INTRODUCTION
Advances in bone graft substitutes hold the promise of revolutionizing surgical practice. Within the next decades, physicians may be able to offer therapies that provide early fracture healing for both operatively and nonoperatively treated fractures. With complex fractures, surgeons will be able to choose among graft substitutes that offer the potential for minimal fixation, accelerated union, earlier return to function, more independence, and less reliance on hand therapy. The term bone graft substitute will become increasingly inaccurate as the indications for these agents expand well beyond those conceived for traditional autograft or allograft.
An extensive variety of existing graft substitutes serve as gap fillers and can restore structural integrity. Newer synthetic agents actively stimulate bone healing, which has been best shown in animal studies. Expense and our own technologic limitations are such that routine use of biologically active agents in the extremities is restricted to circumstances in which inadequate healing potential exists. Patient demands and the potential economic benefit of using graft substitutes, together with advances in technology, are likely to lead to use of these materials more routinely. Currently worldwide, allografts or autografts are used in approximately 2.2 million orthopedic procedures annually. Although the future may hold much potential within the realm of bone graft substitutes, the importance of adequate fracture stabilization will never be surpassed.
At present, physicians can select from a wide variety of graft substitutes, ranging from allograft-based materials to wholly synthetic agents and from physiologic materials such as collagen and hydroxyapatite (HA) to foreign substances such as calcium sulfate and silicates. The high-value market for such materials has provided medical device companies with a major stimulus to create innovative and effective products. Bone grafting is approximately a $2.5 billion industry. Because corporate-sponsored research has focused on gaining regulatory approval for a wide variety of indications, there is an absence of comparative studies that demonstrate benefits of one agent over another.
This chapter identifies the major types of graft substitutes available or in development and reviews their unique features and capabilities. The indications and potential indications for graft substitutes in the distal radius are discussed, as well as the future directions of these graft substitutes.
CONFOUNDING VARIABLES AND CONFUSING NOMENCLATURE
Bone graft materials promise to augment fracture fixation, fill voids caused by significant comminution, and stimulate new bone formation. Clear indications for use are often lacking, despite the substantial growth in the industry in the past few years ( Fig. 24-1 ).

Limited clinical studies and scientific data, together with each company’s biased advertising claims, produce a confusing landscape of bone substitutes and proteins. The names of products from the time of development to the time to market often change, and frequently larger companies will have purchased the manufacturer before this occurs. Even more confounding is how to categorize and regulate materials. The U.S. Food and Drug Administration (FDA) loosely regulates allograft bone; the Center for Biologics Evaluation and Research of the FDA monitors the processing methods of human and animal bone and soft tissues, as well as carriers and extenders added to human tissue ( http://www.fda.gov/cber/tiss.htm ). Allograft components are the basis of most of the osteoconductive materials in use and are used widely in orthopedic procedures.
In the 1990s, the FDA loosely regulated bone by monitoring its processing, so human bone has not been subject to the rigors of demonstrating safety and efficacy, the two end points required by the FDA for new devices and pharmaceuticals. In May 2005, the FDA released three new comprehensive regulations that relate to manufacturing activities associated with human cells, tissues, and cellular/tissue-based products. The first regulation requires companies that produce and distribute these products to register with the FDA. The second regulation provides criteria that must be met for donor eligibility. The third regulation is referred to as the “Current Good Tissue Practices Rule” and governs the processing and distribution of tissues. More stringent regulation exists for biologic cements, monitored by the FDA’s Center for Devices and Radiological Health (CDRH, http://www.fda.gov/cdrh ). The Orthopaedic Panel of the CDRH reviews and regulates growth factors to date, as well as traditional internal fixation devices. In contrast, the FDA’s Center for Drug Evaluation and Research (CDER, http://www.fda.gov/cder ) reviews and regulates injectable proteins, whereas future genetically altered materials will be reviewed and regulated by the Center for Biologics Evaluation and Research ( http://www.fda.gov/cber/index.html ). Not only is the regulation of bone-related products confusing, the various arms of the FDA regulate similar products designated as devices, drugs, or biologic materials as discrete entities, rather than interrelated materials with shared properties. This represents a dilemma of how technology exceeds the knowledge, ethics, and indications for these novel materials. For the introduction of similar competitive products, FDA 510(k) approval is obtained. This approval requires demonstrating similar safety and efficacy without the rigors required for new device approval.
OVERVIEW OF CURRENT INDICATIONS
Trials of many graft substitutes have focused on the distal radius, since the classic osteoporotic fracture represents a common injury and a relatively homogeneous fracture pattern suitable for study. This fracture represents a classic bending fracture, with the presence of a displaced, comminuted dorsal radial cortex and with variations including involvement of the radiocarpal joint, radial styloid, and distal radioulnar joint (DRUJ). Stability and loss of reduction are of greater concern than healing of the fracture, since metaphyseal fractures rarely proceed to nonunion. Current methods of treatment are based on restoring the anatomy and achieving stability by different means, ranging from cast to invasive techniques depending on the specific pattern and patient factors.
Problems with any of the previously mentioned treatment methods do exist. Failure to restore normal wrist anatomy may correlate with poor function, but restored anatomy and prolonged immobilization may also accompany a wrist that is stiff and weak. Fixation in osteopenic and osteoporotic bone has its own dilemmas, including loss of reduction in simple fractures and hardware failure in complex ones. Healing, however, in this group fortunately is rarely an issue. Pain, loss of motion, and loss of strength often accompany fractures, especially high-energy injuries.
Autogenous bone grafting in distal radius fractures plays an important role in the more complex fractures of the distal radius. The benefits of autogenous graft include earlier removal of external fixation and improved anatomic and functional outcome. This type of graft is not without disadvantages, however, since comorbidity and hospitalization costs accompany iliac crest graft harvest ( Box 24-1 ) Such disparity has driven surgeons and entrepreneurs alike to seek better alternatives for fracture augmentation.
ADVANTAGES OF AUTOGRAFT
Osteoinductive and osteoconductive
Osteogenic
Available in cortical and cancellous forms
Provides structural support
Biocompatible
Incorporates into host site
Remodels to become normal bone
DISADVANTAGES OF AUTOGRAFT
Increased operative time, hospital stay, cost
Increased blood loss, postoperative pain, risk of infection, risk of fracture
Avascular bone: potential nidus for infection
Limited supply
Variable quality
BONE COMPOSITION AND BIOLOGIC ACTIVITY
Bone is an active milieu of mineral, protein, and cellular elements. The structural protein matrix and mineral provide a scaffold for new bone formation ( osteoconduction ). Blood-borne proteins, including growth factors and cytokines, stimulate and signal new bone growth ( osteoinduction ). Currently, mineral substitutes may confer osteoconduction, whereas allograft demineralized bone matrix is purported to exhibit osteoinductive capabilities. New bone formation, osteogenesis, as a discrete entity requires cellular activity. Bone substitutes to date are not osteogenic, since they lack the required cellular complement of osteoblasts, osteoclasts, and osteocytes. Osteogenic substitutes require the presence of cells in some milieu that favors osteoinduction and osteoconduction.
Hydroxyapatite is the most abundant form of calcium phosphate in the mineral phase of bone, with an optimal ratio of calcium:phosphate of 10:6, comprising the basic stoichiometric formula ( Fig. 24-2 ). Its weakly crystalline and unstable structure permits ionic exchange with carbon and the hydroxide ion and flux between different mineral precursors such as calcium phosphate and tricalcium phosphate (TCP). This instability allows bone activity and tolerance of bone substitutes. With the exception of calcium sulfate, these mineral substitutes are physiologic calcium salts. HA deposits on a matrix of type I collagen, where cellular activity occurs, to create the underlying latticework of mammalian bone.

Autograft bone has osteogenic potential because surviving bone cells and matrix proteins are present. Bone resorption is a common term reported for graft substitutes. This refers to the disappearance of bone, usually measured radiographically. Disappearance, however, may represent several possibilities. It may occur rapidly: by chemical dissolution, by physical disruption such as microfracture, or by phagocytosis, such as foreign body reaction. The slower process of bone remodeling may also account for resorption. True remodeling is a cellular activity of bone deposition and removal based on environmental and physiologic stresses. Since the distinction between dissolution and remodeling is rarely described, the surgeon must consider the remodeling versus dissolution effect when choosing a substitute. To complicate this topic, we traditionally invoke Wolff’s law of mechanical stresses to explain bone activity, but this relationship is poorly defined. Wolff’s original description in 1892 speaks of generalities in bone depositing according to lines of stress, but cellular and micro-environmental models to support Wolff’s law are still being defined. Furthermore, specific assays in humans that measure rate and intensity of biologic activity of bone, namely, osteoinduction or osteoconduction, do not exist.
BONE GRAFT SUBSTITUTES CURRENTLY AVAILABLE AND IN DEVELOPMENT
Numerous osteoconductive and osteoinductive materials, as well as synthetic cements, are available for the clinician or are in development. An overview of the available types is provided, followed by examples of currently marketed materials and materials in development.
Osteoconductive Materials
Most available osteoconductive materials are mineral-based, with some complement of the HA backbone. These may be generated from allograft human bone, xenograft bone (usually bovine), sea coral, or synthetically manufactured. Blocks and granules of coral or synthetic minerals and synthetic injectable cements are the major mineral formulations. Collagen-based materials also exist as both a framework and a carrier matrix for other substances. To date, collagen used in humans is bovine-derived.
Osteoinductive Materials
Demineralized bone matrix (DBM) is the most popular form of what is purported to be osteoinductive bone. It is the collagen matrix of allograft bone that remains after extensive processing that removes blood, cells, and minerals. Available in a variety of forms, its carrier material provides texture and pliability to suit the recipient site and is used primarily as a graft extender. Whether demineralized bone matrix is truly osteoinductive in humans has not been established.
Autogenous materials range from the readily obtainable bone marrow aspirate to cancellous and corticocancellous bone harvest. These have osteoinductive and osteoconductive, and potentially osteogenic, capabilities.
EXAMPLES OF SUBSTITUTES
Allograft Bone
Since allograft bone represents the spectrum of osteoinductive and osteoconductive materials, it is treated here as a separate group. Allograft bone in its various forms is commonly used in the distal radius and upper limb. It may be considered for patients with poor healing potential, highly comminuted fractures with a significant bone defect, fractures in patients with osteopenia or osteoporosis, and nonunion. Banked human bone is available in different forms. Bone that retains its morphology ranges from fresh and frozen, which have stronger mechanical properties but greater infection and rejection potential, to freeze-dried, which is weaker but with potentially less toxicity. Corticocancellous allograft is classically used for tumors, large defects from nonunions such as in the humerus, and areas of large defects. Cancellous allograft chips are used more commonly for smaller nonunions and metaphyseal voids, either as an autograft extender or replacement. Disadvantages of allograft bone include that it lacks the rich osteoinduction potential seen with autograft, that the quality of bone is variable and usually unknown to the surgeon before surgery, and that it carries a theoretical risk of disease transmission. Only recently has disease transmission been reported; this more likely represents increased surveillance and centralized database collection than first-time occurrences.
By 2002, the Centers for Disease Control and Prevention (CDC) had reported 26 cases of bacterial infections associated with musculoskeletal tissue allografts. Most infections involved a Clostridium organism, potentially related to the relative decomposition of the donor. All tissues had been processed aseptically but did not undergo terminal sterilization, a process that minimizes disease transmission but also sacrifices biologic incorporation. Four documented cases of HIV transmission from allograft are documented on the CDC website, and the FDA has cited tissue banks when bacterial or viral contamination has been identified in harvested human tissue. The disconcerting news of contamination of specimens, as well as recipient infection and death, supports the quest for developing synthetic materials that lack potential disease transmission.
Demineralized Bone Matrix
DBM is allograft bone treated with acid extraction and therefore theoretically contains noncollagenous proteins, which serve as the osteoconductive scaffold for bone ingrowth as well as osteoinductive growth factors. The processing, which includes chemical and radiation treatment, weakens the bone and its bone formation potential. Growth factors may survive processing; although their actual presence is not documented in the literature. DBM confers osteoinduction in an animal model and therefore suggests the presence of factors. An FDA document that discusses how DBM confers osteoinduction has been sufficient evidence for companies to purport its capabilities, however unsubstantiated. Dry, moldable, or injectable forms exist as powder, granules, gel, paste, or putty, using a carrier that renders DBM suitable for placement. The safest and best carrier agent remains to be identified. Carriers include hyaluronic acid, collagen, glycerol, and gelatin derived from various animal, human, and synthetic sources. Competitive marketing has fueled the debate, questioning the longevity, allergic, immunogenic, and toxic potential of the various delivery substances. Similarly, different processing methods of these DBMs have complicated the literature with variable clinical responses. The main role of DBM is in nonunions or in delayed unions.
A variety of demineralized bone matrices are on the market, generally accepted to be potentially osteoinductive ( Box 24-2 ). Grafton DBM (Osteotech, Inc, Eatontown, New Jersey) has several formulations, including gel, semirigid, and putty forms to improve delivery and integrity. Purported to have osteoinductive properties based on the studies of Urist , the manufacturers report no disease transmission in 1.5 million procedures since its introduction in 1991. A recent study raises concerns about glycerol carrier toxicity in animals, although this toxicity has not been documented in humans. Allosource (Denver, Colorado) markets its original DBM, Allogro, and a putty variant, Allofuse DBM. Dynagraft II (IsoTis, Toronto, Canada, recently acquired by Integra LifeSciences, Plainsboro, New Jersey) is another DBM product, with its carrier a combination of a polymer, ethylene oxide, and propylene oxide available as a putty or gel. The same company also offers the Accell family of products and claims to use a validated in vitro assay to demonstrate osteoinductivity. Accell 100 is the original moldable form, Accell Connexxus uses a reverse-phase medium that makes the DBM resistant to irrigation, and Accell TBM can be infused with bone marrow aspirate using the company’s AspireX technique. DBX (Musculoskeletal Transplant Foundation and Synthes, Paoli, Pennsylvania) is a demineralized human bone matrix with collagen and xenograft hyaluronic acid available in putty or paste. Osteofil (Medtronic Sofamor Danek, Minneapolis, Minnesota) is a DBM in a gelatin and water medium available in paste as well as moldable strips. Intergro (Interpore Cross International of EBI and now Biomet, Warsaw, Indiana) is a DBM with a nonsoluble lipid carrier that will not wash away and comes in putty, paste, or paste premixed with coralline HA granules. Optefil (Exactech, Alachua, Florida, and processed by Regeneration Technologies, Inc) also consists of DBM in a gelatin carrier and comes in injectable or putty form.
Grafton (Osteotech)
Allogro/Allofuse (Allosource)
Dynagraft II/Accell (IsoTis, now Integra LifeSciences)
DBX (Musculoskeletal Transplant Foundation and Synthes)
Osteofil (Medtronic Sofamor Danek)
Intergro (Interpore Cross International of EBI, now Biomet)
Optefil (Exactech)
PlatFORM DBM (Biomet)
All companies claim more rapid ossification via osteoinduction and are quick to point out the potential dangers of other carrier substances. Biomet’s PlatFORM DBM is 100% human tissue, without a synthetic carrier. It can be hydrated with bone marrow aspirate, which adds osteogenic cells, and it comes in flexible sheets. Patent infringement suits exist among the companies in an environment of intense competition.
Bone and Mineral Composites
Cancellous bone and mineral substitutes combined with DBM provide potential osteoinduction and osteoconduction. Opteform (Exactech) includes both Osteofil DBM and cancellous bone chips, which can be molded to suit the needs of the defect or problem. Orthoblast II (IsoTis, now Integra LifeSciences) is a combination of DBM and cancellous bone available in a putty or paste. Allomatrix (Wright Medical Technology, Inc, Arlington, Tennessee) is a putty of Allosource DBM combined with calcium sulfate (Osteoset, Wright Medical Technology, Inc). Osteoset 2DBM) incorporates demineralized bone matrix encapsulated in calcium sulfate pellets, which theoretically allows for sustained release of bone morphogenic proteins ( BMPs) over the period of resorption of the pellets. Each composite has varying capabilities and indications, although, like most substitutes, its use is largely based on the surgeon’s preference. The formulations include putty (customized); a block, indicated for periarticular fractures with purported stability, and injectable varieties. The combination of DBM with calcium sulfate has been shown to be relatively safe and effective in treating bone defects.
Xenografts
Another variation of the mineral composite uses bovine collagen and a mineral substitute, serving osteoconductive purposes. Healos (DePuy Spine, Raynham, Massachusetts) is a matrix composed of cross-linked bovine collagen fibers coated with HA. Another example is Collagraft (distributed in the United States by Zimmer, Inc, Warsaw, Indiana, originally of NeuColl, Campbell, California), one of the first substitutes approved by the FDA in 1993. It is a composite of bovine collagen matrix, HA, and TCP available in granular and strip forms. Collagraft was approved for treatment of diaphyseal and metaphyseal defects to provide osteoconduction. When used with autologous bone marrow aspirate, it was acknowledged as having both osteoconductive and osteoinductive capabilities, perhaps even osteogenic. It possesses no structural support. A prospective, randomized study compared autograft and Collagraft, reporting 213 patients with 249 long-bone fractures. The material performed as well as autograft by radiographic assessment, with similar complication rates.
MINERAL SUBSTITUTES
Ceramics
The mineral substitutes ( Box 24-3 ) may be arbitrarily divided into ceramics and cements. Ceramic is a mineral salt heated to high temperatures, greater than 1000° C, in the process known as sintering . Sintering provides strength to a finished material, but when applied to bone or similar minerals, it decreases remodeling and the resorption capability of bone.
CALCIUM SULFATE
BonePlast (Interpore Cross International, now Biomet)
Calceon (Synthes USA)
Jax-CS (Smith & Nephew)
Osteoset/MIIG (Wright Medical Technology)
Pro Osteon (Interpore Cross International, now Biomet)
TRICALCIUM PHOSPHATE (TCP)
Cellplex (Wright Medical Technology)
Cerasorb (curasan)
ChronOS (Synthase USA)
Vitoss (Orthovita)
COMBINATIONS: HA/TCP
Conduit (DePuy/Johnson & Johnson)
OsSatura: 20/80 (IsoTis, now Integra LifeSciences)
Calstrux (Stryker)
Mastergraft: 15/85 (Medtronic Sofamor Danek)
Jax-TCP (Smith & Nephew)
Many surgeons express concern about the slow disappearance of the mineral substances on radiographs—whether by resorption or remodeling. This concern is supported by several arguments: the material is a foreign object, it obscures bone healing, and it potentially alters the mechanics of joints and soft tissue, since ceramic substitute is a harder substance than the surrounding bone. Although this concern may be unsubstantiated, radiographic appearance of bone trabeculation replacing the substitute is an increasingly desirable attribute of new materials. More extensive interconnected porosity of a substance permits faster bony ingrowth but weakens the material; the ideal pore size is thought to be between 150 and 500 μ. Both sintered and nonsintered porous substances exist, of which the latter permits faster resorption. Whether resorption correlates with remodeling depends on the material and the local environment.
Endobon (Biomet/Merck/Lorenz Surgical, Zwijndrecht, Netherlands) is a bovine-derived ceramic composed of cancellous bone, sintered and processed to eliminate all but the mineral components. The potential lack of immunogenicity is perhaps counterbalanced by the poor remodeling capability rendered by sintering. The HA is porous and permits bone ingrowth. There is a large European experience for use of this ceramic in orthopedic, maxillofacial, and craniofacial fractures and in bone defects. Its use has been reported specifically in distal radius fractures, and it has FDA approval for maxillofacial indications. Like other HA materials, it has little potential for resorption and comes in blocks, cylinders, and granules.
Coralline HA is coral that is thermochemically treated with ammonium phosphate , which demonstrates porosity similar to that of bone ( Fig. 24-3 A and B). The thermochemical conversion process for Pro Osteon (Interpore Cross International, now Biomet) converts 95% of coral calcium carbonate into more slowly resorbed HA. This is not a sintering process, and it is therefore not technically a ceramic, but the conversion has the similar effect of minimizing its resorption. Bone ingrowth has been demonstrated in the interconnected pores, with osteoblasts evident on the surface of the material. Remodeling has not been identified. Formulations include different pore sizes, with the larger (approximately 500 μ) pore size being more appropriate for cancellous bone defects. Its compressive strength is reported as approximately 4 MPa (megapascals). The 200-μ size is used more commonly in dentistry. Recent formulation with calcium carbonate on its surface (Pro Osteon R) permits some resorption and potentially better ingrowth. Pro Osteon was the first calcium phosphate-based bone graft substitute to receive FDA approval for fracture treatment in 1992. It received general use approval in 1998. The 500-μ pore size formulation has found widespread orthopedic applications and has been shown to be useful in fractures of the distal radius for both internal and external fixation.

Osteoinduction may be introduced with the osteoconduction of coralline HA when grafting is combined with autologous platelets from a special autotransfusion process. The cytokines, transforming growth factor beta (TGF-β) and platelet-derived growth factor (PDGF), have been identified in this filtrate through the technique called autologous growth factors (AGFs, also marketed by Interpore Cross).
Calcium Sulfate
Calcium sulfate, better known as plaster of Paris, results from the calcination of gypsum (CaSO 4 , 2H 2 O), which partiallydehydrates to produce a hemi-hydrate (CaSO 4, ½H 2 O).
( CaSO 4 , 2 H 2 O ) + heat = ( CaSO 4 , ½ H 2 O ) + 1.5 H 2 O
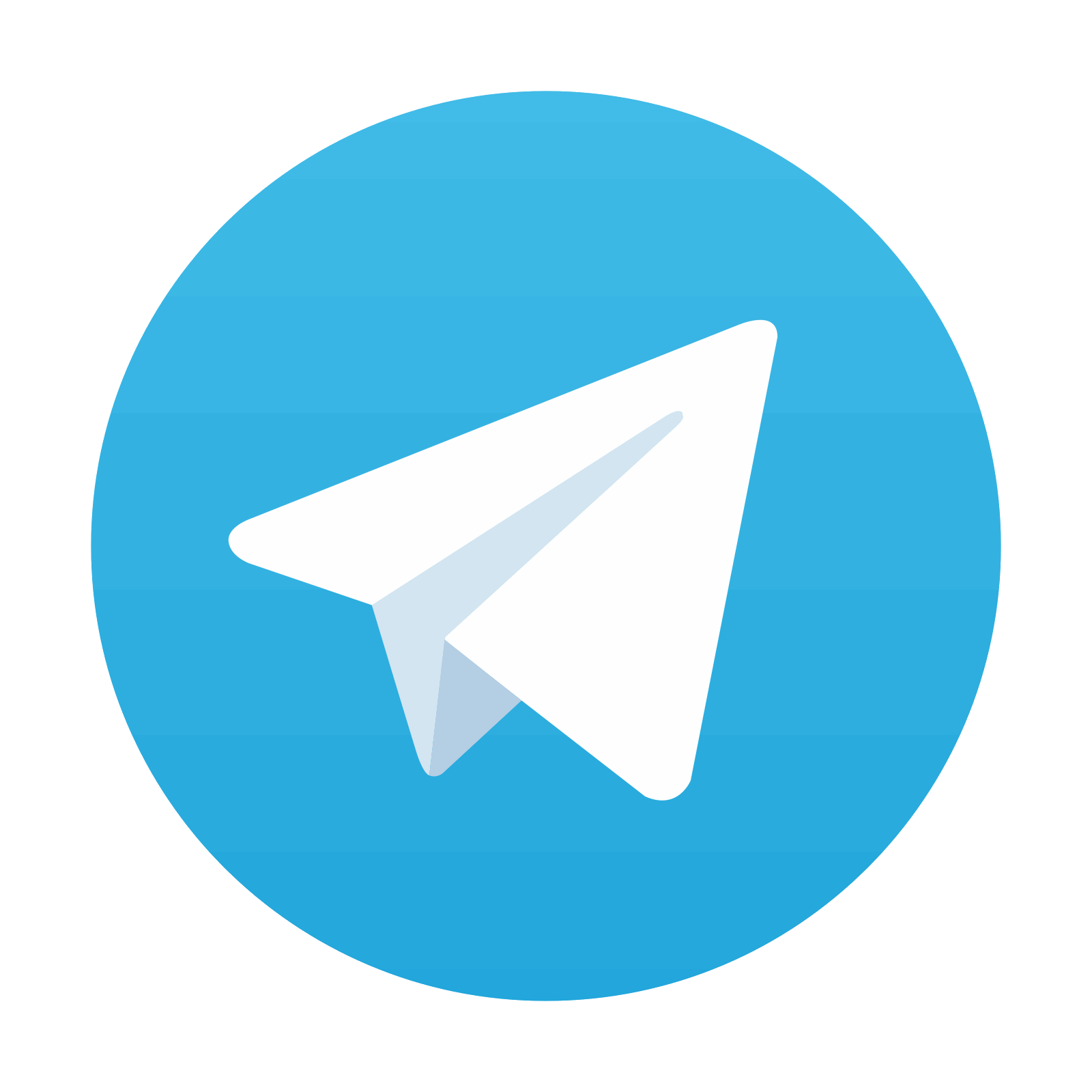
Stay updated, free articles. Join our Telegram channel

Full access? Get Clinical Tree
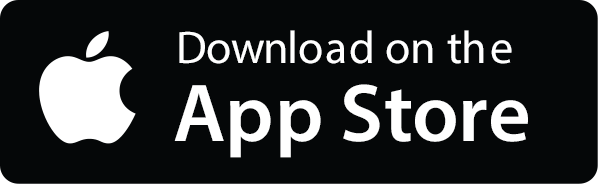
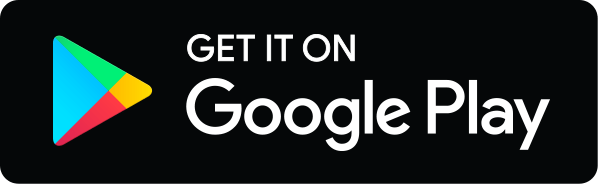