Cervical Cord Neurapraxia
Frank H. Valone III, MD
K. Daniel Riew, MD
Dr. Riew or an immediate family member has received royalties from Biomet and Medtronic; is a member of a speakers’ bureau or has made paid presentations on behalf of AOSpine, NASS; has stock or stock options held in Amedica, Benvenue, Expanding Orthopedics, Nexgen Spine, Osprey, Paradigm Spine, Spinal Kinetics, Spineology, and Vertiflex; has received research or institutional support from Cerapedics and Medtronic Sofamor Danek; has received nonincome support (such as equipment or services), commercially derived honoraria, or other non-research–related funding (such as paid travel) from Broadwater; and serves as a board member, owner, officer, or committee member of AOSpine and the Global Spine Journal. Neither Dr. Valone III nor any immediate family member has received anything of value from or has stock or stock options held in a commercial company or institution related directly or indirectly to the subject of this article.
Background
Cervical cord neurapraxia (CCN) is a transient neurologic deficit that occurs after cervical cord trauma in the absence of instability or structural deficiency of the cervical spine. CCN occurs at a rate of 1.3 to 6 per 10,000 athletes.1 The highest rates of CCN have been found in football players, in whom it is estimated to be as high as 7.3 per 10,000 participants.2 Torg and coworkers developed the classification system for CCN as defined by the type of neurologic deficit: type 1, “plegia” for episodes with complete paralysis; type 2, “paresis” for episodes with motor weakness; and type 3, “paresthesia” for episodes that involve only sensory changes without motor involvement. CCN is further classified by grade according to the duration of symptoms: grade 1 (<15 minutes), grade II (15 minutes–24 hours), and grade III (>24 hours). Last, CCN can be defined by anatomic distribution of the neurologic symptoms: “quad” for episodes involving all four extremities, “upper” for episodes involving both arms, “lower” for episodes involving both legs, and “hemi” for episodes involving an ipsilateral arm and leg.2,3
Symptoms
In Torg et al’s case series of 110 adult patients, the incidence of plegia was 40%, the incidence of paresis was 25%, and the incidence of paresthesia was 35%. The majority of CCN episodes were grade 1 (74%), resolving within 15 minutes, with grade 2 (15%) and grade III (11%) occurring less frequently. Additionally, the CCN pattern was quad extremity involvement in the majority of cases (80%), upper extremity in 15%, lower in 2%, and hemi in 3%.1 Neck pain and loss of cervical range of motion (ROM) are not frequently experienced in adults at the time of the injury.4 This is in contrast to children, in whom the deficits were most commonly upper extremity paresis (38%), quadriparesis (31%), hemiparesis (23%), and lower extremity paresis (8%). Additionally, the mean duration of symptoms in children was 26 hours, lasting as long as 5 days in one case, and 77% of pediatric patients experienced neck pain and decreased cervical ROM at the time of their injury.5
Mechanism of Injury
The mechanism of injury in CCN involves hyperflexion, hyperextension, or an axial load to the cervical spine causing a temporary derangement in the axonal permeability of the spinal cord.6,7 In 1962, Penning described the pincer mechanism for cervical spinal cord compression. In extension, the spinal cord becomes pinched between the posterior inferior aspect of the superior vertebral body and the anterior superior aspect of the inferior lamina. Conversely, in flexion, the cervical spine becomes compressed between the lamina of the superior vertebrae and the posterior superior aspect of the inferior vertebral body.7,8
Laboratory studies were conducted to quantify these theories. In a system designed to apply uniaxial tension at high strain rates with varying degrees of stretch, Torg and coworkers tested the giant squid axon of the squid Loligo pealei. The membrane potential and the cytosolic free calcium concentrations were recorded throughout the experiment. The study demonstrated that rapid stretch
resulted in calcium influx. The rise in the calcium concentration was directly proportional to the rate and amount of tension applied to the axon. The calcium influx caused hyperpolarization followed by a prolonged period of depolarization. During this depolarization period, the axon was no longer excitable. Neurologic recovery was inversely proportional to the rise in the calcium concentration. Additionally, this study concluded that local anoxia due to venous spasm did not delay recovery because of the maintenance of the cellular and structural anatomy in the cervical spine.7
resulted in calcium influx. The rise in the calcium concentration was directly proportional to the rate and amount of tension applied to the axon. The calcium influx caused hyperpolarization followed by a prolonged period of depolarization. During this depolarization period, the axon was no longer excitable. Neurologic recovery was inversely proportional to the rise in the calcium concentration. Additionally, this study concluded that local anoxia due to venous spasm did not delay recovery because of the maintenance of the cellular and structural anatomy in the cervical spine.7
Risk Factors for Cervical Cord Neurapraxia
A large epidemiologic study was completed to evaluate the risk factors associated with CCN. The study had five cohorts: cohort 1 (n = 227), college football players who did not have a history of CCN; cohort 2 (n = 97), professional football players who did not have a history of CCN; cohort 3 (n = 45), high school, college, and professional football players with at least one episode of CCN; cohort 4 (n = 75), individuals with permanent quadriplegia after a football cohort; and cohort 5 (n = 105), a control group of nonathletes without a history of CCN. Cohort 3 had a significantly smaller ratio of diameter of spinal canal to vertebral body (Torg ratio) (Figure 11-1A) (P <0.05; C3 ratios: cohort 1, 0.873 + 0.115 mm; cohort 2, 0.849 ± 0.116 mm; cohort 3: 0.732 ± 0.156 mm; cohort 4, 0.958 ± 0.115 mm; and cohort 5, 0.998 ± 0.123). Additionally, cohort 3 had a significantly smaller mean diameter of the cervical spinal canal (Figure 11-1B) (P <0.05; C3 diameter of spinal canal: cohort 1, 18.789 ± 2.031 mm; cohort 2, 18.474 ± 2.323 mm; cohort 3, 15.556 ± 2.704 mm; cohort 4, 19.093 ± 2.188 mm; and cohort 5, 19.025 ± 1.883 mm). The results of this study demonstrated that symptomatic athletes had significantly smaller spinal canals, suggesting an association between stenosis and neurapraxia.9
![]() FIGURE 11-1 A, Torg ratio: ratio of the spinal canal diameter to the vertebral body diameter between cohorts. B, Spinal canal diameter (in millimeters) among cohorts. |
Spinal Stenosis
Cervical spinal stenosis is common in pediatric and adult athletes, with the prevalence in football players reported between 7.6 and 29 cases per 100 players.9,10 In an evaluation of 104 plain radiographs in adult patients who had experienced an episode of CCN, only 7% had normal findings, and 86% had cervical stenosis, 50% had osteophytic ridging, 21% had loss of cervical lordosis, and 28% had degenerative disk disease. Additionally when MRIs were evaluated in this same group of patients, only 8% of patients had normal findings, but 81% had evidence of disk bulging, 36% had disk protrusion, 55% had osteophytic ridging, and 47% had neuroforaminal compromise.2 None of the MRIs in this study, nor Maroon et al’s study of five professional athletes who had experienced CCN, demonstrated any posttraumatic cord swelling, deformity, or syrinx.2,3
Cervical spinal stenosis can be determined by a variety of techniques. In a study of 200 asymptomatic patients, Wolf and coworkers established normative values for the sagittal cervical spine. Measurements were taken from the posterior vertebral body to the site of fusion of the spinous process and laminae (spinolaminar line) (Figure 11-2). Wolf and coworkers found the average AP diameter was 22 mm at C1, 20 mm at C2, and 17 mm from C3 to C7. Consensus was that between C3 and C7, sagittal diameters larger than 15 mm were normal, and stenosis was found with sagittal diameters smaller than 13 mm.11 Similarly, a study of 1066 human cadaveric specimens from the Hamann-Todd collection at the Cleveland Museum of Natural History found an absolute sagittal value of smaller than 13 mm was strongly associated with cervical spinal stenosis at all levels.12
The Torg ratio is calculated as the ratio of the spinal canal diameter to the vertebral body diameter at the C3 to C7 levels as measured on a lateral plain radiograph (Figure 11-3). A Torg ratio of less than 0.8 is considered evidence of congenital stenosis. They concluded that the low positive predictive value of 0.2% precluded its use as a screening method for participation in contact sports. However, because this method determines a ratio rather than absolute numbers, it is independent of magnification factors caused by differences in target distance and object to field distance.6 A criticism of this technique is that it does not take into account disproportionate differences in vertebral body size in some patient populations. Herzog and associates demonstrated that football players commonly have larger vertebral bodies relative to other spinal element, and found abnormal Torg ratios in 49% of professional football players they studied.13 Additionally, Odor and coworkers observed that one third of asymptomatic professional football players have a Torg ratio of less than 0.8 at one or more levels.14
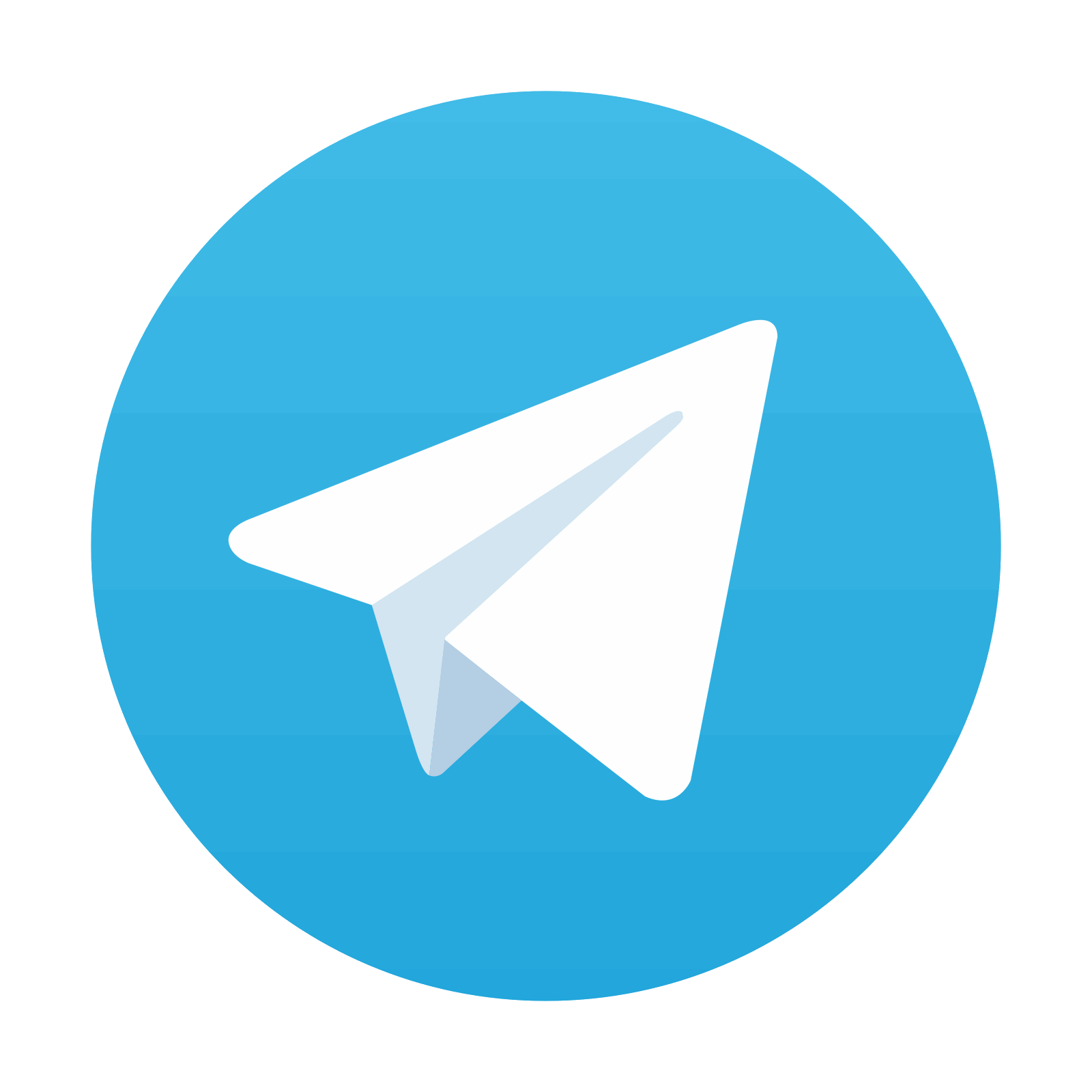
Stay updated, free articles. Join our Telegram channel

Full access? Get Clinical Tree
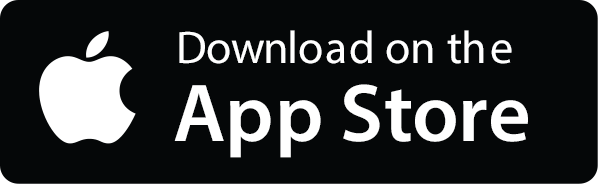
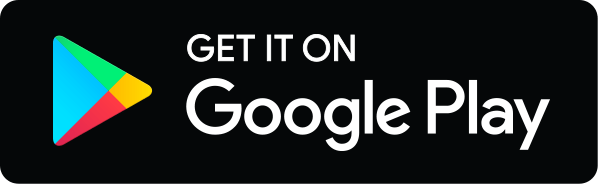