Abstract
Articular cartilage is a non-vascularized and poorly cellularized connective tissue that is frequently damaged as a result of trauma and degenerative joint diseases such as osteoarthrtis. Because of the absence of vascularization, articular cartilage has low capacity for spontaneous repair. Today, and despite a large number of preclinical data, no therapy capable of restoring the healthy structure and function of damaged articular cartilage is clinically available. Tissue-engineering strategies involving the combination of cells, scaffolding biomaterials and bioactive agents have been of interest notably for the repair of damaged articular cartilage. During the last 30 years, cartilage tissue engineering has evolved from the treatment of focal lesions of articular cartilage to the development of strategies targeting the osteoarthritis process. In this review, we focus on the different aspects of tissue engineering applied to cartilage engineering. We first discuss cells, biomaterials and biological or environmental factors instrumental to the development of cartilage tissue engineering, then review the potential development of cartilage engineering strategies targeting new emerging pathogenic mechanisms of osteoarthritis.
1
Introduction
Articular cartilage may undergo many alterations due to inflammatory causes, trauma, or aging. These complications lead to decreased cellularity and further degradation of the cartilage extracellular matrix (ECM). Cartilage damage has been known for more than 200 years. In 1743, the Scottish doctor William Hunter wrote in an article of the Royal Society, “Since Hippocrates and until now, it is universally accepted that the ulcerated cartilage is embarrassing and that, once destroyed, can not be repaired”. The absence of vascularization and proliferation of chondrocytes provides articular cartilage with poor self-healing capacity. The cartilage damage and associated catabolic processes are irreversible and lead to long-term development of osteoarthritis (OA).
OA is the most common joint disorder affecting a growing part of the aging population. The incidence of this degenerative disease increases with age and is a major health concern. Worldwide, more than 10% of men and 18% of women older than 60 years is estimated to be affected by OA. OA is accompanied by degradation of the articular cartilage, thickening of the subchondral bone, formation of osteophytes and variable degrees of synovium inflammation . In this context, the scientific community has been interested for many years in different strategies to regenerate a functional cartilaginous tissue. However, surgical techniques developed in an attempt to repair cartilage damage, such as the abrasive chondroplasty, microfracture and spongialisation , lead to the formation of transient fibrocartilaginous tissue. In parallel, transplantation of tissue with chondrogenic properties, such as periosteum and perichondrium transplants and osteochondral grafts (mosaicplasty), have been proposed.
Still today and despite advances in orthopedic surgery, treatment of the cartilage damage remains challenging. The existence of numerous limitations associated with the above-mentioned techniques such as graft instability, calcification, and restricted applications to focal lesions have led to the development of new therapeutic strategies based on cell therapy and tissue engineering. Tissue engineering, a field of research that emerged during the 1990s , was defined by Langer and Vacanti in 1993 as “an interdisciplinary field that applies the principles of engineering and the life sciences toward the development of biological substitutes that restore, maintain, or improve tissue function” .
In this review, we focus on the different components of tissue engineering applied for cartilage engineering. We discuss the promising development of cartilage engineering strategies targeting recently identified pathogenic mechanisms of OA.
2
Articular cartilage tissue engineering
Tissue engineering is an emerging discipline that combines the principles of engineering and biological sciences to develop biological substitutes that can notably restore the functions of altered tissues . This discipline is based on an association of biomaterials, cells and biological or environmental factors, also known as the “tissue engineering triad” ( Fig. 1 ). Cartilage tissue engineering has greatly benefited from recent advances in material engineering and in our understanding of the role of growth factors and stem cells in tissue regeneration.
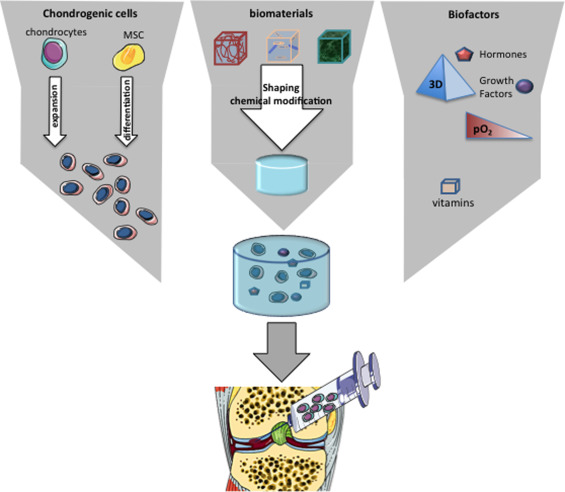
2.1
Biomaterials
In tissue engineering, cells are generally seeded onto a scaffold, whose primary objective is to replicate the characteristics of the target-tissue ECM. By mimicking the ECM, biomaterials provide cells with an environmental structure able to support cell viability, proliferation and secretory activities. Many ECM-like scaffolds are available and have been considered for cartilage tissue engineering ( Table 1 ). These biomaterials can be classified as synthetic or natural matrices, in which we can distinguish those based on proteins or polysaccharides . The ideal biomaterial should be biocompatible to prevent inflammatory and immunological reactions. It must provide a favorable environment for the 3D maintenance of chondrocyte phenotype and be adhesive to enable attachment of the cells within the lesion. It must be permeable to allow the diffusion of molecules, nutrients and growth factors. Finally, it should be biodegradable enough to be integrated in the physiological processes of tissue remodeling and ideally, should be injectable, thereby allowing for implantation by minimally invasive surgery.
Matrices | ||
---|---|---|
Type | Component | Commercial product name |
Protein | Collagen | MACI ® , Maix ® , Atelocollagen ® , MaioRegen ® |
Fibrin | Tissucol kit ® | |
Silk | ||
Polysaccharides | Hyaluronic acid | HYAFF-11 ® |
Chitosan | BST-CarGel ® | |
Cellulose | ||
Alginate | ||
Synthetic | Poly(lactic-co-glycolic acid) | Bio-Seed ® -C |
Polylactic acid | ||
Polyethylene glycol |
Among the protein-based matrices, membranes of type I and III collagen are clinically used in the autologous chondrocyte transplantation kits Matrix associated chondrocyte implantation (MACI ® ; Verigen, Leverkusen, Germany) , Maix ® (Matricel, Hezoenrath, Germany) and Chondro-Gide ® (Geistlich Biomaterials, Wolhusen, Switzerland). A type I collagen gel (Atelocollagen ® , Koken Co., Tokyo) has been used for the 3D culture and in vivo implantation of human autologous chondrocytes and bone marrow-derived mesenchymal stem cells (MSCs) in case studies.
Among the polysaccharide-based matrices, Hyalograft ® C consists of autologous chondrocytes associated with a hyaluronic acid matrix named HYAFF-11 ® (Fidia Advanced Biopolymers, Abano Terme, Italy). This concept has led to clinical improvements in articular cartilage in humans . However, because of an unfavorable review of the European Medicines Agency citing an overall lack of safety and efficacy data from well-controlled studies, Hyalograft ® C is no longer available. BST-CarGel ® (Piramal Healthcare, Aurora, ON, Canada) is a scaffold made of chitosan and β-glycerophosphate that was developed for use as part of the MACI procedure. The BST-CarGel ® procedure significantly improved clinical outcomes but only modestly improved patient quality of life .
Bio-Seed ® -C (BioTissue Technologies, Freiburg, Germany) belongs to synthetic matrices; it is a porous biomaterial based on polyglycolic acid (PGA), polylactic acid (PLA) and polydioxanone, on which chondrocytes suspended in fibrin glue are deposited . BioSeed ® -C enables the formation of hyaline cartilage and leads to clinical improvement in joint function. The use of biphasic biomaterials has become fashionable. These bilayered scaffolds consist of 2 different materials: a lower ceramic-like layer, which can be fitted as a plug into the subchondral bone, and an upper one intended for the cartilaginous compartment of osteochondral defects. Different combinations of ceramic, synthetic polymers and natural materials such as collagen or hydroxyapatite in bilayered scaffolds have been investigated in clinical trials ). MaioRegen ® (Finceramica, Italy) is a bioceramic multi-layered scaffold in a single gradient structure consisting of deantigenated type I equine collagen and magnesium enriched-hydroxyapatite . The product has shown promising initial results that remains to be supported by further studies.
Despite the large number of preclinical and clinical studies related to biomaterials for cartilage tissue engineering, none of the matrices discussed may be injected percutaneously. In this context, recent studies are directed toward the development of self-hardening and injectable materials that can be used in the percutaneous transplantation of chondrogenic cells. The properties and structures of hydrogels make them ideal candidates for implantation by minimally invasive surgery . Hydrogels are composed of chains of synthetic or natural macromolecules capable of forming hydrogels after physical, ionic or covalent crosslinking . They exhibit a high level of hydration close to that of the articular cartilage, which allows them to mimic the 3D environment of chondrocytes . Confirming this idea, we recently used a self-setting cellulose-based hydrogel to repair articular cartilage with chondrocytes and MSCs in a rabbit model of osteochondral defects .
In summary, the culture of phenotypically stable chondrocytes as well as the chondrogenic differentiation of MSCs was demonstrated with a number of different types of biomaterials ( Table 1 ). However, despite an abundant variety of scaffolds developed for cartilage engineering, only a few have been successfully transferred to the clinic .
2.1.1
Cells
Chondrocytes, fibroblasts, stem cells and genetically modified cells have all been tested in cartilage engineering. However, chondrocytes and MSCs remain the most largely investigated sources of chondrogenic cells for cartilage repair.
Adult chondrocytes having the ability to form an ECM can be isolated from various sources such as articular cartilage, nasal septum, costal cartilage or auricular cartilage . Nevertheless, auricular chondrocytes are derived from an elastic cartilaginous tissue, which does not have the mechanical properties of hyaline cartilage (joint, nasal septum). Isogai et al. showed that each type of chondrocyte gives rise to a tissue with the characteristics of the original one . In the context of articular cartilage engineering, hyaline cartilage will be the preferred source of chondrocytes. Comparison of the various sources of hyaline chondrocytes (nasal, costal and articular cartilages) has demonstrated the superiority of costal and nasal chondrocytes over articular chondrocytes in terms of the amount of cartilage formed in subcutaneous sites . However, only 2 procedures involving articular chondrocytes are marketed today. The first, Carticel ® (Vericel Corp.), is a procedure for isolation and in vitro amplification of autologous chondrocytes for use during autologous chondrocyte implantation; Carticel ® is indicated for the repair of cartilage defects of the femoral condyle but not for treatment of cartilage damage associated with generalized OA. The second procedure, ChondroCelect ® (Tigenix, Leuven, Belgium), is a method for isolation and in vitro amplification of autologous chondrocytes expressing specific markers. These chondrocytes are then used for autologous chondrocyte implantation. However, one of the main drawbacks in the use of autologous chondrocytes, whatever their origin, is the instability of their phenotype in monolayer culture. Indeed, chondrocytes cultured in monolayer rapidly lose their phenotype, as evidenced by the loss of expression of chondrocytic markers such as type II collagen, aggrecan and the superficial zone protein . This loss is accompanied by the orientation of cells toward a fibroblastic phenotype characterized by increased expression of type I collagen and adoption of the characteristic spindle shape of fibroblasts . Despite the reversibility of this phenomenon in 3D culture , this dedifferentiation process has motivated the search for alternative cells to chondrocytes for cartilage tissue engineering.
Adult MSCs are multipotent cells characterized by their ability to proliferate and differentiate. MSCs can give rise to several types of differentiated cells, including chondrocytes, adipocytes, osteoblasts, and myogenic and neuronal cells . The discovery of these cells has opened the way to new therapeutic strategies, in part because they are easily accessed. The most-studied source of MSCs is the bone marrow, but MSCs have also been isolated from muscles, adipose tissue, periosteum and perichondrium . In addition, MSCs isolated from bone marrow, adipose tissue, muscle and synovium showed chondrogenic differentiation ability. Consequently, adult MSCs have been considered of major interest in tissue engineering . However and despite a huge number of studies reporting the promising properties of MSCs for cartilage tissue engineering, only a few human clinical studies have reported the use of MSCs with or without biomaterials in a joint site. Overall, these studies have had encouraging results, with improved clinical signs in treated patients . Currently, 58 clinical studies involving MSCs for OA are referenced at ClinicalTrials.gov. The first results of these studies will help refine our knowledge of the efficacy of MSCs in repairing cartilage lesions. MSCs are also of major interest in tissue engineering because they generally do not express molecules of the class II major histocompatibility complex (MHC-II), responsible for immune rejection . This particularity seems to hint to an immunological privilege state of MSCs suggesting that they could be also used as allogeneic cells. In this context, the Korean Food and Drug Administration has recently approved Cartistem ® , an allogeneic umbilical cord-blood stem cell product for treating OA. The clinical results of Cartistem ® have not been reported. Currently, this immune privilege state is largely debated and needs to be clarified before using “off-the-shelf” MSC therapies.
Recently, the possibility of generating human induced pluripotent stem cells (hiPSCs) from somatic cells (i.e., cutaneous fibroblasts) reprogrammed by the expression of pluripotency-associated transcription factors Oct4, Sox2, Myc and Klf4 has been discovered. This breakthrough discovery, responsible for the 2012 Nobel Prize in Medicine or Physiology awarded to Sir John B. Gurdon and Shinya Yamanaka, is likely to pave the way for new therapeutic developments in regenerative medicine. In addition, the generation of functional chondrocytes from hiPSCs that was recently reported , is of particular interest because it provides a limitless source of patient-specific cells for the regeneration of articular cartilage. However, the efficacy and safety of hiPSC-derived chondrocytes for cartilage regeneration remains to be tested in relevant animal models of cartilage defects and OA. The possibility of producing large amounts of hiPSC-derived functional chondrocytes may catapult the field of cartilage engineering forward.
2.2
Biological and environmental factors
The formation of articular cartilage at the embryonic level follows a process closely related to endochondral ossification. Several growth factors involved in the development of cartilage growth plate were identified as factors deeply affecting the chondrogenic differentiation of MSCs. Among these factors, the transforming growth factor β (TGF-β) superfamily members TGF-β1 and -β3 are the most commonly used to induce the chondrogenesis of adult MSCs. Alternatively, the use of bone morphogenetic protein with or without TGF-β has been extensively investigated . Other growth or differentiation factors have been tested to modulate the chondrogenic differentiation of MSCs, with various effects .
MSCs can be induced or stimulated to chondrogenic differentiation by using specific environmental factors. Among these factors, 3D culture, low oxygen tension and mechanical stimuli are probably the most widely documented. Similar to chondrocytes, for which 3D culture allows the maintenance or recovery of their phenotype , the chondrogenic differentiation of MSCs is strongly promoted by 3D culture . High-density aggregation by centrifugation places cells in an in vitro 3D environment that closely mimics the cell condensation observed during in vivo embryonic development of cartilage . Of interest, the biomaterials we describe have been successfully investigated for the 3D culture and chondrogenic differentiation of MSCs .
Articular cartilage is avascular and develops naturally in the presence of a low oxygen tension (hypoxia) ranging from 2% to 7%. Furthermore, hypoxia can upregulate the chondrocytic phenotype by hypoxia inducible factors (HIFs), which promote the expression of cartilage ECM genes . Therefore, placing MSCs in a hypoxic condition can promote the chondrogenesis of MSCs . In addition, low oxygen tension has been reported to prevent the terminal hypertrophic conversion of MSCs . Hence, oxygen tension is undoubtably a safe and easily tunable factor for controlling the chondrogenic differentiation of MSCs.
Finally, considering that articular cartilage is a tissue subjected to various mechanical stimuli, including hydrostatic pressure and compressive or shear stress, the role of mechanical stimuli has been considered. Physiological loading is a pivotal factor affecting the chondrogenic differentiation of MSCs during articular cartilage development. In addition, mechanical stimuli applied to chondrocytes has been found essential for maintaining cartilage integrity. Accordingly, we have convincing reports that mechanical stimuli can be used for generating cartilage-engineered constructs with a structural organization of ECM components (i.e., collagen and glycosaminoglycan) closely resembling that of native cartilage .
2
Articular cartilage tissue engineering
Tissue engineering is an emerging discipline that combines the principles of engineering and biological sciences to develop biological substitutes that can notably restore the functions of altered tissues . This discipline is based on an association of biomaterials, cells and biological or environmental factors, also known as the “tissue engineering triad” ( Fig. 1 ). Cartilage tissue engineering has greatly benefited from recent advances in material engineering and in our understanding of the role of growth factors and stem cells in tissue regeneration.
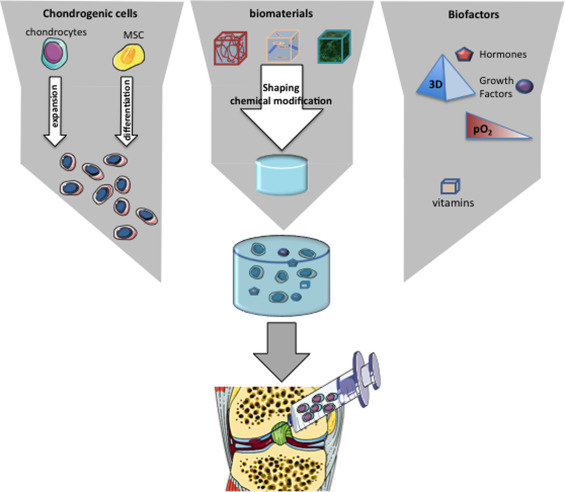
2.1
Biomaterials
In tissue engineering, cells are generally seeded onto a scaffold, whose primary objective is to replicate the characteristics of the target-tissue ECM. By mimicking the ECM, biomaterials provide cells with an environmental structure able to support cell viability, proliferation and secretory activities. Many ECM-like scaffolds are available and have been considered for cartilage tissue engineering ( Table 1 ). These biomaterials can be classified as synthetic or natural matrices, in which we can distinguish those based on proteins or polysaccharides . The ideal biomaterial should be biocompatible to prevent inflammatory and immunological reactions. It must provide a favorable environment for the 3D maintenance of chondrocyte phenotype and be adhesive to enable attachment of the cells within the lesion. It must be permeable to allow the diffusion of molecules, nutrients and growth factors. Finally, it should be biodegradable enough to be integrated in the physiological processes of tissue remodeling and ideally, should be injectable, thereby allowing for implantation by minimally invasive surgery.
