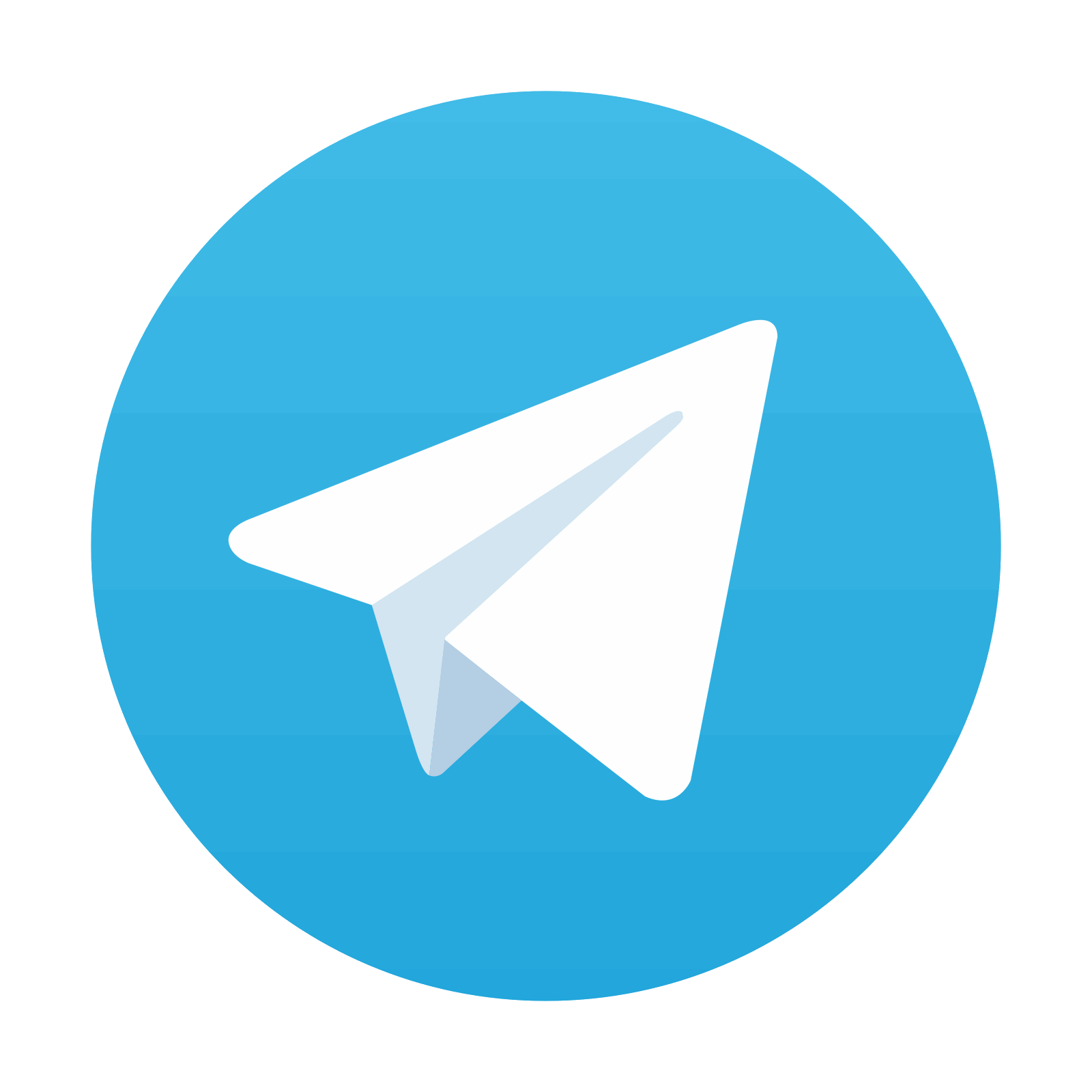
Stay updated, free articles. Join our Telegram channel

Full access? Get Clinical Tree
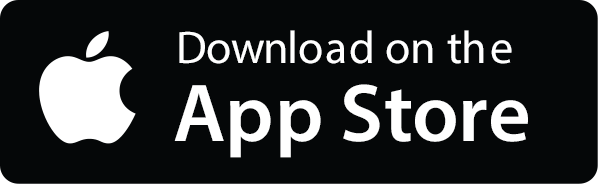
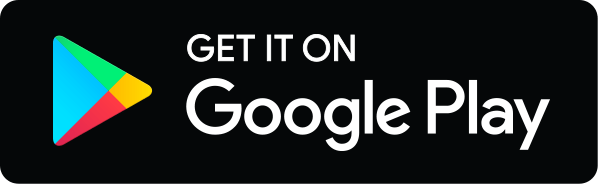
