Introduction
This study evaluated the masseter muscle changes after surgical-orthodontic treatment in patients with a skeletal Class III malocclusion using automatic segmentation.
Methods
Images of 120 patients with skeletal Class III malocclusion were obtained and reconstructed at T0 (pretreatment), T1 (presurgery), and T2 (6-12-month postsurgery). The patients were divided into symmetrical and asymmetrical groups. The volume, major axis length, maximum cross-sectional area, horizontal cross-sectional area 5 mm above the mandibular foramen (CSAF), and orientation were calculated automatically.
Results
In the asymmetrical group, the volume and major axis length on the deviated side were lower than on the nondeviated side at T0, T1, and T2 ( P <0.05). There were no significant differences in maximum cross-sectional area and CSAF bilaterally. The orientation was coronally more vertical and sagittally more forward on the deviated side (both P <0.001). In the symmetrical group, there were no significant bilateral differences at T0, T1, and T2. The volume, major axis length, and CSAF decreased, and the coronal orientation was more vertical on the nondeviated side at T2 than at T0 in both groups ( P <0.05). The coronal plane orientation was more inclined on the deviated side at T2 than at T0 in the asymmetrical group ( P <0.05).
Conclusions
The smaller volume on the deviated side at T2 indicates the need for myofunctional training after surgery. The masseter muscle volume and the cross-sectional area did not recover to the preorthodontic levels. Studies with longer follow-up durations are needed to confirm these findings.
Highlights
- •
The automatic masseter muscle segmentation model was used.
- •
The muscle volume remained smaller on the deviated side 6-12 months postsurgery.
- •
Myofunctional training may be required postsurgery on the deviated side.
With the development of society, facial esthetics have played san increasingly significant role in a person’s quality of life. Facial symmetry is an important factor that affects facial esthetics. Studies have reported a higher prevalence of mandibular asymmetry in patients with Class III malocclusion than in those with Class II malocclusion. , Mandibular prognathism and asymmetry have a negative impact on facial appearance, masticatory function, and social psychology. Therefore, patients often seek the help of orthodontists and surgeons for the correction of mandibular prognathism and asymmetry.
Facial asymmetry may be related to the hard tissues, soft tissues, or both. , Facial soft tissues impact visual perception by aggravating or compensating for any underlying skeletal asymmetries. , Clinical asymmetry may be present in patients with asymmetrical soft tissues even in the presence of skeletal symmetry on radiographic analysis. The masseter muscle is located on the surface of the ramus and supports the mandibular angle area. It has a noticeable effect on the facial morphology. ,
The biomechanical features of muscles include size, thickness, cross-sectional area, strength, and orientation. The masseter muscle is an important masticatory muscle, and its cross-sectional area and thickness are important determinants of the magnitude of bite force. , A previous study demonstrated significantly greater occlusal contact areas and bite forces on the deviated sides than on nondeviated sides in patients with skeletal Class III malocclusion with asymmetry. However, there are some controversies about the bilateral morphologic characteristics of the masseter muscle in patients with Class III malocclusion with mandibular asymmetry. ,
Surgical-orthodontic treatment is important for severe craniofacial deformities, including mandibular protrusion, mandibular retrusion, and facial asymmetry. , , , Presurgery orthodontic treatment for dental sagittal and transverse decompensation can alter the masticatory function. , , Although orthognathic surgery is based on skeletal asymmetries, the soft tissue covering the bone surface also changes with 3-dimensional (3D) bony movements. It can also change the attachment position of the masseter muscle. Furthermore, the masseter muscle may also change as a result of the postoperative changes in the masticatory function. Therefore, it has been suggested that the masseter muscle undergoes significant alterations after surgical-orthodontic treatment. , The stability of the surgical-orthodontic treatment is also closely related to the masseter muscle. The soft- and hard-tissue changes caused by surgical-orthodontic treatment affect the facial esthetics and masticatory function and may be a cause of great concern to the patients.
As stated previously, the masseter muscle has an important relationship with facial esthetics, oral function, and the stability of surgical-orthodontic treatment. , There is an overall lack of longitudinal studies on the masseter muscle in patients with skeletal Class III malocclusion with asymmetries after surgical-orthodontic treatment, , and there is no sound evidence regarding postoperative changes in the masseter muscle.
This study evaluated the changes in the masseter muscle morphology after surgical-orthodontic treatment in patients with skeletal Class III malocclusion with and without mandibular asymmetry using the automatic masseter muscle segmentation model.
MAterial and methods
The patient images used in this study were obtained from the Department of Oral and Maxillofacial Surgery and the Department of Orthodontics, Peking University School and Hospital of Stomatology. The study was approved by the Biomedical Ethics Committee of Peking University School and Hospital of Stomatology (PKUSSIRB-201944062).
The inclusion criteria were as follows: (1) aged ≥16 years; (2) skeletal Class III (ANB angle <0°); (3) cone-beam computed tomography (CBCT) or computed tomography (CT) performed at pretreatment (T0), presurgery (T1), and 6-12-month postsurgery (T2), including the entire masseter muscle; and (4) double-jaw surgery with maxillary LeFort I osteotomy and bilateral sagittal split ramus osteotomy (BSSRO).
The exclusion criteria were as follows: (1) missing teeth, retained deciduous teeth, or impacted teeth; (2) severe posterior crowding; (3) severe periodontitis or periapical periodontitis; (4) systemic diseases; (5) cleft lip or palate; and (6) temporomandibular joint disorder.
Based on the inclusion and exclusion criteria, a total of 120 patients with skeletal Class III malocclusion were included ( Table I ). The patients were further divided into 2 subgroups on the basis of the degree of mandibular deviation from the midsagittal plane, measured using 3D CBCT images. The asymmetrical group included 60 patients (17 males and 43 females; average age, 22.13 ± 5.00 years) with mandibular deviation >3.5 mm, whereas the symmetrical group included 60 patients (17 males and 43 females; average age, 22.23 ± 4.71 years) with mandibular deviation <2 mm. The mandibular deviation was evaluated on the basis of menton deviation compared with the midsagittal plane. The deviated side was defined as the side on which the menton shifted toward the midsagittal plane, whereas the other side was defined as the nondeviated side.
Characteristic | Asymmetrical Class III | Symmetrical Class III |
---|---|---|
Sample size (n) | 60 | 60 |
Age (y) | 22.13 ± 5.00 | 22.23 ± 4.71 |
Sex | ||
Male | 17 | 17 |
Female | 43 | 43 |
Vertical facial morphology (n) | ||
High angle | 15 | 15 |
Normal angle | 25 | 25 |
Low angle | 20 | 20 |
Mandibular deviation (mm) | 7.21 ± 3.20 | 1.11 ± 0.72 |
ANB (°) | −2.91 ± 2.58 | −3.70 ± 2.47 |
Postsurgery follow-up duration (mo) | 8.06 ± 2.87 | 7.73 ± 3.81 |
The sample size was calculated on the basis of the bilateral masseter muscle angle, defined as the angle between the Frankfort plane and the anterior border of the masseter muscle in a study by Lee et al. The difference in masseter muscle angles between the deviated and nondeviated sides was 2.5°, and the standard deviation was 3.6°. Considering this as a clinically relevant difference, a minimum sample size of 37 was required in each group for a significant analysis, with a significance level of 0.05 and statistical power of 90%. The sample size was calculated using the PASS software (version 11; NCSS, Kaysville, Utah).
CBCT scans were obtained using a NewTom VGi machine (Newtom, Verona, Italy) with the following settings: a field of view of 24 × 19 cm, 90 kV, and 6.0 mA; a scan time of 15 seconds; and a voxel size of 0.3 mm. As this was a retrospective study, CT was performed before the surgery for some patients. The CT images were acquired using a 64-row spiral CT scanner (Philips Inc, Andover, Mass) at 120 kVp, 230 mA, and 1.0 mm layer thickness. The CT scan resolution was set to 512 × 512 × 236, and the voxel size was 0.4 × 0.4 × 1 mm³.
To standardize the orientation of craniofacial structures, 3D reference planes were set for the T0 CBCT images: (1) the Frankfort plane was defined as the horizontal plane; (2) the midsagittal plane was perpendicular to the Frankfort plane, passing through the nasion and basion; and (3) the coronal plane was perpendicular to these 2 planes, passing through the basion ( Fig 1 ). The reorientation of T0 images was performed using Dolphin Imaging software (version 11.8; Dolphin Imaging and Management Solutions, Chatsworth, Calif).

To ensure consistent image orientation for the same patient at different periods, T1 and T2 CBCT or CT scans were superimposed on the basis of the frontal base area of the oriented T0 scan using the Dolphin Imaging software ( Fig 2 ). The images were then exported in digital imaging and communications in medicine format and used for masseter muscle segmentation and measurements.

In our previous study, a deep learning-based automatic approach was used to accurately segment the masseter muscle from CBCT, refined using high-quality paired CT. There was no significant difference in the auto-segmentation accuracy for CBCT and CT. Therefore, it avoided the errors caused by some T1 images, which were CT scans, and some T1 images, which were CBCT scans. The reoriented T0, T1, and T2 images were imported into the deep learning-based model for auto-segmentation of the deviated and nondeviated masseter muscles ( Fig 3 ). Moreover, in this study, we randomly selected 11 CBCT and 11 CT images from the samples and then made manual segmentation for the masseter muscle of CBCT and CT images. The dice similarity coefficient between manual segmentation and auto-segmentation was applied to test the performance of the deep learning model ( Fig 4 ).


The mesh volume and long axis length were computed using Pyradiomics (version 3.0.1, Brigham and Women’s Hospital, Harvard Medical School, Boston, Mass; https://pyradiomics.readthedocs.io ), a library for radiomic feature extraction from medical imaging ( Table II ).
Variable | Definition |
---|---|
Volume | Mesh volume of the masseter muscle auto-segmentation |
Major axis length | The largest axis length of the segmentation-enclosing ellipsoid, using the largest principal component |
CSAM | Maximum cross-sectional area in all cross-sections of unilateral auto-segmentation parallel to the Frankfort plane |
CSAF | Cross-sectional area 5 mm above the mandibular foramen parallel to the Frankfort plane |
MMFHS | The angle of the regression line and Frankfort plane in the sagittal plane |
MMFHC | The angle of the regression line and Frankfort plane in the coronal plane |
The oriented images were imported into the ITK-SNAP software (version 3.8.0, Penn Image Computing and Science Laboratory (PICSL), Pennsylvania, Pa; http://www.itksnap.org ), and the coordinates of bilateral mandibular foramen were obtained. Using the deep learning-based model, the voxels for each auto-segmentation cross-section of the masseter parallel to the Frankfort plane were obtained, and the maximum cross-sectional area (CSAM) and cross-sectional area 5 mm above the mandibular foramen (CSAF) were calculated ( Table II ).
The coordinates of the centroid for each cross-section were calculated using the deep learning-based model. The centroid coordinates for masseter cross-sections from 1 cm above to 2 cm below the mandibular foramen were fit to the regression line using the least squares principle in MATLAB (version R2018b; MathWorks Inc, Natick, Mass). MMFHS and MMFHC were defined as the angles between the regression line and the Frankfort plane in the sagittal and coronal planes, respectively ( Fig 5 ).

Statistical analysis
The Bland-Altman method was performed to show the agreement between the auto-segmentation and manual segmentation of masseter muscle for CBCT and CT separately ( Fig 4 ). Paired t test was used to compare the parameters between deviated and nondeviated sides in each group ( Tables III and IV ). The independent-sample t test was used to compare the parameters between the asymmetrical and symmetrical groups ( Tables V ). Repeated-measures analysis of variance and Bonferroni post-hoc comparisons were used to compare the changes in parameters from T0 to T2 ( Tables VI and VII ). All statistical evaluations were performed using SPSS software (version 27.0; IBM Corp, Armonk, NY). Significance was defined as P <0.05.
Variables | T0 | T1 | T2 | |||||||||
---|---|---|---|---|---|---|---|---|---|---|---|---|
Deviated | Nondeviated | Difference, % | P value | Deviated | Nondeviated | Difference, % | P value | Deviated | Nondeviated | Difference, % | P value | |
Volume (cm³) | 21.1 ± 6.2 | 21.9 ± 5.8 | −4.9 ± 12.0 | 0.018 ∗ | 19.7 ± 5.9 | 20.6 ± 6.1 | −5.3 ± 10.2 | 0.001 ∗∗ | 18.9 ± 6.1 | 19.5 ± 5.7 | −4.6 ± 11.7 | 0.030 ∗ |
Major axis length (mm) | 64.9 ± 6.8 | 67.4 ± 5.8 | −4.1 ± 6.3 | <0.001 ∗∗∗ | 64.9 ± 6.6 | 67.7 ± 5.8 | −4.8 ± 7.3 | <0.001 ∗∗∗ | 62.6 ± 7.3 | 64.7 ± 6.4 | −3.6 ± 5.6 | <0.001 ∗∗∗ |
CSAM (cm 2 ) | 4.3 ± 0.9 | 4.3 ± 0.9 | −1.8 ± 12.6 | 0.627 | 4.1 ± 0.9 | 4.1 ± 0.9 | −0.4 ± 9.9 | 0.881 | 4.2 ± 0.9 | 4.2 ± 0.9 | −0.6 ± 12.4 | 0.942 |
CSAF (cm 2 ) | 4.1 ± 0.9 | 4.1 ± 0.9 | −0.7 ± 12.2 | 0.995 | 3.8 ± 0.8 | 3.8 ± 0.9 | −0.2 ± 10.0 | 0.923 | 3.9 ± 0.9 | 3.9 ± 0.9 | −0.2 ± 12.9 | 0.741 |
MMFHS (°) | 80.1 ± 5.3 | 82.2 ± 5.3 | −2.9 ± 5.0 | <0.001 ∗∗∗ | 78.0 ± 5.8 | 81.2 ± 6.0 | −4.2 ± 5.4 | <0.001 ∗∗∗ | 80.2 ± 5.2 | 83.0 ± 5.4 | −3.7 ± 7.0 | <0.001 ∗∗∗ |
MMFHC (°) | 90.5 ± 3.1 | 84.6 ± 2.6 | 6.4 ± 4.6 | <0.001 ∗∗∗ | 90.6 ± 3.5 | 84.7 ± 3.1 | 6.4 ± 5.3 | <0.001 ∗∗∗ | 89.4 ± 3.1 | 86.1 ± 3.1 | 3.6 ± 4.7 | <0.001 ∗∗∗ |
Variable | T0 | T1 | T2 | |||||||||
---|---|---|---|---|---|---|---|---|---|---|---|---|
Deviated | Nondeviated | Difference, % | P value | Deviated | Nondeviated | Difference, % | P value | Deviated | Nondeviated | Difference, % | P value | |
Volume (cm³) | 22.1 ± 5.0 | 22.0 ± 5.2 | 0.2 ± 11.1 | 0.728 | 20.7 ± 5.2 | 20.6 ± 5.0 | 0.1 ± 7.9 | 0.579 | 19.8 ± 4.5 | 19.6 ± 4.5 | 0.2 ± 9.2 | 0.493 |
Major axis length (mm) | 66.5 ± 5.1 | 66.8 ± 4.9 | −0.5 ± 3.5 | 0.423 | 66.8 ± 5.4 | 66.7 ± 4.8 | 0.2 ± 3.9 | 0.575 | 64.5 ± 5.4 | 64.0 ± 4.8 | 0.6 ± 3.8 | 0.137 |
CSAM (cm 2 ) | 4.5 ± 0.8 | 4.5 ± 0.8 | 0.0 ± 10.2 | 0.687 | 4.3 ± 0.8 | 4.2 ± 0.8 | 0.2 ± 8.5 | 0.873 | 4.3 ± 0.7 | 4.3 ± 0.8 | 0.4 ± 11.2 | 0.443 |
CSAF (cm 2 ) | 4.2 ± 0.8 | 4.2 ± 0.8 | 0.3 ± 10.4 | 0.421 | 3.9 ± 0.8 | 3.9 ± 0.8 | 0.7 ± 8.7 | 0.871 | 3.9 ± 0.7 | 3.9 ± 0.7 | −0.7 ± 10.5 | 0.975 |
MMFHS (°) | 80.9 ± 4.9 | 81.5 ± 5.3 | −0.8 ± 3.5 | 0.105 | 80.0 ± 5.7 | 80.5 ± 5.6 | −0.7 ± 3.9 | 0.238 | 81.3 ± 5.6 | 80.9 ± 5.2 | 0.3 ± 5.1 | 0.474 |
MMFHC (°) | 87.7 ± 2.0 | 87.2 ± 2.4 | 0.6 ± 2.5 | 0.062 | 87.7 ± 2.2 | 87.1 ± 2.4 | 0.6 ± 3.0 | 0.117 | 88.0 ± 2.8 | 88.1 ± 2.8 | −0.1 ± 3.8 | 0.975 |
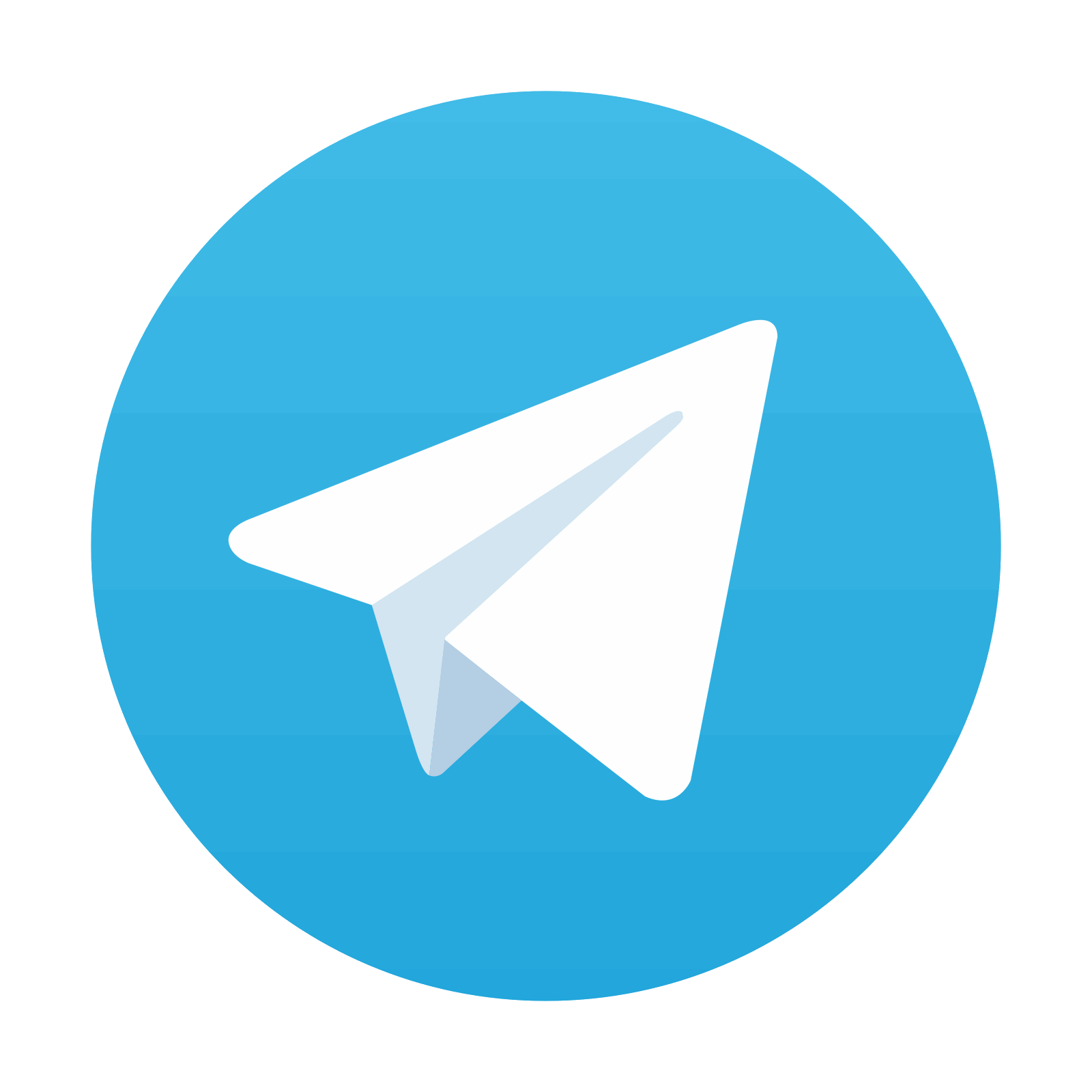
Stay updated, free articles. Join our Telegram channel

Full access? Get Clinical Tree
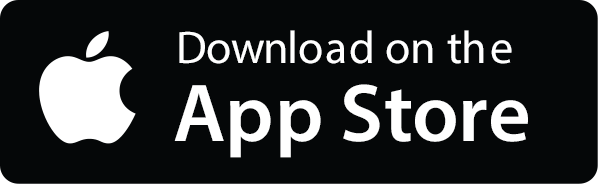
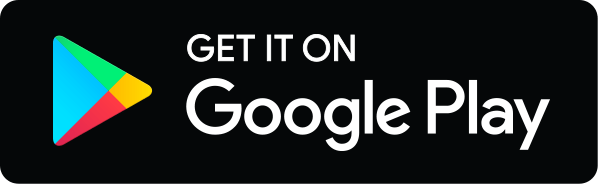
