Cervical Trauma
Khalid M. I. Salem
Charles G. Fisher
Introduction
Cervical spine injury is common. There is a bimodal distribution with one peak observed among the young (15 to 24 years), in whom it usually follows high-energy trauma, and a second peak among patients above the age of 55 years who largely sustain the injury as a result of low energy trauma. The challenges in diagnosis and determination of stability require an understanding of relevant anatomy and a working knowledge of injury classifications to avoid catastrophic consequences. Advances in pre-hospital care have improved the survivorship of the more severely injured, thus increasing the burden of responsibility on health professionals managing such cases.
Anatomic Considerations
In consideration of traumatic injuries, the cervical spine can be divided anatomically into the upper cervical spine (from the base of the skull to C2) and the subaxial spine (C3–C7). The upper cervical spine has unique anatomic characteristics that allow for approximately 60% of the cervical spine rotation (about 80 degrees), 40% of the flexion-extension motion (about 40 degrees), and 45% of the overall neck motion. This relative freedom is enabled by ligamentous structures with minimal contribution from intrinsic bony stability.
Ligamentous stabilizers in the upper cervical spine include the two alar ligaments that arise along the medial aspect of the occipital condyles and lateral masses of C1 and insert onto the lateral aspect of the odontoid. Alar ligaments act as a primary restraint to rotation, as each would tighten with rotation to the contralateral side, and also serve as a secondary restraint in flexion and lateral bend but play no role in extension. Other stabilizers include the apical ligament which connects the tip of the dens to the anterior edge of the foramen magnum and the posterior longitudinal ligament (PLL) which extends superiorly as the tectorial membrane (Fig. 4.1). The tectorial membrane attaches to the base of the skull and acts together with the cephalad extension of the anterior longitudinal ligament (termed the atlantooccipital membrane) to sandwich the alar and apical ligaments and effectively limit occipitocervical junction (OCJ) distraction, flexion, and extension.
The primary stabilizer of the atlantoaxial (C1–C2) motion segment is the cruciate ligament complex. This structure contains the transverse atlantal ligament (TAL). The TAL crosses the waist of the odontoid peg, effectively limiting translation at C1/C2 with flexion, thereby protecting the spinal cord from compression while allowing rotation. This arrangement of the principal ligaments allows the atlas to function as an intercalated segment that in turn permits coupled, multiplanar movement.
The subaxial cervical spine is responsible for most of the sagittal plane movement in the neck (about 15 degrees/segment). Coronal movement in this section is about 5 to 10 degrees/segment. The spinous processes are classically short and bifid; thus, some superficial muscles (e.g., trapezius and splenius capitis) attach to the nuchal ligament instead of directly to bone. The “vertebra prominens,” or C7 spinous process, is distinctively long, not bifid, and nearly horizontal and ends in a tubercle on which the nuchal ligament is attached. Importantly, the spinous process of T1 or C6 is more palpable than C7 in 30% of the cases, and thus cannot be used by itself to confirm levels. Tension bands formed anteriorly by the anterior longitudinal ligament and the annulus fibrosus and posteriorly by the supraspinous, interspinous ligaments and the ligamentum flavum serve to resist hyperextension and hyperflexion, respectively.
Of note, the cross-sectional area of the spinal canal is largest at C2 and smallest at C7. Therefore, spinal cord damage is more frequently observed with lower cervical trauma.
Clinical Evaluation
Trauma patients should be managed using standardized advanced trauma and life support (ATLS) protocols. It is important to keep in mind that the head/torso ratio in the pediatric patient is disproportionately high; during immobilization this should be respected. In these patients, an occipital recess should be used
or alternatively the torso should be elevated with padding. In patients with an ankylosed spine (such as with ankylosing spondylitis, diffuse idiopathic skeletal hyperostosis, or extensive ossification of the posterior longitudinal ligament) cervical immobilization should take into account the pre-injury deformity, which is often kyphotic.
or alternatively the torso should be elevated with padding. In patients with an ankylosed spine (such as with ankylosing spondylitis, diffuse idiopathic skeletal hyperostosis, or extensive ossification of the posterior longitudinal ligament) cervical immobilization should take into account the pre-injury deformity, which is often kyphotic.
![]() Figure 4.1 Sagittal T2-weighted MR image of a patient with a traumatic occipitocervical dissociation that demonstrates a disrupted tectorial membrane (arrow). |
The neurologic examination should be documented, repeated, and structured to allow the documentation of a baseline and identify the deterioration early. The American Spinal Injury Association (ASIA) score provides an excellent tool for monitoring the neurologic status and is highly recommended. Additionally, cranial nerve examination (particularly the abducens and hypoglossal nerves) should be considered part of the assessment in patients reviewed for possible craniocervical injuries, which should be ruled out following high-energy trauma. A thorough secondary survey should also be completed as patients with cervical spine injury have an associated extraspinal injury in approximately 50% of the cases and a noncontiguous spinal injury in 15% of the case.
A number of clinical pictures for incomplete spinal cord injury have been described (Table 4.1). The unique anatomy of the spinal tracts at the brain stem/spinal cord junction results in an additional number of unusual forms of incomplete spinal cord injury described as cervico-medullary syndromes. These include Bell cruciate paralysis and Wallenberg hemiplegia cruciata. Bell cruciate paralysis presents with a picture similar to central cord syndrome but affects the upper limbs proximally more than distally. Wallenberg hemiplegia cruciata (lateral medullary syndrome or Wallenberg syndrome) results from lateral medulla and inferior cerebellum ischemia secondary to a posterior inferior cerebellar artery (PICA) obstruction. The syndrome results in a myriad of symptoms including dysphagia, ipsilateral facial numbness with contralateral trunk and extremity numbness, ipsilateral cerebellar findings, vertigo, and an ipsilateral Horner syndrome.
TABLE 4.1 TYPES OF INCOMPLETE SPINAL CORD INJURIES | |||||||||||||||
---|---|---|---|---|---|---|---|---|---|---|---|---|---|---|---|
|
Imaging
Computed tomography (CT) is now considered the initial investigation of choice for the traumatized cervical spine. It allows for excellent assessment of the bony anatomy, alignment, and anterior cervical soft tissue swelling. CT also allows for clear visualization of junctional cervical areas (OCJ and cervicothoracic junction [CTJ]) making it more sensitive, efficient, and cost-effective than plain radiography in the multi-traumatized patient.
Standard plain radiographic evaluation involves anteroposterior, lateral, and open mouth views. An additional swimmer’s view is obtained if the CTJ was not adequately visualized. The National Emergency X-Radiography Utilization Study (NEXUS) criteria and the Canadian C-Spine rule are both highly sensitive tools and can be used to aid the decision to image patients or proceed to clinical clearance of the cervical spine.
Adequate lateral radiographs directly identify 85% of the injuries. Indirect signs of injury on a lateral radiograph include widening of the soft tissue shadow anterior to the spinal column (normal is <10 mm at C1, <7 mm at C3, and <20 mm at C6). A power ratio >1 signifies an OCJ injury (calculated as the ratio of a line drawn from the basion to the posterior arch of the atlas over a line from the opisthion to the anterior arch of the atlas) (Fig. 4.2). An atlanto-dens interval (ADI) >3 mm in adults and >5 mm in children suggests, in the least, a ruptured TAL. In the subaxial spine, flexion injuries associated with >11 degrees of segmental angulation or 3.5 mm translation compared to an adjacent level are suggestive of mechanical instability. On the other hand, the presence of significant anterior soft tissue swelling might be the only indication of an unstable injury. A number of additional radiographic alignment lines and measurements have been described particularly for the OCJ but have been largely supplanted by CT and MRI imaging.
Magnetic resonance imaging (MRI) is very sensitive in identifying soft tissue injuries (disk and ligaments) and neural element injury; the latter carries an additional prognostic value. MRI is recommended in patients who present with a neurologic deficit or have experienced deterioration in their neurologic status and in patients with suspected disk and ligament disruption. MRI has moderate specificity in characterizing posterior ligamentous complex injuries. In a meta-analysis of 1,550 patients with a normal cervical spine CT scan following blunt trauma, MRI identified a clinically significant injury that altered treatment in 6% of the cases (5% needing continued collar immobilization while 1% required surgical stabilization).
There is merit in obtaining both a CT and an MRI scan for definitive clearance in all cervical spine trauma cases. Advocates argue that significant injuries might be missed as it can anatomically reduce in a well-applied hard collar. On the other hand, MRI scan costs, the logistics of patient transport, positioning in the scanner and access to the patient during the study, coupled with the low incidence of a clinically significant injuries missed on CT, are other factors to be considered. In the conscious, neurologically intact trauma patient with a normal CT scan, it has been our practice to obtain additional up-right cervical radiographs out of the collar. If the radiographs are both adequate and negative, the collar can be safely discontinued.
Craniocervical Injuries and their Treatment
Occipital Condyle Fractures
Occipital condyle fractures are being detected more frequently because of CT trauma screening. The fracture is commonly the result of a high-energy injury and can be associated with a noncontiguous spine fracture (30%) or an extraspinal injury (85%). The hypoglossal nerve is particularly at risk as it exits the base of the skull through the hypoglossal canal. Based on the mechanism of injury, Anderson and Montesano classified the injury into axial load injures including condyle impaction (type I) or a condyle fracture with an associated skull base fracture (type II) while rotational or lateral bend forces present as alar ligament avulsion fractures (type III).
Stability dictates the management plan. Evidence of malalignment or significant ligamentous disruption on MRI is an indication for surgical stabilization. In the absence of these features, injuries can be managed with rigid cervical orthosis for 6 to 8 weeks.
Occipitocervical Instability/Dissociation
Injuries to the OCJ carry significant morbidity and mortality. They are the result of high-energy trauma. Instability can be classified based on the direction of displacement between the occiput and C1:
Anterior displacement (type I) which is more common in children due to the disproportional head size
Vertical displacement (type IIa) or at C1/C2 (type IIb)
Posterior displacement (type III)
Early identification is crucial to outcome. The power ratio is a helpful radiologic indicator, however, other measurements are more predictive. A basion-dens interval (BDI) or basion–posterior axial intervals (BAI), best measured on a midsagittal CT reconstruction, greater
than 12 mm is highly suggestive of occipitocervical dissociation (also known as the Harris rule) (Fig. 4.3). An MRI will help identify occult ligamentous injuries, which can have normal measurements on CT images.
than 12 mm is highly suggestive of occipitocervical dissociation (also known as the Harris rule) (Fig. 4.3). An MRI will help identify occult ligamentous injuries, which can have normal measurements on CT images.
In situations in which alignment is normal on static imaging (radiographs and CT scan) but the MRI signal is abnormal, provocative traction has been suggested to identify occult instability. This must be done extremely carefully. Distraction >2 mm with traction is suggestive of instability.
Once OCJ instability is diagnosed, strict precautions should be maintained until surgical fixation can be performed. Halo immobilization may be used to provide temporary, provisional stability while definitive treatment is planned for patients who are too ill to undergo immediate surgery.
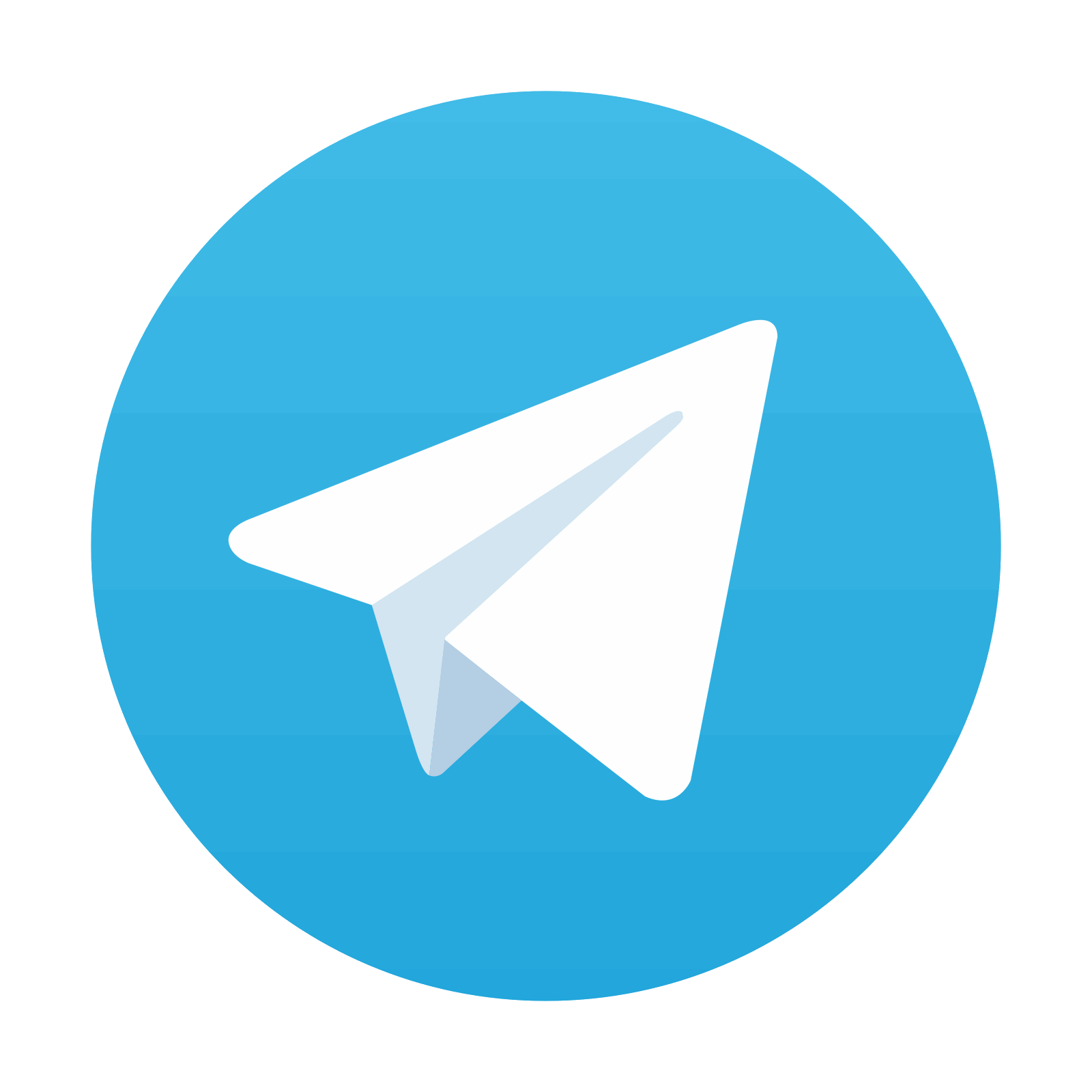
Stay updated, free articles. Join our Telegram channel

Full access? Get Clinical Tree
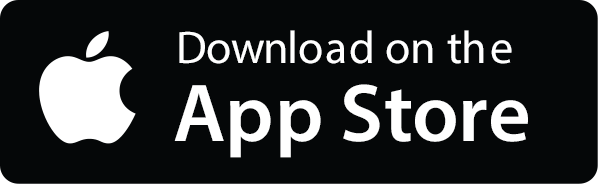
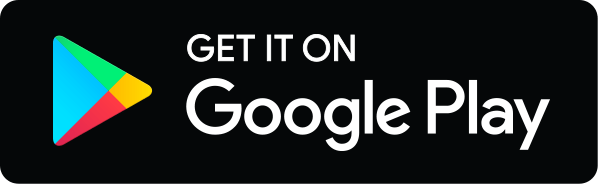