Abstract
Section 2 of the basic science (Tr & Orth) syllabus is a large topic, difficult to grasp at face value as it appears quite removed from the average orthopaedic surgeon’s practice. However, it pervades many aspects of clinical practice and therefore must be understood.
It contains large sections of A-list topics that just need to be learnt as well as possible, otherwise marks will be thrown away.
Introduction
Section 2 of the basic science (Tr & Orth) syllabus is a large topic, difficult to grasp at face value as it appears quite removed from the average orthopaedic surgeon’s practice. However, it pervades many aspects of clinical practice and therefore must be understood.
It contains large sections of A-list topics that just need to be learnt as well as possible, otherwise marks will be thrown away.
Candidates may be asked very general questions or questions in more detail, so you need to cover both bases. Esoteric or un-Googleable questions are also fairly common in this section.
Candidates will need to double time on this section to both understand the topics and then work through viva practice sessions to home in on the target answers.
This section was generally well received in the first edition viva book and we have kept and updated the majority of previous viva questions. As such, in certain sections there is a rough marking scheme, so you can judge your level.
1. Bone structure and function
This is an A-list topic. The topic is large with a number of subtopics within the main topic that the examiners can easily focus in on.
Structured oral examination question 1
EXAMINER: What is bone?
CANDIDATE: Bone is a dynamic composite form of specialized connective tissue composed of cells (10%) and matrix (90%). The matrix has inorganic (60%) and organic (40%) components …
COMMENT: The viva could start off awkwardly with a definition that may catch the unsuspecting candidate off-guard. Go for an uncomplicated, non-controversial answer that allows you to continue talking if you feel confidently able to do so.
Or
CANDIDATE: Bone is an organ.
CANDIDATE: [Pause] I am not sure.
COMMENT: Make sure you know the definition of an organ.1 Try to avoid giving an answer that will lead you up a blind alley.
An organ is composed of multiple tissue types. For bone these include:
Bone tissue (a.k.a. osseous tissue).
Fibrous connective tissue.
Cartilage.
Vascular tissue.
Lymphatic tissue.
Adipose tissue.
Nervous tissue.
CANDIDATE: The three main functions of bone are:
1. As a reservoir for calcium.
2. As a source of haematopoietic cells such as erythrocytes, leucocytes and platelets.
3. A mechanical role in supporting the body’s tissues, providing attachment for muscles and protecting internal organs.
COMMENT: There are other minor functions, but again, keep it simple.
CANDIDATE: There are two main macroscopic types of bone, either (1) lamellar or (2) woven. The structure of lamellar bone can be either cortical compact or cancellous trabecular bone. Woven bone can be either immature (fracture callus) or pathological.
COMMENT: Candidates may be pressed in a bit more detail about the differences between woven and lamellar bone, especially at the beginning of a viva, because it is basic information candidates would be expected to know.
Woven bone
Has a random arrangement of collagen, there are no lamellae, it is weaker and more flexible than lamellar bone.
More cellular (×8 lamellar bone).
More metabolically active with increased turnover.
Variable irregular mineral content.
It is found in the embryonic skeleton and the metaphyseal region of growing bones. It is also present in early hard callus and found in pathological bone such as tumours, osteogenesis imperfecta and pagetic bone.
It is found where bone needs to be laid down rapidly.
Lamellar bone
This is mature adult bone that is subdivided into cortical or cancellous bone.
Osteoblasts lay down collagen matrix in microscopic thin, layered sheets called lamellae.
Collagen fibres are orientated more parallel to each other.
Low number of cells.
Slow deposition/turnover.
Stronger and less flexible than woven bone.
Anisotropic.
Table 20.1 Lamellar bone versus woven bone.
Property | Woven bone | Lamellar bone |
---|---|---|
Definition | ‘Primitive’, immature | ‘Mature’ bone, remodelled woven bone |
Found in | Immature (embryonic/neonatal skeleton, metaphyseal region fracture healing) | Cortical compact |
Pathological bone (pagetic bone, tumour) | Cancellous trabecular | |
Composition | Dense collagen fibres, varied mineral content, greater turnover, more cells per unit volume | Formed by intramembranous or endochondral ossification, contains collagen fibres |
No lamellae | ||
Organization | Randomly arranged collagen fibres, disorganized | Highly ordered, stress-orientated collagen fibres |
Response to stress | Isotropic: independent of direction of applied forces | Anisotropic: mechanical behaviour differs according to direction of applied force |
Bone’s greatest strength is parallel to its longitudinal axis | ||
Biomechanics | Weaker, more flexible, more easily deformed | More stiffness and strength |
High-yield orthopaedics: Differentiating features of woven vs. lamellar bone.
No lamellae, isotropic.
EXAMINER: What is the difference between cortical and cancellous bone (Table 20.2)?
CANDIDATE: Cortical bone is compact with a high matrix mass per unit volume, low porosity and is subjected to bending, torsional and compressive forces. It is usually found in the diaphysis of long bones. The basic structure is the osteon or Haversian system.
Cancellous bone is found in the metaphysis or epiphysis of long bones. It has an architecture of 3D lattice rods and plates, high porosity with large spaces between trabeculae and predominantly subjected to compressive loads.
CANDIDATE: Isotropic refers to uniform properties in all directions, independent of the direction of load application.
EXAMINER: So, which type of bone is isotropic?
CANDIDATE: Woven.
EXAMINER: So, what about lamellar bone. Is this isotropic?
CANDIDATE: No.
EXAMINER: Why?
CANDIDATE: I am not sure.
COMMENT: These are fairly straightforward questions but may catch the unprepared candidate out. Lamellar bone has stress-orientated collagen fibres and has anisotropic features. The mechanical behaviour differs according to the direction of applied force: the bone’s greatest strength is parallel to the longitudinal axis.
EXAMINER: What is the structure of bone?
CANDIDATE: The main structural unit within cortical bone is the Haversian system (osteon) with its central neurovascular channels enclosed within concentric lamellae.
Lying in between intact osteons separated by cement lines are incomplete lamellae called interstitial lamellae. These fill the gaps between osteons and are remnants of bone remodelling.
Neither collagen nor canaliculi cross cement lines, forming areas of relative weakness.
Each osteon or Haversian system consists of five to seven concentric layers (lamellae) of bone matrix.
Volkmann’s canals run perpendicular to the long bone axis carrying blood vessels to and from the Haversian systems to the outer surfaces of the bone.
COMMENT: The viva may just begin by candidates being shown a diagram of the bone Haversian system and being asked to talk through the diagram. Less likely but still possible is for candidates to be asked to draw out the bone Haversian system.
CANDIDATE: Bone compromises cells (10%) and extracellular matrix (90%).
The cells include osteoblasts, osteocytes, osteoclasts and bone lining cells.
The matrix has organic (collagens, mainly type 1) and inorganic (calcium phosphate, osteocalcium phosphate) constituents.
CANDIDATE: The main types of bones cells, and their function, are as follows:
1. Osteoblast: bone-forming cells.
2. Osteoclast: bone-resorbing cells, multinuclear irregular giant cells.
3. Osteocyte: maintains bone, important for calcium and phosphate homeostasis.
4. Osteoprogenitor cell: precursors to osteoblasts that line the Haversian system and can be stimulated to differentiate into osteoblasts and form new bone.
5. Bone lining cells: inactive osteoblasts.
COMMENT: Some textbooks mention only three or four types of bone cells, usually omitting osteoprogenitor cells and sometimes bone lining cells.
The osteoprogenitor cells originate from mesenchymal stem cells and line the Haversian canals, endosteum and periosteum. They can quickly differentiate into osteoblasts if needed.
EXAMINER: How do osteoblasts and osteoclasts differ?
CANDIDATE: Osteoblasts are derived from undifferentiated mesenchymal cells; they are bone-forming and lay down osteoid (type 1 collagen) as well as activating osteoclasts to resorb bone via the receptor activator of nuclear factor κβ (RANK) and its ligand (RANKL) system. RANKL is expressed by macrophages and osteoblasts, and functions as an activator of RANK. RANK, expressed on osteoclast precursors, is a key regulator of osteoclastogenesis.
These processes are controlled by cytokines, growth factors and bone morphogenic protein (BMP).
Osteoclasts are derived from a haemopoietic monocyte cell lineage. They are multinucleated giant cells that resorb bone and are characterized by a cytoplasm that has a homogeneous foamy appearance due to a high concentration of vesicles and vacuoles. These vacuoles include lysosomes filled with acid phosphatase.
They can sit in small pits called Howship’s lacunae, on the bone surface, or lead cutting cones that tunnel through the bone. Under their ruffled brush border, with an increased surface area, they create a low pH microenvironment that dissolves inorganic apatite crystals. Enzymes are released (tartrate resistance acid phosphatase, TRAP) and proteases then break down the organic matrix components. This process is controlled via the RANKL system (inhibited by osteoprotegerin) of activated osteoblasts.
Osteocytes are osteoblasts that have become trapped in bone matrix (comprising up to 90% of the cells in bone). They have an important role in the homoeostasis of calcium and phosphate metabolism.
CANDIDATE: Wolff’s law states that bone will adapt to the loads placed through or across it. It is the result of the close coupling within bone remodelling units consisting of osteoblasts, osteoclasts and supporting stromal tissues. If loading on a particular bone increases, the bone will remodel itself over time to become stronger to resist that sort of loading.
COMMENT: Bone models and remodels in response to the mechanical stresses it experiences, resulting in a minimal-weight structure that is adapted to its applied stresses.
EXAMINER: Can you give me an example of Wolff’s law?
CANDIDATE: The racket-holding arms of tennis players are stronger than the other arm. There is thicker cortical bone alongside hypertrophy of the muscle attachment sites. The arm is about a third bigger in size (35%).
The femoral neck width of obese people changes to accommodate the added weight. In this case the width of the femoral neck has increased to dissipate weight throughout the bony area by increasing surface area and strength through redistribution of bone.
The reverse is true: if loading on a bone decreases, the bone will become less dense and weaker due to the lack of stimulus required for continued remodelling.
CANDIDATE: Remodelling occurs in small packets of cells known as basic multicellular units (BMUs). Compressive forces inhibit longitudinal growth; tension stimulates it. This law suggests mechanical factors influence longitudinal growth, bone remodelling and fracture repair. The underlying mechanisms remain unclear.
CANDIDATE: Two transcription factors are important for osteoblastic differentiation.
1. Osterix (Osx) is an osteoblast-specific transcription factor essential for osteoblast differentiation and bone formation.
2. Runx2 is a multifunctional transcription factor that induces differentiation of multipotent mesenchymal cells into immature osteoblasts.
CANDIDATE: Transcription factors are proteins involved in the process of converting, or transcribing, DNA into RNA. Transcription factors include a wide number of proteins, excluding RNA polymerase, that initiate and regulate the transcription of genes.
COMMENT: A picture was shown of an osteoclast in Howship’s lacuna (Figure 20.1).
Figure 20.1 Activated osteoclast. The osteoclast plasma membrane is divided into multiple domains. At the ruffled border, the osteoclast secretes acid and lysosomal enzymes that digest the mineral and protein components of the underlying bone. The degradation products of collagen and other matrix components are endocytosed, transported through the cell and exocytosed through a functional secretory domain.
CANDIDATE: This is an activated osteoclast.
EXAMINER: How do osteoclasts resorb bone?
CANDIDATE: Osteoclasts resorb bone by binding to the bone surface using integrin anchor proteins and secreting hydrogen ions into the sealed area produced with a carbonic anhydrase system allowing dissolution of hydroxyapatite mineral matrix. They also secrete proteolytic lysosomal enzymes that hydrolyze the organic cellular components. The ruffled border greatly increases the surface area osteoclasts.
EXAMINER: What do you understand by the term remodelling? Describe the process.
CANDIDATE: Remodelling is the process whereby the structure of bone is transformed from disorganized, haphazard immature bone to organized lamellar bone by osteoclast cutting cones. The osteoclasts dissolve the inorganic matrix by acid secretion and as they move forwards the resorption cavity is occupied by osteoblasts that lay down osteoid before it is calcified.
COMMENT: Learn to draw a cutting cone and be able to describe how this functions to remodel bone as you go along (Figures 20.2 and 20.3).
Figure 20.2 Osteoclastic cutting cone. Candidate drawing. The cutting filling cone has a head of osteoclasts that cut through the bone, and a tail of osteoblasts that form a new secondary osteon.
Figure 20.3 Osteoclastic cutting cone. At its tip, osteoclastic resorption takes place while in the latter parts of the cone osteoblasts deposit osteoid with subsequent mineralization. Reversal refers to a 1- to 2-week interval between completion of resorption and initiation of bone matrix formation. The structure terminates as a closing zone in which osteoblasts close the newly excavated osteon by adding centripetal layers of lamellar bone inward from the cement line boundary.
CANDIDATE: The blood supply to bone is derived from three sources.
(a) High-pressure nutrient artery system. The nutrient arteries are branches of the systemic circulation and enter the bony mid-diaphysis through the nutrient foramen passing to the medullary canal before branching into ascending and descending vessels and arteriolar branches supplying the inner two-thirds of the diaphyseal cortex (endosteal circulation).
Remember that the end arterioles run in the Volkmann canals which drain into the Haversian system and finally drain back into the central venous sinus and out via the nutrient vein.
(b) The low-pressure periosteal system circulation. This supplies the outer third of the bone cortex and consists of an extensive network of capillaries covering the length of the diaphysis. The normal direction is centrifugal; however, following endosteal damage this system is reversed to centripetal. This is the dominant system in children, which allows their circumferential growth.
(c) Metaphyseal–epiphyseal system is the periarticular vascular complex that penetrates the cortex and supplies the metaphysis, physis and epiphysis with end arterioles.
EXAMINER: What is the direction of blood flow within a long bone?
CANDIDATE: Arterial flow in mature bone is centrifugal (inside to outside), which is the net effect of the high-pressure nutrient artery system and the low-pressure periosteal system.
When a fracture disrupts the nutrient artery system, the periosteal system pressure predominates, and blood flow is centripetal (outside to inside).
Flow in immature, developing bone is centripetal because the highly vascularized periosteal system is the predominant component.
CANDIDATE: The periosteum consists of an outer layer of fibroblasts and an inner layer of osteoblasts. The outer fibrous layer is structural, less cellular and continuous with the joint capsule, while the inner cambial layer is vascular, osteogenic and contributes to bone growth and fracture healing. With age, the periosteum thins and has less osteogenic capability.
EXAMINER: What are the functions of the periosteum?
CANDIDATE: Functions of the periosteum include:
Medium through which muscles, tendons and ligaments are attached.
Forms a nutritive function.
Can form bone when required.
Forms a limiting membrane that prevents bone tissue from ‘spilling out’ into neighbouring tissues.
CANDIDATE: Collagen is type 1 in bone. The structural unit of type 1 collagen is called tropocollagen and is a trimer composed of three polypeptide chains. Two chains are α1 chains and the third chain is α2. The three chains form a distinctive unit in which the polypeptide chains wrap around each other for most of their length, forming a tight triple helical braid.
Each polypeptide chain is a left-handed helix, but the triple helix is a right-handed superhelix (i.e. the opposite way around) (Figure 20.4).
The triple helical structure is not the same as the α helix that is formed by a single polypeptide chain and is the defining feature of all collagen. Collagen is secreted as an oversized molecule, procollagen, with specialized enzymes removing the N and C terminal propeptides leaving a triple helix with short non-triple helical stubs at the amino and carboxyl terminal chain ends. These non-helical regions are denoted telopeptides and they play a role in the registering of collagen α chains and cross-linking.
It is a fibril-forming collagen.
Figure 20.4 Several tropocollagen molecules are aggregated in an organized head-to-tail fashion into a structure called a collagen fibril. These collagen fibrils can be seen with an electron microscope and exhibit a 67-nm D-period banded appearance due to staggered gaps between the heads and tails of the molecules in each row.
EXAMINER: How is collagen assembled?
CANDIDATE: Collagen biosynthesis and assembly is a complex process that involves several steps. Intracellular events include post-translational hydroxylation of proline and lysine and subsequent glycosylation. Procollagen is secreted out of the cell. Extracellular events include terminal peptide cleavage and cross-linkage and self-assembly of collagen fibrils.
CANDIDATE: Osteoclastogenesis refers to the process of osteoclast differentiation and function. It is regulated by receptor activator of nuclear factor κB (RANK), RANK ligand (RANKL) and osteoprotegerin (OPG). RANK is expressed on the surface of osteoclast precursors and mature osteoclasts.
Osteoblasts secrete receptor activator of nuclear factor κβ ligand (RANKL) and macrophage colony-stimulating factor (M-CSF) to activate osteoclasts.
RANKL is a potent inducer of osteoclast formation.
With RANK activation, specific genes are switched on and the osteoclast becomes programmed to resorb bone. OPG acts as a decoy receptor, blocking RANKL binding and subsequent activation of the RANK system, thus inhibiting osteoclast differentiation and bone resorption.
CANDIDATE: Osteotropic factors include1,25-dihydroxyvitamin D3, parathyroid hormone, prostaglandin E2, and interleukin 11.
They induce the formation of osteoclasts by upregulating RANKL expression on the surface of marrow stromal cells and immature osteoblasts (see Figure 20.5).
Figure 20.5 Osteoblast/osteoclast coupling.
CANDIDATE: An osteoclastic cutting cone is a mechanism to remodel cortical bone by osteoclastic tunnelling (cutting cones). Osteoclasts at the front of the cutting cone remove bone and are followed by layering of osteoblasts and successive deposition of layers of lamellae after the cement line has been laid down.
The tunnel size narrows to a Haversian canal.
The head of the cutting cone is made up of osteoclasts (which bore holes through hard cortical bone).
Behind the osteoclast front are capillaries followed by osteoblasts (which lay down osteoid to fill the resorption cavity).
EXAMINER: Can you draw a cutting cone out?
COMMENT: [Figure 20.3] Remember to keep the diagram simple.
COMMENT: Again, keep the diagram simple. Use the orthopaedic 20-second diagram. Candidates should discuss the structure of bone as they draw paying particular attention to Haversian systems (Figure 20.6).
Figure 20.6 Structure of bone. Candidate drawing.
EXAMINER: What is bone composed of?
CANDIDATE: Bone consists of cells (10%) and extracellular matrix (90%). The extracellular matrix has organic (40%) and inorganic (60%) components.
Organic (40%).
Collagen (type I) 90%.
Proteoglycans.
Matrix proteins (non-collagenous).
▪ Osteocalcin, osteonectin, osteopontin.
Growth factors and cytokines.
Inorganic (60%).
Primarily hydroxyapatite Ca5(PO4)3(OH)2.
CANDIDATE: Type 1 [Remember – BONE].
EXAMINER: Draw me some collagen.
CANDIDATE:
COMMENT: Figure 20.7(a) focuses on the triple helix. Figure 20.7(b) is more complicated, but allows candidates more opportunity to focus on the hierarchical collagen arrangement.
Figure 20. 7 (a) Candidate drawing. Structure of collagen. (b) More complex drawing of collagen assembly.
EXAMINER: What do you know about the collagen structure in osteogenesis imperfecta (OI)?
CANDIDATE: Collagen is type 1 in bone. The structural unit of type 1 collagen is called tropocollagen and is a trimer composed of three polypeptide chains. Two chains are α1 chains and the third chain is α2. The three chains form a distinctive unit in which the polypeptide chains wrap around each other for most of their length, forming a tight triple helical braid.
The collagen triple helix forms because both the α1 and α2 chains contain repeat sequences of amino acids (-gly-X-Y), where gly is glycine, X is proline and Y is usually hydroxyproline.
This arrangement results in a constrained structure that imparts a tight kink in the polypeptide chain with the glycine chain preventing steric hindrance that would otherwise impair wrapping of the helical band.
With OI the majority of identified mutations are single nucleotide substitutions that result in alteration of glycine codons within the triple helical domain of either of the chains of type I procollagen (Figure 20.8). These substitutions for glycine within the triple helix are severely destabilizing and interrupt the triple helix. One-fifth of glycine substitutions in α2(I) are lethal, whereas nearly one-third of all glycine substitutions in α1(I) are lethal.
Figure 20.8 Collagen structure in osteogenesis imperfecta. Triple helix steric hindrance.
EXAMINER: What is the gene coding for OI?
CANDIDATE: COL1A1 and COL1A2 are the genes that encode the two chains pro α1(I) and pro α2(I), respectively, of type I procollagen.
EXAMINER: What about qualitative versus quantitative collagen deficiencies in osteogenesis imperfecta (OI)?
CANDIDATE: OI is a group of disorders with broad variations in clinical severity. Inheritance can be autosomal dominant or recessive. Quantitative defects are often heterozygous, with one copy not producing any collagen. Qualitative defects are usually errors in substitution or deletion leading to abnormal, less effectual collagen.
CANDIDATE: An eccentrically loaded bone has a compression and tension side. When bending occurs, one side of the bone is in tension, the other side is in compression. Bone resists compression better than tension and the side with tension will fracture first. Whenever feasible, any internal or external fixation device should be applied to the tension side to provide maximum stability.
EXAMINER: Draw me a longitudinal cross section of a long bone and tell me the areas (Figure 20.9).
Figure 20.9 Drawing of a typical long bone.
CANDIDATE: Osteoblasts are responsible for laying down new bone and are found in the growing portions of bone such as the periosteum and endosteum.
Osteocytes occupy lacunae that are contained within the calcified matrix of bone between lamellae. Osteocytes are derived from osteoblasts and are essentially osteoblasts surrounded by the products they secrete (Figure 20.10).
CANDIDATE: Skeletal dysplasias are a large group of rare, complex, heterogeneous disorders that involve cartilage and bone. Rubin’s classification of bone dysplasia is based on the type of abnormality (hypo-/hyperplasia) and the site involved in the bone: (1) epiphyseal, (2) physeal, (3) metaphyseal, (4) diaphyseal location.
Table 20.2 Cortical bone versus cancellous bone.
Property | Cortical bone | Cancellous (trabecular bone) |
---|---|---|
Description | Dense or compact bone | Spongy or cancellous bone |
Location | Diaphysis (outer layer of long bone) | Metaphysis or epiphysis (long bone) |
Architecture | Basic structure is the osteon | 3D lattice rods and plates |
Rods (thin trabeculae), plates (thick trabeculae) | ||
Loose network of struts | ||
Porosity | Low porosity (10%) | High porosity (50–90%) |
Large spaces between trabeculae | ||
Compactness | Softer, more elastic | |
Higher Young’s modulus of elasticity | ||
Mechanical stress | Subjected to bending, torsional and compressive forces | Predominantly subjected to compressive forces |
High matrix mass per unit volume | Reduced matrix mass per unit volume |
High-yield orthopaedics: cancellous bone.
No osteons – has a 3D lattice of rods and plates high porosity.
Structured oral examination question 2
EXAMINER: Draw me the structure of cortical bone (Figure 20.11a and 20.11b).
COMMENT: The cross-section of a long bone was quickly drawn, but we then almost immediately moved on to discussing the structure of compact bone.
EXAMINER: What runs in a Haversian canal?
CANDIDATE: Haversian canals contain blood vessels, lymphatics and nerves and are enclosed by closely packed concentric lamellae of bone. These canals branch into large transverse Volkmann canals that provide circulation to the cortical bone.
EXAMINER: What is the Haversian system?
CANDIDATE: The Haversian system, or osteon, is the basic structural unit of cortical bone and lies parallel to the long axis of the bone.
The osteon consists of a central Haversian canal that transmits a neurovascular bundle; surrounding this canal are at least five concentric lamellae of collagen. Within each lamella, collagen fibres lie in parallel but perpendicular to those fibres of adjacent lamellae. Volkmann’s canals run transversely to the bone’s long axis and permit communication between the outer vessels of the periosteum and the Haversian canals. Cement lines separate osteons.
CANDIDATE: Osteocytes are trapped osteoblasts located within lacunae between lamellae, communicating with adjacent osteocytes via cytoplasmic processes that travel through canaliculi.
CANDIDATE: Canaliculi are the spaces or ‘canals’ occupied by osteocyte cell processes. They connect the lacunae together within the cortical bone. Canaliculi are thought to modulate the response of bone to mechanical stimuli.
COMMENT: Simple basic questions on the structure of bone, but in the viva situation they usually appear much more than straightforward.
CANDIDATE: Bisphosphonates are a class of anti-resorptive agents used to treat diseases characterized by osteoclast-mediated bone resorption.
There are two classes of bisphosphonates.
1. Nitrogen-containing bisphosphonates (etodronate).
2. Non-nitrogen-containing (alendrolate, zoledronate, risedronate).
They act differently to diminish bone resorption.
Non-nitrogen-containing BPNs are metabolized into non-functioning ATP analogues, which cause eventual osteoclast apoptosis.
Nitrogen-containing BPNs act by inhibiting farnesyl pyrophosphate synthase (FPPS), resulting in decreased prenylation of small GTPases. These small GTPases are signalling proteins that regulate a number of cell processes such as membrane ruffling, cytoskeletal organization and trafficking of vesicles, which are required for osteoclast function.
EXAMINER: Can you tell me some clinical uses of bisphosphonates?
CANDIDATE: Clinical uses would include osteoporosis, hypercalcaemia of malignancy, Paget’s disease, solid tumours and metastatic bone disease, AVN and stress fracture. With children they can be used in fibrous dysplasia, osteogenesis imperfecta and Perthes’ disease.
Structured oral examination question 3
Bone healing, primary vs. secondary. Including cellular signalling pathways. Draw an osteonal cutting cone.
EXAMINER: What do we mean by primary bone healing?
CANDIDATE: Primary bone healing is a direct attempt by the cortex to re-establish the Haversian structure after a fracture but without fracture callus formation. Primary bone healing is led by osteonal cutting cones that consist of osteoclasts at the front of the cone that ream out a tunnel in the bone into which a blood vessel grows. This is followed by trailing osteoblasts that lay down new bone across the gaps to form a secondary osteon.
Primary bone healing occurs only under low interfragmentary movement (rigid fixation). There is direct osteonal remodelling and healing without external callous formation.
Primary bone healing can be further divided into gap and contact healing.
EXAMINER: What is contact healing?
CANDIDATE: Contact healing occurs if bone fragments have direct appositional contact and the gap between bone ends is less than 0.01 mm with an interfragmentary strain of less than 2%.
Osteons are able to grow parallel to the long axis of the bone by tunnelling osteoclastic activity.
Osteons traverse the fracture line.
CANDIDATE: Gap healing occurs when a small stable fracture gap less than 1 mm is present between bony fragments.
The fracture site is initially filled with transverse lamellar bone without intermediate fibrous or cartilage precursors. The bone is initially deposited perpendicular to the long axis of the bone prior to later osteonal remodelling along the lines of functional stress.
COMMENT: Contact healing – essentially no or minimal fracture gap. Direct osteonal remodelling. Absolute stability.
Gap healing – small stable fracture gap.
CANDIDATE: Secondary bone healing involves the formation of fracture callus. There is:
1. Haematoma formation with devascularization of the bone ends.
2. An inflammatory phase with local accumulation of macrophages, MSC, cytokines. Delivery of osteoprogenitor cells from the periosteal cambium layer and osteoclasts starting to remove necrotic bone. Coagulation and fibrin formation.
3. Primary soft callus formation. New blood vessels invade the haematoma, fibroblasts from the periosteum colonize the haematoma and produce collagen fibres. Granulation tissue gradually differentiates into fibrous tissue and afterwards fibrocartilage.
4. Callus mineralization (hard callus). Osteoblasts lay down woven bone at the periphery (intramembranous ossification) and fibrocartilaginous callus bridges the fracture site, chondroid matrix calcifies, new woven bone is laid down (endochondral ossification).
5. Remodelling. According to Wolff’s and Heuter–Volkmann’s laws: remodelling occurs with motion at the fracture site. Cartilage is found during the early stages of healing replaced by woven bone laid down by osteoblasts.
COMMENT: Primary and secondary bone healing comes up repeatedly in the viva exam and candidates need to be very clear about the distinction between them. Key points are as follows:
Primary bone healing
Requires anatomical reduction and interfragmentary compression.
Absolute stability.
This system will not tolerate strain.
Be clear about the differences between gap and contact healing.
Be able to describe and draw a cutting cone.
Secondary bone healing
Relative stability.
Endochondral and intramembranous ossification.
Fibrocartilage develops at the bone ends and this is subsequently calcified and replaced by woven bone or osteoid.
Haematoma, inflammation and cellular proliferation, soft callus (chondrogenic and osteogenic proliferation), hard callus and remodelling.
CANDIDATE: Perren introduced the importance of strain in fracture healing. Fracture gap strain is defined as the relative change in the fracture gap (ΔL) divided by the original fracture gap (L).
Tissue cannot be produced when strain conditions exceed the tissue strain tolerance. Cortical bone can only tolerate 2% strain. Rigid internal compression fixation, which minimizes strain, will lead to primary fracture healing. Lamellar bone can tolerate up to 10% strain, and when this relative stability is present, the fracture heals with callus or secondary fracture healing. Fracture healing will not occur when the strain at a fracture gap exceeds 10%. Comminuted fractures can tolerate more motion than simple fractures, because in a comminuted fracture the overall motion is shared among many fracture gaps.
CANDIDATE: [At the very end of the topic after discussing primary and secondary bone healing, Perren’s strain theory] There was a very well-written important paper published recently that discussed fracture healing. This paper mentioned Perren’s strain theory and introduced some exciting new ideas on fracture healing to challenge our traditional views on these complicated healing mechanisms.
EXAMINER: Which paper is that?
CANDIDATE: It was a paper published in the JBJS.2
EXAMINER: What did the paper say?
CANDIDATE: I haven’t had time to read it fully, so I am not too sure, but it’s a really well-written paper that everyone keeps mentioning and I am going to sit down and read it when I have more time.
COMMENT: The candidate should have kept quiet, they have ruined an otherwise good performance (score 7) and would probably be marked down to a 6. Candidates should refrain from mentioning a paper they haven’t fully read and struggle to say anything sensible about.3
Elliott et al. consider the whole fracture to be a ‘bone-healing organ’ that works as a functional unit and responds to biological and mechanical stimuli. They combined Wolff’s law, Perren’s strain theory, and Frost’s ‘mechanostat’ model to create their own model of bone homeostasis, healing and non-union (BHN conceptual model).
Using BHN, the behaviour of a ‘bone-healing organ’ was determined with respect to the mechanical strain applied to the organ. In BHN, the bone is in homeostasis when under tolerable strain (much less than 2%). For strains greater than 2% and less than 100%, a fracture occurs and is considered to be the beginning of the ‘bone-healing organ’. Finally, for strains above 100%, the ‘bone-healing organ’ stops and fails to heal, leading to non-union.
Structured oral examination question 4
CANDIDATE: Osteoblasts, osteoclasts, osteocytes, bone lining cells and osteoprogenitor cells.
COMMENT: Do not forget osteoprogenitor cells that are derived from primitive mesenchymal cells and are located in the inner cellular layer of the periosteum, the endosteum and the lining of osteonic canals (Figure 20.10).
Figure 20.10 Bone remodelling. The origins and locations of bone cells.
Figure 20.11 Candidate drawing. Cross-section of (a) long bone and (b) osteon.
EXAMINER: What do they all do?
CANDIDATE: Osteoblasts are large cells responsible for the synthesis and mineralization of bone during both initial bone formation and later bone remodelling. Osteoblasts form a closely packed sheet on the surface of the bone, from which cellular processes extend through the developing bone. Osteocytes lie within the substance of fully formed bone. They lie within a small space called a lacuna, which is contained in the calcified matrix of bone. Osteocytes are derived from osteoblasts, or bone-forming cells, and are essentially osteoblasts surrounded by the products they secreted. Cytoplasmic processes of the osteocyte extend away from the cell toward other osteocytes in canaliculi. These canaliculi allow nutrients and waste products to be exchanged to maintain the viability of the osteocyte. [Examiner cut me off, as he realized I would sit and regurgitate the whole book!]
CANDIDATE: Osteoclast precursors are a member of the monocyte/macrophage family, and, although the resorptive cell can be generated from mononuclear phagocytes of various tissue sources, the principal precursor resides in the marrow. RANKL is produced by osteoblasts, binds to immature osteoclasts and stimulates differentiation into active mature osteoclasts and macrophage colony stimulating factor (M-CSF). Osteoprotegerin inhibits bone resorption by binding and inactivating RANKL.
COMMENT: A lot of candidates get confused in this section.
CANDIDATE: MSCs are multipotent stem cells that can differentiate into a variety of cell types. Cell types that MSCs have been shown to differentiate in vitro or in vivo include osteoblasts, chondrocytes, myocytes and adipocytes.
CANDIDATE: There are three types of cartilage: hyaline cartilage, fibrocartilage and elastic cartilage.
COMMENT: Elastic cartilage exists in the epiglottis and the eustachian tube.
EXAMINER: Draw a cross-section of articular cartilage (Figure 20.12).
Figure 20.12 Articular cartilage layers.
COMMENT: Draw and talk about the different layers. This is one of the commonest questions to get asked in the basic science viva, know it well! Fail to draw this diagram at your peril! Practise the drawing and discussion of it at least 10 times.
EXAMINER: What are the differences between articular cartilage and meniscus?
CANDIDATE: Articular cartilage is 68–85% water, 10–20% (type II) collagen and 5–10% proteoglycans. Meniscus is 60–70% water, 15–25% (type II) collagen and 1–2% proteoglycans.
COMMENT: It’s easy to get confused with these facts.
COMMENT: Draw the standard picture seen in many text books (Figure 20.13).
Figure 20.13 Collagen and proteoglycan arrangement in articular cartilage.
CANDIDATE: 30% of the total water exists between the collagen fibres and this is determined by the negative charge of the proteoglycans which lie within the collagen matrix. Because the proteoglycans are bound closely, the closeness of the negative charges creates a repulsion force that must be neutralized by positive ions in the surrounding fluid. The amount of water present in cartilage depends on the concentration of proteoglycans and the stiffness and strength of the collagen network. The proteoglycan aggregates are basically responsible for the turgid nature of the cartilage and in articular cartilage they provide the osmotic properties needed to resist compressive loads. If the collagen network is degraded, as in the case of OA, the amount of water in the cartilage increases because more negative ions are exposed to draw in fluid. The increase in fluid can significantly alter the mechanical behaviour of the cartilage.
EXAMINER: Bearing in mind what we have discussed, what do you want to talk about next?
EXAMINER: Correct answer! Tell me about any classifications you know of specific to the growth plate.
CANDIDATE: Salter–Harris fractures are classified from types I to V in the order of prognosis, with Salter–Harris Type V having the poorest prognosis and the greatest impact on potential deformity. SH I is a slipped growth plate, II the fracture lies metaphyseal, III is epiphyseal, IV both metaphyseal and epiphyseal and in V the physis suffers a compression injury. Salter–Harris Type II fractures are the most common. When all types of Salter–Harris fractures are considered, the rate of growth disturbance is approximately 30%. However, only 2% of Salter–Harris fractures result in a significant functional disturbance.
The fracture types described later, also less common, include:
Type VI (injury to the perichondral structures – rare).
Type VII (isolated injury to the epiphysis only).
Type VIII (isolated injury to the metaphysis).
Type IX (an injury to the periosteum which could interfere with membranous growth).
COMMENT: I would not mention any of this unless you get asked. You may end up speaking to an orthopaedic paediatric professor!
EXAMINER: Draw me a growth plate.
CANDIDATE: [I drew a simple schematic like Figure 20.14]
Zone I is the reserve or resting zone with low rates of proliferation, proteoglycan synthesis and typeIIB collagen. These cells have a high lipid bodyand vacuole content which is involved withstorage for later nutritional requirements. This isnot a germinal layer of ‘mother cartilage cells’.
Zone II is the upper proliferative or columnarregion. The function of the proliferative zones ismatrix production and cell division that results in longitudinal growth. Chondrocytes are flat andare arranged longitudinally. The zone is the truegerminal layer of the growth plate and Type IIcollagen synthesis is increased.
Zone III is the mature proliferating zone,morphologically similar to zone II, but with less DNA synthesis.
Zone IV is the hypertrophic zone, where cell size increases, and the columnar arrangement is lessregular. Metabolic activity is high, with matrixsynthesis approximately threefold compared to the proliferative zone; the main matrix componentssynthesized are types II and X collagenand aggrecan.
[I got stopped at this point but will continue for completeness]
Zone V is the zone of the matrix calcification as this calcified matrix becomes the scaffolding forbone deposition in the metaphysis. High levels of alkaline phosphatase synthesis of type X and type II collagen cell death by hypoxia.
Zone VI is the junction of the growth plate with the metaphysis, the region where the transitionfrom cartilage to bone occurs. Type I collagen, amarker of the osteoblast phenotype, isimmunolocalized to this area.
Figure 20.14 Diagram of a growth plate. B, bone; OB, osteoblast; CC, calcified cartilage; C, cartilage matrix.
COMMENT: There are a lot more facts in many textbooks, but if you know roughly about each zone that will be fine for a pass, more detailed knowledge will equal a good pass.
The zones of the growth plate can be confusing as many textbooks use a variety of differing names, some referring to cell function, others to cell morphology.
A non-controversial standard textbook zone description would be: (1) reserve zone, (2) proliferating zone, (3) hypertrophic zone which can be subdivided into (a) maturation zone, (b) degenerative zone, (c) zone of provisional calcification, (4) metaphysis subdivided into (a) primary spongiosa and (b) secondary spongiosa.
EXAMINER: How is the growth plate regulated?
CANDIDATE: The growth plate is regulated by growth factors, hormones and vitamins. Growth factors include insulin-like growth factor, which is one of the most potent GF for skeletal tissue, previously known as somatomedins. They have significant effects on the growth plate chondrocytes, and IGFs retained in bone matrix are important in the regulation of bone remodelling. IGFs stimulate osteoblast and chondrocyte proliferation, induce differentiation in osteoblasts and maintain the chondrocyte phenotype.
Transforming growth factors (TGFs) have an important role in skeletal tissue, particularly certain members of the TGF-b gene family which includes the bone morphogenetic proteins involved in morphogenesis and regulation of endochondral ossification and in bone remodelling. BMPs are the only molecules so far discovered capable of independently inducing endochondral ossification in vivo.
COMMENT: Urist 1965 is the paper to quote.4 Be able to mention something about BMP2 and BMP7.
Key message5
Over 50 years ago, Urist made the key discovery that demineralized bone fragments implanted either subcutaneously or intramuscularly in animals induced bone formation.
The extracellular matrix of bone contains substances that can stimulate new bone formation when implanted into extraskeletal sites in a host. These substances were later identified as bone morphogenetic proteins (BMPs).
Both BMP2 and BMP7 are approved for use in acute tibial fractures and complex non-unions.
They increase the local signals needed to initiate the cascade of bone healing. Both BMP2 and BMP7 have been shown to induce ectopic bone formation. At present, the use of BMP2 is preferred as studies suggest it may be more effective than BMP7 at promoting healing and it is also less costly.
Study outcomes can be highly variable according to indication, implying that more rigorous prospective studies are needed to precisely identify the fracture population, timing and delivery mechanism via which BMP can be used to optimize bony healing.
Fibroblast growth factor stimulates proliferation of mesenchymal cells in the developing limb that leads to limb outgrowth. This group is also involved in later stages of bone growth.
Platelet-derived growth factor plays a role in bone development and growth, being important in the regulation of bone and cartilage cells, although little is currently known of their role in normal endochondral ossification.
Tumour necrosis factors stimulate bone and cartilage resorption and division, and reversibly inhibit ectopic bone formation in animal models.
If you get to this stage it’s a good pass. Other growth factors to think about are: interleukin I,6,8, interferons, colony-stimulating factors, parathyroid hormone-related peptide and calcitonin gene-related peptide.
2. Structure and function of cartilage: (a) articular
Introduction
This is an A-list basic science viva topic that almost always appears with each diet of exams. In practical terms this means a candidate has a 1 in 4 or 25% chance of being asked this topic.
In the past, there was a predictable line of questions the examiners would ask. However, most candidates are now more aware of A-list topics than ever before and have pre-learnt their answers. As such the challenge for the Intercollegiate Board is to avoid asking the same questions each and every exam sitting.
To make the subject less predictable the topic focus can be changed mid viva onto different more detailed areas within this large topic such as proteoglycan structure and function.
The flip side is that regular examiners become more familiar with the topic and on occasion may ask esoteric questions to stretch you out to see if you are a possible score 7/8 candidate. What is the function of the tidemark and what insets into it, etc.
One word of caution with A-list topics is that candidates can continue to read further and further into a subject and end up concentrating on unfocused minutia details that have no relevance to any possible viva question likely to be asked.6 This is very different to reading extra details, but being able to apply these details into higher-order thinking to better answer a question. However, it is sometimes a thin line between the two.
Props
Candidates may immediately be handed a laminated photograph of articular cartilage at the start of the viva or be asked a couple of warm-up questions before being asked to draw out the structure of articular cartilage. Articular cartilage is definitely on the top 10 list for ‘must-know’ how-to-draw diagrams.
Structured oral examination question 1
EXAMINER: What are the functions of articular cartilage?
CANDIDATE: The two main functions are:
To provide a smooth, low-friction, lubricated surface for joint motion (lubrication).
The surfaces roll or glide during motion.
Hyaline cartilage should be thought of as a fluid-filled wear-resistant surface.
It reduces the coefficient of friction down to 0.0020, which is 30 times superior to the best performing artificial joint.
To resist the compressive forces encountered across the joint under loading (shock absorber).
COMMENT: These are the two main functions of articular cartilage, although some textbooks mention other minor roles.7 There is some controversy as to the relative importance of articular cartilage as a shock absorber. Some authors have suggested that because it is a very thin structure it has negligible shock-absorbing capacity compared to the surrounding muscle and bone. Again, we suggest stay simple and don’t mention this standpoint unless it comes up in discussion (very unlikely).8
EXAMINER: Can you draw the histological appearance of articular cartilage?
[Draw the structure of articular cartilage.9]
What are the articular cartilage layers?
COMMENT: This is one of the commonest diagrams candidates will be asked to draw in the basic science viva. Fail to master this diagram at your peril. Candidates should be able to draw the various layers of articular cartilage without hesitation. Candidates may then be asked to explain why the layers appear like this, with reference to the three-dimensional ultrastructure.10
CANDIDATE: The histological appearance of articular cartilage is structured into zones …:
COMMENT: Take the examiner sequentially through the layers. Focus your discussion on (1) the differing orientation of type II collagen fibrils, (2) orientation and cellular features of the chondrocytes.
Why is there a different pattern of collagen orientation through the layers of cartilage?
How does the differing pattern of collagen affect its properties?
You ‘the candidate’ should explain this as you draw out articular cartilage and not allow the examiner to get in and ask you these questions.11,12
Structure. The histological structure can be divided into four zones.
1. Superficial (tangential/gliding) zone: 10–20% of thickness.
Collagen fibres are arranged parallel to the joint surface, forming a dense mat.
The most superficial part is called the lamina splendens,13 providing a very low-friction lubrication surface. It contains no cells, a clear film of collagen fibrils with little proteoglycan. This dense collagen arrangement reduces leakage of proteoglycans from the articular surface and protects it from the effects of harmful enzymes.
Below this is a cellular layer with chondrocytes parallel to surface, flat-shaped, high density, many cells 1–3 thick.
This layer provides good resistance to shear forces due to tangential arrangement of collagen and provides the greatest tensile strength.
Low metabolic activity, hence low healing potential.
Thinnest layer with the highest concentration of collagen and water and the lowest concentration of proteoglycan.
Water can be squeezed out of the layer to help create lubrication.
May function as a barrier to the passage of large molecules from the synovial fluid.
2. Middle (transitional) zone: 40–60% of thickness.
Collagen fibres arranged obliquely at right angles to each other.
Plentiful concentration of proteoglycan.
Chondrocytes arranged in random orientation, round shape, progressively lower density and fewer cells.
Transitional zone between the shearing forces of the surface layer and resistance to compression in the deep layer.
3. Deep (radial) zone: 30% of thickness.
Provides resistance to compression.
Collagen fibres vertically arranged (perpendicular to articular cartilage) cross the tidemark and are anchored to subchondral bone.
Highest concentration of proteoglycans.
Chondrocytes spherically arranged in vertical columns.
Collagen fibres largest diameter.
Lowest water content.
4. Calcified zone.
This separates the cartilage tissue from the underlying subchondral bone.
Anchor for the various layers.
Collagen type X and hydroxyapatite crystals anchor articular cartilage to subchondral bone.
Forms a barrier to blood vessels supplying subchondral bone.
Matrix mineralization in the calcified zone allows gradual transition of mechanical properties between cartilage and bone.
Tidemark
The junction between the deep and calcified zone is called the tidemark.
COMMENT: As a candidate is describing the histological structure of articular cartilage an examiner may start to probe/interrupt/take over14 and ask esoteric15 questions.
EXAMINER: What is the tidemark? What attaches to the tidemark?
CANDIDATE: The tidemark provides resistance to shear. It is the boundary between the calcified and uncalcified cartilage. It is cell-free and represents a calcification front. The collagen fibres in the deep zone penetrate through the tidemark into the calcified cartilage to provide structural stability for articular cartilage on the subchondral bone.
It is a smooth, basophilic undulating line that is critical for the transmission of load from cartilage to bone.
EXAMINER: What is the composition of articular cartilage (Table 20.3)?
CANDIDATE: The wet weight proportions of articular cartilage are water (65–80%), collagen (10–20%), proteoglycans (10–15%), chondrocytes (5%) and other matrix components such as adhesives and lipids.
COMMENT: This isn’t a wrong answer, but it’s a bit unstructured and cumbersome. A better answer would be to say articular cartilage is composed of cells (chondrocytes) accounting for 5% of the wet weight and extracellular matrix the remaining 95%. The extracellular matrix is made up of fibres and ground substance. The fibres account for 10–20% and are almost exclusively Type II collagen. Water accounts for between 65% and 80% of the extracellular matrix, proteoglycans and glycoaminoglycans 10–15% with small amounts of glycoproteins and degradative enzymes.
Or more simply, articular cartilage is mainly composed of chondrocytes, water, Type II collagen, proteoglycans and a variety of matrix proteins.
COMMENT: Do not mix around the wet and dry weight percentages of articular cartilage components; preferably stick to wet weight. If you have to talk about dry weight mention that collagen accounts for 40–70% of the dry weight and that approximately 90% of the dry mass of articular cartilage is made up of proteoglycan aggrecan, type II collagen and hyaluronan.
EXAMINER: What are the contents of articular cartilage?
COMMENT: There is an overlap between describing the layers and discussing the contents of articular cartilage.
Chondrocytes16
Derived from mesenchymal stem cells, chondrocytes produce and maintain ECM, and are the main cell type of articular cartilage.
Deeper cartilage zones contain no chondrocytes.
Low metabolic rate.
Chondrocytes have no contact with neighbouring cells and are spheroidal.
There are distinct subpopulations of chondrocytes in the different zones of cartilage whose properties differ in terms of their morphology, metabolism, and their response to cytokines.
Cell morphology varies according to zone.
The superficial zone contains a high density of flat cells with approximately three times as many cells in the region compared to the larger cells of the deeper zone.
In the deeper zones, they are arranged in columns, reflecting the articular cartilage role in growth of the epiphysis.
Water
Up to 80% of the extracellular matrix.
Permits load-dependent deformation of articular cartilage by its movement both in and out and within cartilage.
Increased water content leads to increased permeability, decreased strength and decreased elasticity.
Provides a medium for lubrication.
Collagen
About 10–20% wet weight, 60% dry weight.
Gives the articular cartilage its tensile stiffness.
The main collagen in articular cartilage is type II accounting for 90–95% of the collagen.
Types II, IX and XI form a mesh that serves to trap proteoglycans, providing for stiffness and strength.
Type VI helps chondrocytes adhere to the matrix. Increases in early OA.
Type XI constrains the proteoglycan matrix.
Type X is only found near the calcified zone.
Proteoglycans
Proteoglycans are complex macromolecules composed of a protein core to which many glycosaminoglycan side chains are attached.
Proteoglycans trap and hold water, providing the tissue with its turgid nature that resists compression.
They are secreted by chondrocytes.
The most common glycosaminoglycan in articular cartilage is chondroitin-sulphate (two subtypes, chondroitin-4-sulphate and chondroitin-6-sulphate), then keratin sulphate and dermatan sulphate.
Chondroitin-4-sulphate is the most abundant and decreases over the years; chondroitin-6-sulphate remains constant; and keratin sulphate increases with age.
Glycosaminoglycan can link to a protein core by sugar bonds to form a proteoglycan aggrecan (see Figure 20.14).
A proteoglycan aggrecan has three globular domains, G1, G2 and G3. G1 and G2 are near the N terminus and separated by a short interglobular domain. The third globular domain, G3, is near the C terminal of the core protein.
Aggrecan molecules do not exist in isolation within the extracellular matrix, but as proteoglycan aggregates through the interaction with the polysaccharide, hyaluronan.
Each aggregate is composed of a central filament of hyaluronic acid with up to 100 aggrecan molecules radiating from it, with each interaction stabilized by the presence of a link protein.
PG aggregation promotes immobilization of the PGs within the fine collagen meshwork.
PGs have an average lifespan of 3 months and have a great capacity for retaining water, which gives compressive strength and elasticity to the tissue.
Figure 20.15 Proteoglycan aggrecan. A proteoglycan aggrecan has three globular domains, G1, G2 and G3.
COMMENT: This involves differentiating between a proteoglycan aggregate and proteoglycan aggrecan molecule (Figure 20.14). It is not uncommon for candidates to be asked to draw out the structure of either a proteoglycan aggregate or aggrecan molecule (Figure 20.16) or sometimes both.
Volunteer to draw both out for the examiners. As always, rehearse your drawing with dialogue as many times as needed to obtain a smooth, polished flow.
Figure 20.16 Candidate drawing of proteoglycan aggregate.
EXAMINER: How does collagen synthesis take place? (Figure 20.17.)
Figure 20.17 Collagen synthesis.
COMMENT: This question19 may be asked as part of the main viva theme or as an add-on if a candidate has trail-blazed through previous viva questions and is on for a score 8. The secret is to try and simplify a complex process often poorly described in books. Split the process into intracellular and extracellular events.
CANDIDATE: Procollagen is synthesized by a series of steps within the endoplasmic reticulum of cells such as fibroblasts.
This molecule is then released into the extracellular space by transport through the Golgi apparatus and converted into collagen by procollagen peptidases that cleave the water-soluble, non-helical N- and C-terminal portions of the procollagen molecule to form tropocollagen.
Collagen monomers are then covalently cross-linked with each other after certain residues are oxidized by lysyl oxidase.
Table 20.3 Constituents of articular cartilage.
Cells (chondrocytes) (5%) | |||
Extracellular matrix (95%) | Fibres | Collagen (10–20%) | Type II, IX, XI |
Almost exclusively Type II | Type VI, X | ||
Elastin | |||
Ground substance | Water (65–80%) | ||
Proteoglycans and glycosaminoglycans (10–15%) | |||
Glycoproteins | |||
Degradative enzymes (matrix metalloproteinases) |
Steps that occur INSIDE the cell
Synthesis of pro-alpha chain.
Hydroxylation of selected proline and lysine residues.
Glycosylation of selected hydroxylysine residues.
Self-assembly of three pro-alpha-chains into triple helix.
Procollagen triple helix formation.
Then extrusion of procollagen from the endoplasmic reticulum/Golgi compartment into secretory vesicles and then secretion into extracellular matrix.
Steps that occur OUTSIDE the cell
Cleavage of propeptides. Once secreted, procollagen peptidases remove the N-terminal and C-terminal propeptides.
Self-assembly into fibril.
Aggregation of collagen fibrils to form a collagen fibre. Formation of covalent cross-links by the enzyme lysyl oxidase. This enzyme allows hydroxyl groups on lysines and hydroxyl lysines to be converted into aldehyde groups that covalently bond between tropocollagen molecules to form a collagen fibril.
Closely related to synthesis is the structure of collagen and the line of questions may continue on with this subtopic.20
EXAMINER: What is the structure of collagen (Figure 20.18)?
Figure 20.18 Hierarchical structure of collagen ranges from the amino acid sequence, tropocollagen molecules, collagen fibrils to collagen fibres.
CANDIDATE: Collagen is a triple helix of three polypeptide chains.
The amino acids are glycine, proline and lysine.
In articular cartilage collagen is mainly type 2.
CANDIDATE: These interact with collagen fibrils and stabilize the matrix framework.
They help chondrocytes bind to the macromolecules of the matrix.
Matrix glycoproteins are much smaller than aggrecans.
More simply, they act as a tissue glue binding to various matrix components.
CANDIDATE: These degrade collagen and proteoglycan aggregates as part of the normal turnover of the matrix.
CANDIDATE:
Water is attracted and retained in articular cartilage by the ionic pressure created by the high level of negative charges on glycosaminoglycan (GAG) chains on proteoglycan molecules.
The ionic pressure creates a swelling pressure, which will keep imbibing water until it is resisted by the tension in the fibrous component of cartilage, the collagen fibres.
As proteogylcans are bound closely, the closeness of the negative charges creates a repulsion force that must be neutralized by positive ions in the surrounding fluid. The amount of water present in cartilage depends on the concentration of proteoglycans and the stiffness and strength of the collagen network. The proteoglycan aggregates are basically responsible for the turgid nature of the cartilage and in articular cartilage they provide the osmotic properties needed to resist compressive loads. If the collagen network is degraded, as in the case of OA, the amount of water in the cartilage increases because more negative ions are exposed to draw in fluid. The increase in fluid can significantly alter the mechanical behaviour of the cartilage.
COMMENT: Candidates that do not understand this concept particularly well may become trapped quite easily by the examiners (just like water gets trapped by proteoglycans).
EXAMINER: Tell me about matrix metabolism.
CANDIDATE: The production of extracellular matrix, its organization, maintenance and breakdown are controlled by the chondrocyte.
Chondrocyte regulators include hormones, cytokines and growth factors. Turnover of the matrix is needed for remodelling and maintenance. Enzymes include aggracanase and metalloproteinases degrade the matrix. The chondrocytes also make inhibitors of these enzymes called tissue inhibitor of metalloproteinases (TIMP).
EXAMINER: What do we mean by the term matrix region?
CANDIDATE: An alternative zonal classification is by the matrix regions. The matrix is divided into three distinct regions with respect to the distance from the chondrocyte cell membrane.
Matrix regions differ in their collagen content, collagen fibril diameter, collagen fibril orientation and proteoglycan and non-collagenous protein content and organization.
There are three regions.
1. The pericellular matrix is a thin layer adjacent to the cell membrane, completely surrounding the chondrocyte. It contains mainly proteoglycans, as well as glycoproteins and other non-collagenous proteins.
The pericellular matrix region may play a functional role to initiate signal transduction within cartilage with load bearing.
2. The territorial matrix surrounds the pericellular matrix; it is composed mostly of fine collagen fibrils, forming a basket-like network around the cells.
The territorial matrix may protect the cartilage cells against mechanical stresses and may contribute to the resiliency of the articular cartilage structure and its ability to withstand substantial loads.
3. The interterritorial region is the largest of the three matrix regions; it contributes most to the biomechanical properties of articular cartilage.
This region is characterized by randomly oriented bundles of large collagen fibrils and large amounts of proteoglycans.
Structured oral examination question 2
Articular cartilage changes with ageing versus osteoarthritis is more of a section 3 (pathology) topic but there will be a large overlap with section 2 material (structure and function of articular cartilage). Throw in management options for a cartilage defect and this topic is equally at home in an adult and pathology viva.
CANDIDATE: Articular cartilage undergoes significant structural, matrix composition and mechanical changes with age. With increasing age there is an age-related decline in the ability of chondrocytes to maintain the tissue. Chondrocytes become less responsive to the proliferative and anabolic effects of growth factors.
There is a marked increase in the formation of advanced glycation end-products (AGEs). This results in increased cross-linking of collagen molecules altering the biomechanical properties of cartilage resulting in increased stiffness and an increased susceptibility to fatigue failure.
COMMENT: Candidates may be asked to directly compare the biomechanical changes of ageing with osteoarthritis in cartilage. This can be rote-learned from various tables, but a more detailed understanding is a safer bet in case follow-up questions focus in on more comprehensive detail.
EXAMINER: Describe the changes in articular cartilage with osteoarthritis.
What pathological processes are involved in the development of osteoarthritis?
CANDIDATE: The process can be divided into three overlapping stages: (1) cartilage matrix damage, (2) chondrocyte response to tissue damage (3) decline of the chondrocyte synthetic response with progressive loss of tissue.
In the early stages of disease, loss of proteoglycan is reversible, whereas at later stages there is irreversible loss. The earliest visible change is loss of collagen integrity resulting in tissue fibrillation and increased water content. Decreased aggrecan concentration and aggregation and decreased glycosaminoglycan chain length all increase the permeability and stiffness of the matrix and make it vulnerable to further mechanical damage. Despite chondrocyte proliferation and increased collagen and proteoglycan synthesis this response fails to halt disease progression. There is progressive loss of articular cartilage and a reduced chondrocyte anabolic and proliferative response. This is related to a downregulation of chondrocyte function.
With osteoarthritis, the cartilage may show areas of softening, fibrillation, fissures or gross erosion. There may be areas of full-thickness cartilage loss with the subchondral bone exposed and often sclerotic.
Microscopic appearances include surface irregularities and erosions, deterioration of the tidemark, fissuring and damage to the cartilage structure. Water content is increased in OA, with a decrease in the proteoglycan content. The proteoglycan chain is shorter, and the chondroitin/keratin sulphate ratio is increased.
Overall, the collagen content is maintained but the presence of collagenase disrupts its organization and orientation.
CANDIDATE: Conservative measures include targeted physiotherapy, regular oral or topical analgesia/anti-inflammatories, intra-articular injections (corticosteroids or hyaluronic acid) and activity modification.
Surgical management may include joint debridement, osteotomy, arthroplasty and arthrodesis.
CANDIDATE: There are three main types of cartilage injury: (1) superficial matrix disruption, (2) partial thickness defects and (3) full-thickness defects.
Superficial matrix disruption arises from blunt trauma whereby the ECM is damaged but viable chondrocytes aggregate into clusters and are capable of synthesizing new matrix.
Partial thickness defects disrupt the cartilage surface but do not extend into the subchondral bone. These defects are unable to self-repair.
Full-thickness defects arise from damage that penetrates deep into the subchondral bone. These defects can elicit a repair response due to access to marrow cells; however, they are typically filled with fibrocartilage. This type of repair tissue is much weaker than hyaline cartilage and displays poor long-term performance due to poor compressive strength and durability.
EXAMINER: What are the options for treating a symptomatic focal articular cartilage defect in the medial femoral condyle of the knee of a young active patient?21
CANDIDATE: Appropriate management of an articular cartilage defect in a younger patient is often very challenging. Although the natural history of an isolated articular cartilage lesion is not completely understood, these defects may to lead to significant morbidity and progress to diffuse osteoarthritis in time.
Current treatment options fall into three broad categories:
1. Mesenchymal stem cell (MSC) stimulation (microfracture, drilling, abrasion chondroplasty).
2. Substitution options (osteochondral autograft transfer system [OATS], osteochondral allograft).
3. Cell-based, biological replacement options (autologous chondrocyte implantation [ACI], stem cell therapy, tissue engineering).
Marrow stimulation techniques such as abrasion arthroplasty and microfracture penetrate the subchondral bone, causing bleeding within the cartilage defect that leads to fibrin clot formation. Undifferentiated MSCs from the bone marrow migrate into the defect, proliferate and differentiate into fibrochondrocytes. These induce the formation of fibrocartilage repair tissue.
Although excellent short-term clinical outcomes have been reported, the clinical durability of marrow-stimulated repair tissue declines with longer follow-up. The repaired articular cartilage generally fails to replicate the structure, composition and function of normal articular cartilage.
OATS is recommended for smaller lesions, lesions in high-demand athletes, and lesions with associated bone loss.
Microfracture is suited for medium-size defects with little or no bone loss in lower-demand older patients.
Structured oral examination question 3
As viva question 1 initially: what are the functions of AC, draw out and describe the layers of AC, etc. and then a focus midway through on the biomechanical properties of articular cartilage.
CANDIDATE: Cartilage is a biphasic, viscoelastic and anisotropic material demonstrating both creep and stress relaxation.
COMMENT: There is a debate whether cartilage is a biphasic (fluid and solid phase) or triphasic material (see below).
CANDIDATE: A viscoelastic material will exhibit a time-dependent behaviour when subjected to a constant load or constant deformation.
EXAMINER: What are the properties of a viscoelastic material?
CANDIDATE: A viscoelastic material demonstrates creep and stress relaxation.
Creep is time-dependent deformation of a material under constant load that is below its yield strength.
Stress relaxation is the decrease in stress required to maintain constant strain over time.
COMMENT: When a constant compressive stress (load/area) is applied to the tissue, its deformation will increase with time; it will creep until an equilibrium value is reached. Creep produces plastic deformation of a material.
When the tissue is deformed and held at a constant strain, the stress will rise to a peak, followed by a slow stress–relaxation process until an equilibrium value is reached.
COMMENT: Viscoelastic materials display four characteristics:
1. Creep.
2. Stress relaxation.
3. Hysteresis.
4. Strain rate-dependent mechanical properties.
Hysteresis occurs when a viscoelastic material is cyclically loaded and unloaded. It is the ability of the material to dissipate energy between loading and unloading cycles. It is due to the fact that materials do not perfectly obey Hooke’s law. A viscoelastic material is harder to deform when loading than unloading.
Viscoelastic materials are stiffer, tougher and stronger when loaded at a faster rate (higher strain rate) because there is less time for them to strain. A given load produces less deformation over a shorter period of time than a longer period of time.
EXAMINER: Can you draw out the graphs of creep and stress relaxation (Figures 20.19 and 20.20)?
CANDIDATE: Yes, but my mind has gone blank.
EXAMINER: What about hysteresis and strain-dependent mechanical properties (Figures 20.21 and 20.22)?
Figure 20.22 Time-dependent strain behaviour. Stress is proportional to strain rate.
CANDIDATE: No, sorry.
COMMENT: These are predictable questions. The viva is heading for a score 4 or at best 5.
EXAMINER: What about articular cartilage permeability with compression?
CANDIDATE: Articular cartilage permeability decreases non-linearly with compression. This serves to regulate the response of cartilage to compression by preventing rapid and excessive fluid exudation from the tissue with compression loading and by promoting interstitial fluid pressurization for load support. It also regulates the ability of cartilage to dissipate energy during cyclic loading.
There are two causes for this nonlinear effect.
As the tissue is compressed:
(1) The water content or porosity is reduced.
(2) The density of the negative charges on the proteoglycans is increased.
There is a direct relationship between permeability and water content and an inverse relationship between permeability and proteoglycan content.
COMMENT: This is an esoteric question that tests knowledge of the interaction between proteoglycan and water. This is in the score 8 zone for candidates.
EXAMINER: How does the internal architecture of articular cartilage relate to its biomechanical properties?
CANDIDATE: The presence of water within the tissue allows the support of most of the load through pressurized fluid. This fluid support is not uniform between the different zones of the tissue, with the superficial zone demonstrating the highest support (95%) compared to the deep zone (70% of applied load). Over time there is a decreased interstitial fluid support that causes an increased loading of the solid phase including chondrocytes. As such, articular cartilage can be thought of as a biphasic, porous model.
However, dissolved electrolytes together with fixed charges of the solid matrix bring about mechanoelectrochemical phenomena adding to the load bearing of the tissue and described as a third phase.
The triphasic nature of cartilage explains how fluid is drawn back into the cartilage matrix after being excreted during compression. While the triphasic nature of cartilage is a physically intuitive way to model cartilage, it is also very complex.
COMMENT: The biomechanical properties of articular cartilage can be complicated to understand and tests higher-order thinking relating to structure.
Biphasic creep behaviour of articular cartilage during compression. Rate of creep is governed by the rate at which fluid is forced out from the tissue, which, in turn, is governed by the permeability and stiffness of the porous-permeable, collagen–proteoglycan solid matrix.
Structured oral examination question 4
EXAMINER: What are the properties of articular cartilage?
CANDIDATE: Cartilage is avascular, aneural and alymphatic.
EXAMINER: What are the articular cartilage changes that occur with ageing and osteoarthritis (Table 20.4)?
COMMENT: This is an A-list basic science question. While tables provide a succinct summary they do not give a candidate the opportunity to practise out loud and rehearse their answer. Perhaps best remembered as changes occurring in the composition of articular cartilage, i.e. water, chondrocytes, collagen and proteoglycans.
EXAMINER: What are the changes that occur with osteoarthritis?
CANDIDATE: With osteoarthritis, the cartilage may show areas of softening, fibrillation, fissures or gross erosion. There may be areas of full-thickness cartilage loss with the subchondral bone exposed and often sclerotic.
Microscopic appearances include surface irregularities and erosions, deterioration of the tidemark, fissuring and damage to the cartilage structure. Water content is increased in OA, with a decrease in the proteoglycan content. The proteoglycan chain is shorter, and the chondroitin/keratin sulphate ratio is increased.
Overall, the collagen content is maintained, but the presence of collagenase disrupts its organization and orientation.
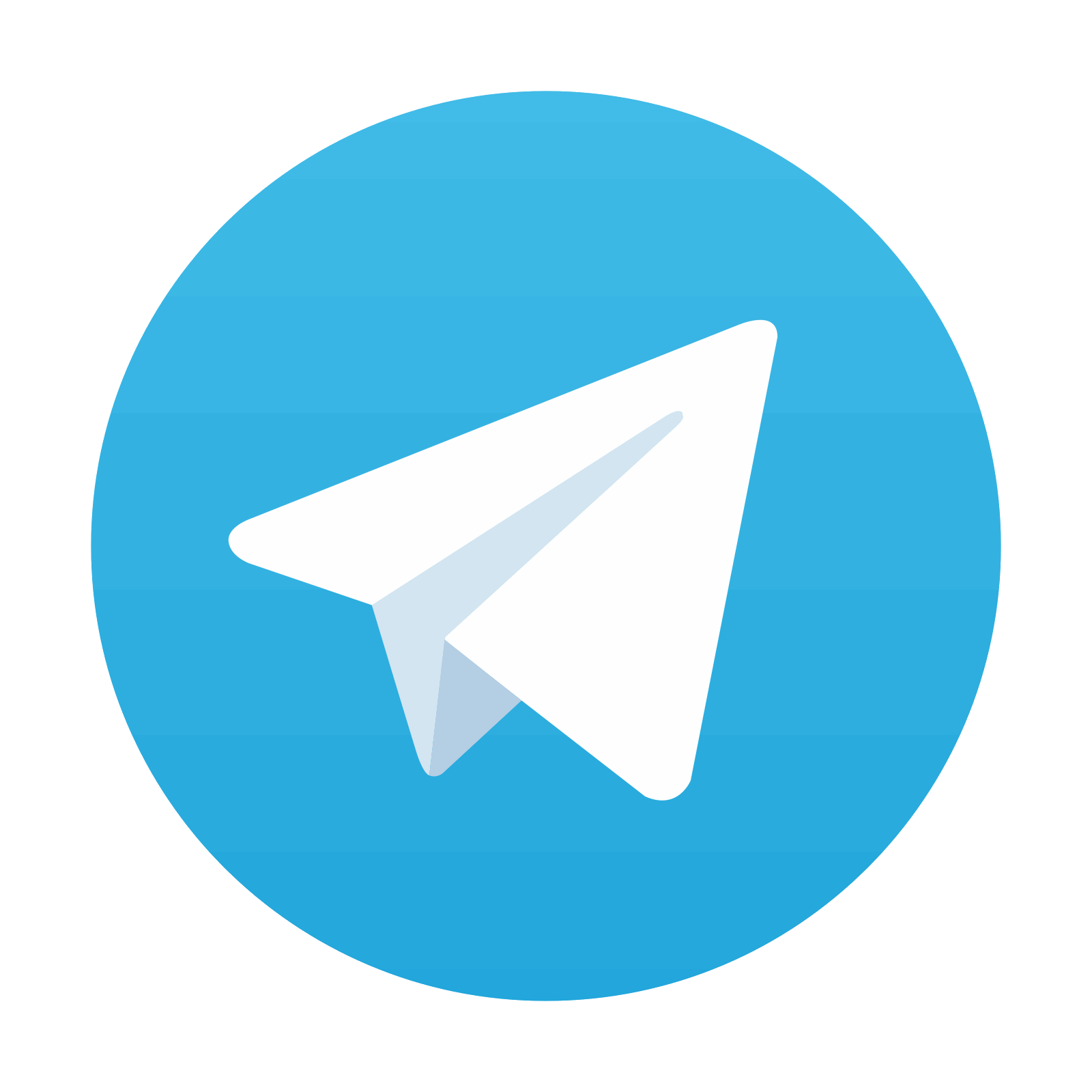
Stay updated, free articles. Join our Telegram channel

Full access? Get Clinical Tree
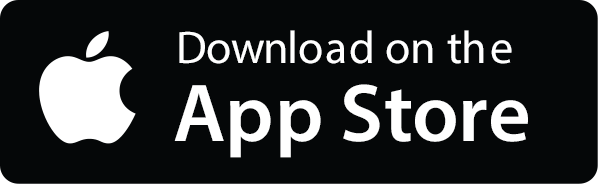
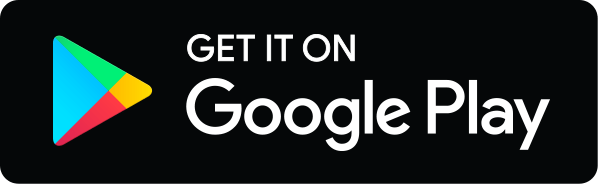
